Abstract
Inherited cholestasis of hepatocellular origin has long been described in the neonate or during the first year of life [1]. Many of these infants were categorized as having idiopathic neonatal hepatitis after biliary atresia, metabolic diseases, and congenital infections were excluded [2]. The prognosis in familial cholestasis was poor compared with sporadic cholestasis that sometimes had an identifiable etiology. As the clinical and genotypic heterogeneity of these inherited disorders has become apparent, it is now recognized that patients may present initially and progress to end-stage liver disease at ages ranging from infancy to adulthood [3, 4].There may be significant overlap in clinical features such as intense pruritus and a low serum concentration of gamma-glutamyltransferase (GGT). The histopathology, immunohistochemical staining, and hepatic ultrastructure may provide additional diagnostic clues as to the underlying defect. However, next generation sequencing including use of targeted gene panels has proven of great value in rapidly and reliably discriminating cholestatic diseases of childhood, may suggest therapy with varying success based on the genotype of the patient, and has advanced our understanding of molecular mechanisms of bile secretion and acquired cholestasis [5]. It is not surprising that, so far, mutations in three genes encoding ATP-dependent transport proteins localized to the canalicular membrane that result in progressive cholestasis and liver injury have been discovered. The features of these disorders are compared in Table 13.1. Other genes encoding proteins involved in membrane transport, vesicular trafficking, and integrity of the cell junction may also be mutated in some patients. Mutations in the genes responsible for PFIC may be found in some adults with cryptogenic cholestasis and women with cholestasis of pregnancy including in the heterozygous state [6]. Owing to an immaturity of hepatic excretory function, cholestasis may occasionally occur in inherited diseases because of systemic illness rather than a primary defect in the liver (see Table 9.1). These disorders will not be considered in this review.
Introduction
Inherited cholestasis of hepatocellular origin has long been described in the neonate or during the first year of life [1]. Many of these infants were categorized as having idiopathic neonatal hepatitis after biliary atresia, metabolic diseases, and congenital infections were excluded [2]. The prognosis in familial cholestasis was poor compared with sporadic cholestasis that sometimes had an identifiable etiology. As the clinical and genotypic heterogeneity of these inherited disorders has become apparent, it is now recognized that patients may present initially and progress to end-stage liver disease at ages ranging from infancy to adulthood [3, 4].There may be significant overlap in clinical features such as intense pruritus and a low serum concentration of gamma-glutamyltransferase (GGT). The histopathology, immunohistochemical staining, and hepatic ultrastructure may provide additional diagnostic clues as to the underlying defect. However, next generation sequencing including use of targeted gene panels has proven of great value in rapidly and reliably discriminating cholestatic diseases of childhood, may suggest therapy with varying success based on the genotype of the patient, and has advanced our understanding of molecular mechanisms of bile secretion and acquired cholestasis [5]. It is not surprising that, so far, mutations in three genes encoding ATP-dependent transport proteins localized to the canalicular membrane that result in progressive cholestasis and liver injury have been discovered. The features of these disorders are compared in Table 13.1. Other genes encoding proteins involved in membrane transport, vesicular trafficking, and integrity of the cell junction may also be mutated in some patients. Mutations in the genes responsible for PFIC may be found in some adults with cryptogenic cholestasis and women with cholestasis of pregnancy including in the heterozygous state [6]. Owing to an immaturity of hepatic excretory function, cholestasis may occasionally occur in inherited diseases because of systemic illness rather than a primary defect in the liver (see Table 9.1). These disorders will not be considered in this review.
Table 13.1 Progressive familial intrahepatic cholestasis
Type 1 | Type 2 | Type 3 | |
---|---|---|---|
Transmission | Autosomal recessive | Autosomal recessive | Autosomal recessive |
Chromosome | 18q21-22 | 2q24 | 7q21 |
Gene | ATP8B1/FIC1 | ABCB11/BSEP | ABCB4/MDR3 |
Protein | Familial intrahepatic cholestasis 1 (FIC1) | Bile salt export pump (BSEP) | Multidrug resistance-associated protein 3 (MDR3) |
Location | Wide tissue distribution including almost all epithelial cells: on apical membranes | Hepatocyte canalicular membrane | Hepatocyte canalicular membrane |
Function | ATP-dependent aminophospholipid flippase; may influence farnesoid X receptor-mediated signaling; may alter function and stability of membrane proteins | ATP-dependent bile acid transport | ATP-dependent PC translocation |
Phenotype | Progressive cholestasis with severe pruritus, diarrhea, steatorrhea, growth failure, sensorineural hearing loss, pancreatitis, respiratory disease | Rapidly progressive cholestatic giant cell hepatitis, growth failure, pruritus, hepatocellular carcinoma | Later-onset cholestasis, portal hypertension, minimal pruritus, intraductal and gallbladder lithiasis |
Histology | Initial bland cholestasis; coarse, granular canalicular bile on EM | Neonatal giant cell hepatitis, amorphous canalicular bile on EM | Bile ductular proliferation, periportal fibrosis, eventually biliary cirrhosis |
Biochemical features | Normal serum GGT; minimal to modest serum aminotransferase elevation, high serum bile acid levels, low biliary bile acid secretion | Normal serum GGT; high serum, low biliary bile acid concentrations | Elevated serum GGT; low to absent biliary PC; absent serum lipoprotein X; normal biliary bile acid concentrations |
Treatment | Biliary diversion, ileal exclusion, liver transplantation – but post-orthotopic liver transplantation diarrhea, pancreatitis, steatorrhea, fatty liver with possible progression to cirrhosis | Biliary diversion, liver transplantation (possible recurrent disease after transplantation) | Ursodeoxycholic acid if residual PC secretion; liver transplantation |
EM, electron microscopy; PC, phosphatidylcholine
Progressive Familial Intrahepatic Cholestasis Type 1 (ATP8B1 Deficiency)
Byler disease was initially described in direct descendants of Jacob Byler. Byler disease and benign recurrent intrahepatic cholestasis (BRIC), diseases that are now known to be caused by defects in the gene ATP8B1 (once known as FIC1), were some of the first clinically well-described forms of familial intrahepatic cholestasis [7]. A large part of the published literature regarding these disorders predates the molecular genetics of these diseases, so completely accurate genotype–phenotype correlation is not possible. BRIC was first described as an intermittent form of intrahepatic cholestasis characterized by variable periods of intense pruritus often associated with jaundice.
Classically, FIC1 disease was considered to be two different disorders: Byler disease or progressive familial intrahepatic cholestasis type 1 (PFIC1) and BRIC [3]. However, we now view these two disorders as two ends of a continuum, so this historical nomenclature of Byler disease and BRIC may be outdated. Many clinicians now refer to all these diseases in a general sense as FIC1 disease. The natural history of the disease is rarely observed in the current era as clinical interventions alter the course of disease.
Clinical Features
Progressive familial intrahepatic cholestasis type 1 is an autosomal recessive liver disease characterized by unremitting cholestasis with pruritus and jaundice that usually starts before the age of one year and progresses to cirrhosis and liver failure [8, 9]. Serum GGT activity is normal and aminotransferase levels are only minimally elevated. The latter may distinguish the presentation of FIC1 from bile salt export pump (BSEP) disease (PFIC type 2) [10]. The average age at onset is two months, but some patients are affected as neonates and rarely cholestasis may not be manifest until adolescence. Diarrhea, malabsorption, and failure to thrive are common in the first months of life. Fat-soluble vitamin malabsorption can lead to a potentially fatal bleeding diathesis from vitamin K deficiency, rickets from vitamin D deficiency, and neuromuscular dysfunction from vitamin E deficiency. The disorder typically does not progress to end-stage liver disease during early childhood but gradually evolves to cirrhosis in the second decade of life [3]. As such, hepatosplenomegaly eventually develops as a manifestation of progressive liver disease. Few patients with early onset of cholestasis have survived into the third decade without treatment. Several patients have also been described with recurrent attacks of cholestasis beginning in infancy and that eventually became permanent as adults.
Pruritus is the dominant feature of cholestasis in the majority of patients and is often out of proportion to the level of jaundice [10, 11]. It may initially vary in intensity and may be exacerbated during intercurrent illness. Pruritus may not be noticed until six months of age because the neural pathways necessary for concerted scratching are not fully developed. However, affected infants often are irritable and fretful and sleep poorly with onset of cholestasis. Scratching is usually evident first as digging at the ears and eyes, which are the first areas to show evidence of excoriation. By one year of age, patients may show generalized mutilation of skin, usually most severe on the extensor surfaces of the arms and legs and on the flanks of the back. The pruritus is very disabling and often responds poorly to pharmacologic therapies. In contrast to other cholestatic disorders, these patients do not develop xanthomas.
Growth failure is another major feature of PFIC1 [10, 11]. Most patients have short stature (less than the fifth percentile), although their weight for height is often normal, giving a stocky appearance. Delayed onset of puberty and sexual development is characteristic of patients surviving until adolescence without treatment. Patients receiving effective treatment experience normal sexual development and several have borne normal children. Intellectual development and school performance are generally normal in patients receiving effective treatment but may be delayed before treatment, probably as a result of constant pruritus and associated problems with sleep and inattention.
There is an association of FIC1 disease with a variety of extrahepatic manifestations, which we now understand may be the result of the wide tissue distribution of ATP8B1 expression [12]. The more commonly described extrahepatic manifestations include recurrent pancreatitis, diarrhea that is independent of cholestasis, sensorineural hearing loss, chronic cough/ wheezing, and somatic short stature. Many of these problems persist and may even worsen after liver transplantation, supporting the notion that they are the result of ATP8B1 expression in organs other than the liver [10]. Severe diarrhea and progressive steatohepatitis are particularly problematic after liver transplantation. Steatosis is only seen after liver transplantation and can be progressive, leading to cirrhosis in less than ten years [13].
A form of benign recurrent intrahepatic cholestasis (BRIC1 – BRIC associated with defects in FIC1) is characterized by attacks of jaundice and pruritus separated by symptom-free intervals [10, 11]. Patients may also experience fatigue, anorexia, steatorrhea, dark-colored urine, and weight loss. Progression to cirrhosis and long-term complications of chronic liver disease are less commonly observed, but may occur later in the course compared with individuals with more severe FIC1 disease. The disorder is also caused by mutations in ATP8B1 and is inherited as an autosomal recessive trait.
The age of presentation of the first attack of jaundice ranges from 1 to 50 years, but jaundice usually occurs before the age of 20 years [3]. Attacks usually are preceded by a minor illness and consist of a preicteric phase of two to four weeks (characterized by malaise, anorexia, and pruritus) and an icteric phase that may last from 1 to 18 months. In some patients, hormonal factors such as the use of oral contraceptives or pregnancy have been associated with precipitation of an attack. Patients may have severe coughing during episodes, as is seen sometimes in patients with PFIC1.
During the icteric phase, the concentrations of serum bile acid, bilirubin, and alkaline phosphatase are increased. Serum GGT concentration, however, remains low. Liver biopsy results are very benign, often showing no pathologic change even during an episode. Some specimens show hepatocellular cholestasis and cholate injury, mostly centrilobular. During the asymptomatic period, all parameters are normal: clinical, laboratory, and liver histology.
Laboratory Findings
The laboratory findings in PFIC1 and BRIC1 are remarkable for the presence of low serum GGT and normal or near normal serum cholesterol levels, even in the presence of severe cholestasis [11]. Patients may have serum GGT >100 IU/L while receiving microsomal inducers such as phenobarbital. Serum concentrations of alkaline phosphatase, bilirubin, and bile salts do not differ from those seen in several other cholestatic disorders and may be normal or near normal early in the course of the disease. Serum aminotransferase levels are usually no higher than twice normal values. Patients with PFIC1 or a prolonged episode of BRIC1 frequently develop complications of cholestasis, including fat-soluble vitamin deficiency. Sweat chloride and sodium levels may be elevated. With progressive liver injury, features of hepatic failure and portal hypertension develop that are similar to those of any other end-stage liver disease.
A low serum GGT level is highly unusual in the presence of cholestasis. It should suggest the possibility of one of the forms of PFIC or BRIC [11]. However, the differential diagnosis should include inborn errors of bile acid synthesis. In PFIC and BRIC, serum concentrations of primary bile acids are markedly elevated, whereas in bile synthetic defects, the serum contains abnormal bile acid precursors but no primary bile acids. Pruritus is an uncommon manifestation in bile acid synthetic defects. The mechanism for the low serum concentration of GGT in PFIC and BRIC is not clear. This enzyme is normally bound to the canalicular membrane by a glycosyl phosphatidylinositol (GPI) anchor. In obstructive cholestasis, when excessive amounts of bile salts accumulate in the canalicular lumen under increased pressure, GGT is released from the membrane by detergent action and refluxes back into serum, possibly via leaky intercellular junctions. However, in PFIC and BRIC, alterations in lipid bilayer characteristics may lead to release of canalicular enzymes into bile. Immunohistochemical studies indicate that some canalicular proteins, including GGT and carcinoembryonic antigen, are poorly expressed at the canaliculus in PFIC1 [3].
Consistent with severe cholestasis, total serum bile acid concentrations are markedly elevated (usually >200 μmol/L; normal, <10 μmol/L) with an elevated ratio of chenodeoxycholic acid to cholic acid conjugates, usually >10:1. The total biliary bile acid concentrations are generally low (0.1–0.3 mmol/L; normal, >20 mmol/L), with a predominance of cholic acid conjugates. These findings have suggested a defect in biliary excretion, particularly of chenodeoxycholic acid conjugates.
Histopathology
A bland hepatocellular and canalicular cholestasis with some pseudo-acinar transformation are the most uniform histologic findings early in the course of the disease (Figure 13.1) [14–16]. Minimal giant cell formation and ballooning of hepatocytes also may be found. Giant cells are present more often during infancy and may regress with age. Bile duct damage is minimal in infants but may be more prominent later, leading to ductal paucity. The degenerating biliary epithelium shows apoptotic changes consisting of small hyperchromatic nuclei, attenuated cytoplasm, and loss of duct lumina, but inflammation is absent. The typical progression of fibrosis starts early, with 76% of patients having some fibrosis by two years of age, and fibrosis may appear initially either as pericentral sclerosis or portal fibrosis, or sometimes both. Portal to central bridging then develops in association with lacy lobular fibrosis and eventually leads to cirrhosis. Proliferating bile ductules are observed at the edge of the portal tracts in patients with significant fibrosis. The rate of progression of the fibrosis is highly variable but correlates loosely with the severity of the clinical disease. Mallory hyaline and hepatocellular carcinoma may be seen with very advanced disease. Unfortunately, well-characterized and clinically useful antibodies against the FIC1 protein do not exist, so routine direct histochemical analysis cannot be performed to diagnose FIC1 disease. Indirect analysis of GGT staining may suggest a diagnosis of FIC1 disease [16].
Figure 13.1 Histopathology in progressive familial intrahepatic cholestasis type 1. Liver biopsy from a 2-year-old patient showing swelling of hepatocytes, a bland hepatocellular, and canalicular cholestasis, and occasional necrotic hepatocytes
Electron microscopy on liver samples from patients who are not receiving ursodeoxycholic acid (UDCA) shows distended bile canaliculi with microvilli that are reduced in number and length (Figure 13.2). Bile canaliculi contain unusually coarse and granular bile (so-called Byler bile). In some confirmed cases of PFIC1, aggregated vesicles of membranous material rather than coarse granular bile are observed. Bile is retained in hepatocytes and Kupffer cells and is predominantly periportal in localization. The pericanalicular ectoplasm is often thickened. These ultrastructural abnormalities are highly suggestive of, but not absolutely specific for PFIC1 [15].
Figure 13.2 Ultrastructural pathology of progressive familial intrahepatic cholestasis type 1. Electron microscopy of liver from a patient shows distended bile canaliculi with microvilli that are reduced in number and length. Bile canaliculi contain unusually coarse and granular bile (so-called Byler bile).
Genetics
Gene linkage analysis of BRIC and Byler disease indicated that the same gene is involved in both of these diseases, and is mapped to chromosome 18q21. Refined linkage analysis and gene sequencing of patients with well-characterized disease ultimately led to the discovery that defects in the P-type ATPase (FIC1 or ATP8B1) are responsible for FIC1 disease [7]. ATP8B1 contains 28 exons, spans at least 77 kb, and yields no alternatively spliced transcripts [17]. The FIC1 protein consists of 1251 amino acid residues and has the ten predicted membrane-spanning domains typical of P-type ATPases. Mutational analyses in 180 families with PFIC1 and 50 families with BRIC1 identified 54 distinct disease mutations, including ten mutations predicted to disrupt splicing, six nonsense mutations, 11 small insertion or deletion mutations predicted to induce frameshifts, one large genomic deletion, two small in-frame deletions, and 24 missense mutations [16, 17]. Mutations of ATP8B1 were detected in 30% and 41%, respectively, of the PFIC and BRIC families screened. Most mutations were rare, occurring in one to three families, or were limited to specific populations. Compound heterozygotes were commonly observed (nine in 39 with PFIC1 and 12 in 20 with BRIC1). Based on sequence analysis and the predicted effects of specific mutations, it is hypothesized that BRIC-like disease is the result of functionally less severe mutations in ATP8B1. Missense mutations were more common in BRIC1 (58% vs. 38% in PFIC1), whereas nonsense, frameshifting, and large deletion mutations were more common in PFIC1 (41% vs. 16% in BRIC1) [17]. In one study, 14 of 16 patients with BRIC1 were either homozygous or compound heterozygous for the I661T mutation, which was frequently found in European patients [17]. Non-sense mutations at the 5′ end of the ATP8B1 coding region are typically associated with severe disease, whereas mutations at the 3′ end are associated with milder and intermittent disease. A functional assay examining signaling by the nuclear receptor farnesoid X receptor (FXR) suggests that disease severity correlates with FIC1 function [18]. Those with BRIC type mutant FIC1 may have reduced plasma membrane targeting that can be partially corrected by chemical chaperones [19].
Pathophysiology
Occurrence of FIC1 appears to be the result of abnormalities in the enterohepatic circulation of bile acids [3]. In vivo clearance of radiolabeled bile acids in patients with Byler disease and careful examination of biliary bile acids suggest that there is reduced hepatic canalicular excretion of bile salts. Clinical response to partial biliary diversion or ileal exclusion indicates that enhanced intestinal reabsorption of bile acids may also be involved in the disease process. Animal models of Byler disease have not yielded a simple explanation of the molecular events involved in FIC1 [20]. The G308V mutation initially described in the Byler kindred was introduced into mice. Adult Atp8b1G308V/G308V mice expressed mRNA for FIC1 but not protein. The mice were normal appearing, although serum aspartate aminotransferase and bile salts were elevated compared with wild-type littermate controls. Paradoxically, bile flow and hepatic excretion of bile salts were enhanced in the Byler mice. Cholate feeding of the Atp8b1G308V/G308V mice exacerbated the pathology and suggested that abnormal regulation of bile acid homeostasis is operative in the pathogenesis of FIC1 [21].
The Byler mouse has been a clearer model of some aspects of extrahepatic disease. Hearing deficits, as assessed by auditory brainstem responses, develop in Byler mice as they age [22]. The protein FIC1 is produced in the hair cells of the organ of Corti and the organ of Corti degenerates as the Byler mice age. Bacteria-induced lung injury is worsened in Byler mice and correlates with the cardiolipin clearance from lung fluid [23].
At present, the exact molecular pathophysiology of FIC1 is uncertain. This is in contrast to defects in BSEP and MDR3, in which the presumed pathophysiology follows directly from protein function. Based on sequence and homology analysis, it has been presumed that FIC1 is a P-type ATPase that functions as an aminophospholipid flippase, transferring aminophospholipids from the outer to inner hemi-leaflet of cell membranes with a preference for phosphatidylcholine [24]. Aminophospholipid translocase activity has been demonstrated in rat liver canalicular membrane vesicles that contained FIC1. Transfection of a mutagenized Chinese hamster ovary cell line that lacks FIC1 cells with ATP8B1 cDNA resulted in the production of FIC1 in membrane preparations and energy-dependent translocation of a fluorescent analogue of phosphatidylserine. Studies in mice have also shown that transmembrane protein 30 A (TMEM30A) and CDC50A are essential for the expression and membrane localization of ATP8B1 [25, 26]. These data suggest that FIC1 is an aminophospholipid transporter that helps to maintain the appropriate asymmetric distribution of aminophospholipids between the inner and outer leaflets of the plasma membrane [27]. Lipid bilayer composition may influence the activity of integral membrane proteins and may also increase the susceptibility of the membrane to the detergent effects of bile. Cholesterol depletion is found in FIC1-deficient canalicular membranes and this depletion is associated with diminished BSEP function [28]. Byler mice have diminished resistance of the bile canalicular membrane to hydrophobic bile acids. As such, canalicular ectoenzymes are sloughed into bile and there is a marked reduction in the cholesterol content of the bile canalicular membrane, which would be expected to diminish BSEP activity and lead to cholestasis. The relevance of lipid asymmetry at the canalicular membrane is highlighted by the counteracting effects of aminophospholipid flipping and phosphatidylcholine flopping demonstrated in the Atp8b1 and Abcb4 double knockout mice [29]. In this model, the deleterious effects of Abcb4 loss of function are partially rescued by the superimposition of Atp8b1 dysfunction.
Alterations in FIC1 may alter apical membrane protein production independent of flippase activity [27]. Asymmetry of aminophospholipids in lipid bilayers may also play a role in regulating important lipid-dependent signaling pathways. The protein FIC1 is produced at the canalicular membrane of the hepatocyte and at the apical membrane of bile duct epithelial cells and enterocytes [30]. It is abundant along the entire length of the gastrointestinal tract from the stomach to the colon and is also found in the pancreas. There does not appear to be a direct linkage between FIC1 and bile acid transport proteins.
An alternative hypothesis for the pathogenesis of Byler disease is based upon the supposition that ATP8B1 expression may influence post-translational modification of FXR, leading to enhancement of its nuclear localization and transcriptional activity [31]. The FXR is a nuclear protein that is integral to the bile acid responsiveness of a variety of genes involved in bile acid biosynthesis and transport. It positively regulates the expression of BSEP and negatively regulates the expression of the ileal apical sodium-dependent bile acid transporter. In FIC1 deficiency, it is proposed that there is diminished FXR activity, leading to reduced production of the canalicular BSEP and increased production of the ileal apical sodium-dependent bile acid transporter. The net effect of these changes would be enhanced reabsorption of intestinal bile acids coupled with diminished hepatic excretion of bile acids, yielding marked hypercholanemia and diminished hepatic bile acid secretion (Figure 13.3). Analysis of ileum from individuals with FIC1 disease reveals evidence of diminished FXR signaling and increased expression of the ileal apical sodium-dependent bile acid transporter [31]. These findings have not been observed in the Byler mice [10]. Assessment of FXR signaling and BSEP expression in human liver from individuals with Byler disease has yielded conflicting results and is limited by small numbers of samples and inadequate controls. Silencing FIC1 in primary human hepatocytes is associated with diminished FXR signaling and reduced endogenous BSEP production [32].
Figure 13.3 Hypothetical model of the effect of familial intrahepatic cholestasis 1 (FIC1). This is a membrane protein that alters membrane aminophospholipid asymmetry and transduces an unknown signaling pathway (curved arrows). This leads to a post-translational modification of the farnesoid X receptor (FXR), possibly through some type of phospholipid-dependent signaling pathway (indicated by the attached star). The post-translational modification is necessary for nuclear translocation of FXR. FXR in the nucleus then activates itself (FXR), the ileal lipid-binding protein (ILBP), the inhibitory transcription factor, the short heterodimer partner (SHP), and the bile salt export pump (BSEP). FXR, via the effect of SHP, inhibits the expression of the apical sodium-dependent bile acid transporter (ASBT) and cholesterol 7α-hydroxylase (CYP7A). PE, phosphatidylethanolamine; PS, phosphatidylserine.
Progressive Familial Intrahepatic Cholestasis Type 2 (ABCB11 Deficiency)
Following the identification of the gene underlying PFIC1 and better delineation of its clinical features, it became clear that there was genetic and clinical heterogeneity in patients with PFIC and low serum GGT. A second locus for PFIC was then mapped to 2q24 by homozygosity mapping and linkage analysis in six consanguineous families of Middle Eastern origin [33]. Mutations were defined later in a liver-specific gene of unknown function, initially called the sister of P-glycoprotein, which was found within this region. Further studies revealed that this gene’s protein, a member of the ATP-binding cassette family of transporters, was located exclusively on the canalicular membrane of hepatocytes and functioned as an ATP-dependent bile acid export pump. Numerous mutations in ABCB11 (was BSEP) have been defined in patients with the form of PFIC linked to 2q24 (now named PFIC2) [33, 34]. The phenotype of these patients is consistent with defective bile salt excretion at the hepatocyte canalicular membrane. Mutations of ABCB11 have also been detected in some patients with BRIC. Variants of ABCB11 have also been associated with drug-induced cholestasis and some cases of intrahepatic cholestasis of pregnancy [29]. The protein BSEP consists of 1,321 amino acid residues (molecular mass ~160 kDa) and, in keeping with other members of the ABC superfamily, has a predicted topology of 12 membrane-spanning domains [29]. It has a tandemly duplicated structure with each half of the molecule composed of six predicted transmembrane domains and a large cytoplasmic nucleotide-binding domain. The protein transports monovalent bile acids with high affinity. Expression of ABCB11 is regulated by FXR, which is activated by bile acids [35].
Clinical Features
PFIC2 is the most common form of PFIC, and usually presents in the neonatal period with progressive cholestasis [3]. In general, these patients lack the relapsing course seen in the early stages of PFIC1 and instead have a more rapid progression to cirrhosis without therapy [8]. Irritability and bleeding related to vitamin K deficiency are commonly seen. Rickets is another complication caused by vitamin D deficiency. Patients may clinically manifest vitamin deficiencies even in the absence of jaundice. Failure to thrive occurs, related to fat malabsorption and poor intake. The majority of patients have hepatomegaly; significant splenomegaly implies portal hypertension related to advanced fibrosis or cirrhosis [8, 34]. Cholelithiasis has been observed in at least 30% of patients, owing to impaired bile acid secretory function and supersaturation of bile with cholesterol. Similar to patients with PFIC1, these patients do not have xanthomas. Extrahepatic features may help to distinguish PFIC1 and PFIC2; watery diarrhea, pancreatitis, and impaired hearing occur in PFIC1 but not in PFIC2 [8].
Pruritus is the dominant feature of the disorder in the majority of patients before complications of cirrhosis develop [3, 8]. Pruritus is often out of proportion to the level of jaundice and is not clinically evident in the first months of life.
Patients with PFIC2 are at risk of developing hepatocellular carcinoma and cholangiocarcinoma [3]. Malignancy may occur as early as ten months of age and in patients with normalized liver tests following biliary diversion [36].
Patients fitting the phenotype of BRIC have been described with mutations in ABCB11 [36]. These patients had at least two recurrent episodes of cholestasis and were clinically healthy and biochemically normal between attacks. The age of onset and total number of recurrent episodes were highly variable. Cholelithiasis occurred in seven of 11 patients with BRIC2. Several patients had a relatively early onset of the disease and developed permanent cholestasis and advanced liver disease as adults after initial periods of recurrent attacks. A patient with transient neonatal cholestasis has also been reported with a small heterozygous deletion in the long arm of chromosome 2.
Laboratory Findings
Patients with PFIC2 have low serum GGT and normal or near normal serum cholesterol levels [3, 34]. The serum GGT concentration may increase to >100 IU/L in patients receiving microsomal inducers such as phenobarbital and rifampicin. Serum concentrations of alkaline phosphatase, bilirubin, and bile salts do not differ from those seen in many other cholestatic disorders. In contrast to patients with PFIC1, serum aminotransferase levels are usually elevated to at least five times normal values. Patients frequently develop complications of cholestasis, including fat-soluble vitamin malabsorption and steatorrhea [36].
Histopathology
Liver morphology in PFIC2 shows a neonatal hepatitis with giant cell transformation of hepatocytes and lobular cholestasis that may persist beyond infancy [3, 15]. Canalicular cholestasis is prominent, particularly in zone 3. Balloon cholestasis of hepatocytes and isolated hepatocyte necrosis may be found. Injury to hepatocytes results in perivenular, pericellular, and periportal fibrosis with progression to cirrhosis. There is mild ductular proliferation and scattered polymorphonuclear leukocytes in portal tracts. The interlobular bile ducts are normal. Immunohistochemistry demonstrates that BSEP antibody staining is abnormal or absent in >90% of cases [34, 37, 38].
Electron microscopy (Figure 13.4) demonstrates effaced microvilli and dilated bile canaliculi that contain finely granular or filamentous bile.
Figure 13.4 Ultrastructural pathology in progressive familial intrahepatic cholestasis type 2. Amorphous bile is observed in the canaliculus of this 2-year-old patient with absent hepatic immunostaining for bile salt export pump and low gamma-glutamyltransferase cholestasis. Bile canaliculi are distended, and microvilli are reduced in number and length (magnification 8000×, bar = 1.25 μm).
Genetics
Progressive familial intrahepatic cholestasis type 2 is inherited as an autosomal recessive trait. ABCB11 is located on chromosome 2q24 and consists of 28 exons. Approximately 200 mutations in ABCB11 have been reported in the Human Gene Mutation Database (www.hgmd.org), including missense, nonsense, deletions and insertions, and slice site mutations [34]. These variants lead to inherited cholestasis of varying severity and predispose to acquired disorders, such as drug-induced cholestasis and intrahepatic cholestasis of pregnancy. The most common defects are missense mutations, and these were present in at least one allele in 79% of 109 families. There is some correlation of mutations with ethnicity with mutations found on at least one allele leading to E297G and D482G in 58% of European families. In PFIC2, about half the mutations resulted in an early stop codon or a frameshift in the encoded protein [34]. The other PFIC2 mutations and all BRIC2 mutations were non-synonymous. Heterogeneity in clinical phenotype from a particular single gene mutation suggests contributions from additional genetic and/or environmental modifiers to the severity of the disease [3].
In a 2001 report describing the genetic analysis of 194 patients with low-GGT PFIC, 103 mutant ABCB11 alleles were found in 63 families [39]. In this group, there were five different nonsense mutations, 19 different missense mutations, eight different 1 or 2 base pair insertions or deletions, two major gene rearrangements, and one complete gene deletion. In 22 of these families, the affected patients were homozygotes; in 41 families, the affected individuals were compound heterozygotes. In a more recent study of 109 families, 82 different mutations (52 novel) were identified (nine nonsense mutations, ten small insertions and deletions, 15 splice-site changes, three whole-gene deletions, 45 missense changes) [34]. Two protein-truncating mutations conferred particular risk of hepatocellular carcinoma, with eight out of 21 patients (38%) carrying them developing malignancy vs. 11 out of 107 patients (10%) with potentially less severe genotypes. In BRIC2, “milder” missense mutations are found more commonly than those leading to an absence of protein on the canalicular membrane and these mutations may occur in less conserved regions of the gene than in the critical Walker A/B motifs.
The more severe mutations result in a marked reduction or complete loss of BSEP expression on the canalicular membrane [34, 37]. Of a group of patients with ABCB11 mutations, ten out of 11 showed no BSEP on the canalicular membrane by immunohistochemical staining. These patients had a variety of abnormalities in ABCB11, including missense, nonsense, and deletional mutations. No ABCB11 mutations were found in any of the eight patients with positive canalicular BSEP staining. This suggests that in the majority of patients with PFIC2, the gene defect is severe enough to produce no product or a protein that cannot be inserted into the canalicular membrane. Immunolocalization can provide a means of diagnosing PFIC2 in the clinical setting [40]. However, detectable BSEP does not exclude the possibility of a functional BSEP defect.
Many of known ABCB11 missense mutations and single-nucleotide polymorphisms have been analyzed and frequently result in impaired BSEP processing in the endoplasmic reticulum or aberrant pre-mRNA splicing. Primary defects at either the protein or the mRNA level (or both) contribute significantly to BSEP deficiency. Specific therapies may be feasible using agents to correct abnormal protein processing with BSEP cell surface expression or to modulate splicing defects [41].
Pathophysiology
The clinical implications of defective canalicular BSEP are quite clear; there will be markedly diminished bile salt secretion and progressive cholestasis. In patients studied by Jansen et al. [37], biliary bile salt concentrations were 0.2 ± 0.2 mmol/L (<1% of normal) in patients with PFIC2 vs. 18.1 ± 9.9 mmol/L (~40% of normal) in patients with other forms of PFIC. Both biliary cholesterol and phospholipid secretion were also markedly reduced. A bile salt kinetic study in one BSEP-deficient patient showed a dramatic decrease in bile salt secretion, with most of the bile salt pool confined to a “central compartment” consisting of liver, blood, and the extracellular space. Owing to bile secretory failure, bile salts and other biliary constituents are retained in the hepatocyte and lead to progressive liver damage. Seven patients studied by Jansen et al. [37] were treated with UDCA but were able to excrete very low amounts of UDCA into bile. These findings indicate that in addition to secretion of the primary bile salts, cholic acid, and chenodeoxycholic acid, BSEP is largely responsible for canalicular transport of UDCA. Patients are more likely to respond to UCDA therapy if there is some residual BSEP function.
Targeted inactivation of Bsep (homologue of Abcb11) in mice has yielded some surprising results [42]. The Bsep–/– mice were growth retarded but exhibited no signs of overt cholestasis or abnormalities in serum liver biochemical tests. As expected, the secretion of cholic acid in mutant mice was greatly reduced (6% of wild type), but total bile salt output in mutant mice was about 30% of wild type. Production of the Mdr1 P-glycoprotein was enhanced in these mice and provided an alternative but incomplete mechanism for bile salt secretion. Secretion of a large amount of tetrahydroxylated bile acids occurred in mutant but not wild-type mice. These results suggest that hydroxylation and an alternative canalicular transport mechanism for bile acids compensate for the absence of Bsep function and protected the mutant mice from severe cholestatic damage. However, feeding the mutant mice with a more hydrophobic bile salt, cholic acid, led to severe cholestasis characterized by jaundice, weight loss, elevated plasma bile acid concentrations, elevated serum aminotransferases, cholangiopathy (with proliferation of bile ductules and cholangitis), liver necrosis, and high mortality [42].
A number of missense mutations in ABCB11 that have been associated with PFIC2 have been studies in vitro [34]. Five mutants, G238V, E297G, G982R, R1153C, and R1268Q, prevented the protein from trafficking to the apical membrane, and E297G, G982R, R1153C, and R1268Q also abolished taurocholate transport activity, possibly by causing BSEP to misfold. Mutant C336S may not be disease causing as it had no effect on transport activity or apical trafficking of BSEP; D482G did not affect the apical expression but partially decreased the transport activity of BSEP. Mutant G238V was rapidly degraded in both MDCK and Sf9 cells and a proteasome inhibitor resulted in intracellular accumulation of this and other mutants, suggesting proteasome-mediated degradation is involved in processing of mutant BSEP. These studies provide useful information on amino acid residues that are critical for BSEP function.
The consequences of mutant E297G and D482G have also been studied [43]. Both arise from missense mutations affecting the second intracellular loop and the first ATP-binding domain, respectively. Introduction of these residue changes into the human BSEP resulted in a significantly reduced BSEP production in kidney cell lines. Most of the D482G and some of the E297G BSEP was retained intracellularly, probably in the endoplasmic reticulum in an immature, core-glycosylated form. However, the transport function of the BSEP mutants assessed in membrane vesicles isolated from transfected HEK293 cells was normal. These studies indicate that impaired membrane trafficking is the abnormality produced by the E297G and D482G mutants. As a strategy to treat certain forms of PFIC2, it may be feasible to develop agents that can induce trafficking of BSEP mutants that retain transport activity to the canalicular membrane. A BSEP deficiency model has been generated using patient-specific induced pluripotent stem cell-derived hepatocyte-like cells, and may prove useful for pathophysiological analysis of various mutations and drug development [44].
Treatment of Progressive Familial and Benign Recurrent Intrahepatic Cholestasis
The treatment of PFIC includes standard measures related to the management of chronic cholestasis and specific approaches to these forms of intrahepatic cholestasis. Fat-soluble vitamin supplementation and monitoring are necessary for all forms of chronic cholestasis. Coagulopathy in the newborn period has resulted in intracranial hemorrhage and death in children with PFIC, particularly when vitamin K is not administered in the newborn period. Nutritional supplementation with medium-chain triglycerides may be necessary for adequate caloric assimilation. The most difficult therapeutic issue in PFIC relates to management of pruritus. Conventional therapies with antihistamines and UDCA are of limited efficacy in this patient population. Opioid antagonists are problematic to administer and not terribly efficacious. Variable and typically temporary response may be observed with rifampicin. Therefore, most common medical approaches to the intractable pruritus associated with cholestasis are often ineffective in PFIC1 and PFIC2. Interruption of the enterohepatic circulation can yield excellent clinical, biochemical, and histological responses in a number of children with progressive intrahepatic cholestasis. The exact mechanism(s) by which this intervention works is unclear, although it is likely that there is a significant change in the composition of the bile acid pool. At present, it is unclear if these approaches are optimal for specific genetic forms of PFIC. It is possible that these interventions may be best for severe FIC1 (PFIC1) and milder phenotypic variants of PFIC2. There are two major surgical techniques to permanently interrupt the enterohepatic circulation, namely cutaneous external biliary diversion (PEBD) and internal partial ileal exclusion. In the first procedure, one end of a jejunal conduit is anastamosed to the dome of the gallbladder and the other is used to form a cutaneous ostomy. Bile in the gallbladder then flows either out of the ostomy or into the intestine. Typically 30–70% of bile drains out of the ostomy and is discarded. This procedure, first described by Whitington and Whitington [45], yields excellent clinical responses in a significant percentage of patients with low-GGT intrahepatic cholestasis (presumed FIC1 disease in many cases). The response often includes complete amelioration of pruritus and importantly biochemical and histological stabilization and possible improvement. The response may be transiently diminished in females near the time of puberty. In addition, some children develop episodes that resemble BRIC after successful biliary diversion. Modifications of this procedure including internal diversion to the colon and use of a “button” have been described as means to obviate the need for a chronic ostomy bag.
An alternative, although less commonly used, surgical approach to interrupting the enterohepatic circulation of bile acid involves internal partial ileal exclusion [36]. The vast majority of intestinal bile salts are reabsorbed in the distal ileum, that is, the distal 20–25% of the small intestine. Therefore, exclusion of this segment of intestine may lead to bile acid wasting, as has been extensively demonstrated in the surgical treatment of hypercholesterolemia. The small intestine is transected at a point that demarcates the distal 15% of the small intestine, and a blind loop is formed with the distal ileal segment. The proximal loop of the intestine is sewn end to side to the cecum, completing the internal bypass of the distal ileum. There is limited experience with this surgical approach in PFIC, although some success has been reported. Avoidance of an ostomy is attractive for many patients and their families. Accurate assessment of the appropriate amount of ileum for bypass is likely to be critical; too little is unlikely to be therapeutic and too much is likely to yield bile acid-induced diarrhea. At present, it is not clear if there will be compensatory responses in the intestine that will ultimately diminish the long-term effectiveness of ileal exclusion in PFIC.
The evidence base for the efficacy of surgical treatments of PFIC is emerging, but it is unlikely that a prospective, randomized trial of these procedures will be feasible. In a recent retrospective analysis of various procedures to interrupt the enterohepatic circulation 18 BSEP and 16 FICI patients experienced less severely scored pruritus after biliary diversion (FIC1: 64% vs. 10% and BSEP 50% vs. 20% preoperatively vs. 24 months postoperatively, respectively) [46]. Three FIC1 and six BSEP patients eventually required liver transplantation.
In another study 22 FIC1 and 35 BSEP patients underwent PEBD [47]. Most FIC1 patients (81%) experienced subjective improvement of pruritus after PEBD, with sustained improvement in 57%. Two common European BSEP mutations, D482G and E297G, are thought to be milder and likely reflect partial functional activity. Patients with these mutations had a 76% sustained response to PEBD compared with a 33% response rate in patients with other mutations. Overall, PEBD also improved biochemical parameters and growth, with substantial variation in individual response. Milder BSEP and FIC1 patients survived longer after diversion without developing cirrhosis, being listed for or undergoing liver transplantation, or dying, compared to BSEP-other patients.
Pharmacologic interruption of the enterohepatic circulation using bile acid transport inhibitors or potent bile acid sequestrants is a theoretical alternative to these surgical approaches, and is covered in Chapter 9. Extreme caution should be considered with the latter approach as low intraluminal bile acid levels can predispose to severe fat-soluble vitamin deficiency with potentially life-threatening coagulopathy. Temporary endoscopic nasobiliary drainage was used to induce long-standing remissions in three adults with BRIC1 who had been refractory to medical therapies [36]. Pruritus disappeared within 24 hours, with normalization of serum bile acid concentrations. This approach might be useful in selected cases to help to determine whether a permanent surgical approach will be efficacious.
A long-term therapeutic goal will be to cure these disorders using precision genome editing approaches such as using CRISPR-Cas9. In the meantime, there has been steady progress to identify novel small molecules or repurposed drugs for mutation specific therapy. The approach will be to restore full-length protein synthesis or rescue impaired protein trafficking and protein degradation. For example, in vitro studies have suggested that 4-phenylbutyrate may rescue missense mutated proteins that underlie some forms of PFIC. Several small studies have provided a proof of principle that this approach may be efficacious. Seven patients with BSEP deficiency had improved liver blood tests, serum bile acids, and pruritus [48, 49]. Improved histology and canalicular BSEP expression were also observed. In contrast, there was only improved pruritus in three treated patients with PFIC1 [50]. Further research is required to identify or repurpose other small molecules including cystic fibrosis transmembrane regulator potentiators and correctors to target specific mutations in the ATP-binding cassette superfamily of transporters.
Ursodeoxycholic acid is frequently given to patients with PFIC1 and PFIC2 in a daily dosage of 10–20 mg/kg. Liver tests may improve, but the drug has little benefit in patients with severe pruritus [9]. Moreover, there is no evidence that UDCA alters the natural history of these disorders, including the need for biliary diversion or liver transplantation, or shortens the duration or frequency of episodes of BRIC.
Liver transplantation remains an option for the management of end-stage liver disease in PFIC and as an alternative approach in patients with refractory and severe pruritus [51]. In BSEP and MDR3 disease, in which the disease is hepatocyte specific, liver transplantation is usually a definitive approach [3]. In contrast, in FIC1 disease, liver transplantation is potentially fraught with a number of potential complications related to the extrahepatic expression of ATP8B1. The most prominent post-transplantation problems include intractable diarrhea, hepatic steatosis, poor growth, and recurrent pancreatitis. The steatosis can be progressive, leading to cirrhosis in less than ten years [13]. Interestingly, post-transplant steatosis and diarrhea was responsive to biliary diversion [52]. Therefore, in FIC1 disease, non-transplantation surgical approaches should be considered the preferred first-line of therapy.
Ten children with PFIC2 have been reported who developed recurrent normal GGT cholestasis mimicking primary BSEP deficiency after liver transplantation [53]. Time to onset of biochemical low GGT, cholestasis, and histologic evidence of cholestasis without rejection ranged from nine months to 17 years after liver transplantation [51, 53]. As in their original disease, there is extensive giant cell transformation of hepatocytes on liver biopsy. When assay was possible, anti-BSEP antibodies were found in these patients that were not found in the serum of patients who had undergone transplantation for BSEP disease and who did not develop recurrence. Antibodies recognize epitopes on BSEP that were critical for bile acid transport and as such this disease process results as a manifestation of antibody-mediated disruption of BSEP function. Genotyping showed genetic defects in ABCB11 that were predicted to lead to a congenital absence of BSEP protein. Therefore, pre-transplant tolerance to native human BSEP by B-cells and/or T-cells was less likely to occur after its introduction in the allograft. Intensification of immunosuppression regimens was variably and typically only temporarily successful in treating recurrent cholestasis, and recurrent disease with circulating high-titer antibodies against BSEP usually occurred after retransplantation. Stem cell transplantation may provide a therapeutic option for cases refractory to conservative treatment. In those cases where a second transplant was performed for “recurrent” disease, recurrence always ensued with progressive disease leading to death. Therefore, clinical decision-making in children with BSEP disease manifest by absent BSEP protein is problematic. One must balance the ongoing risk of development of hepatocellular carcinoma in the native liver with the risk of recurrent disease in a new transplanted liver.
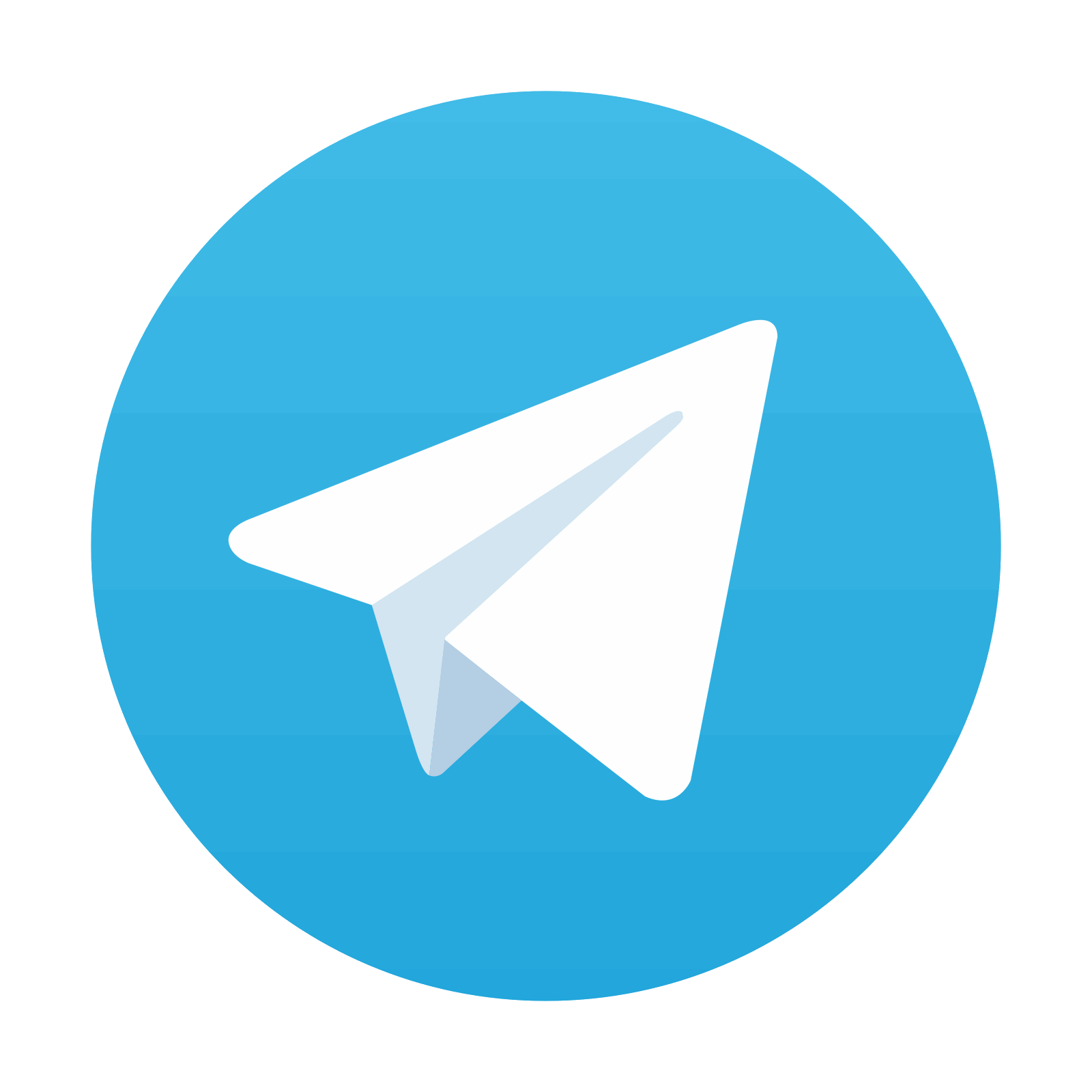
Stay updated, free articles. Join our Telegram channel

Full access? Get Clinical Tree
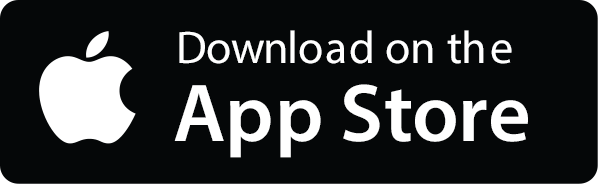
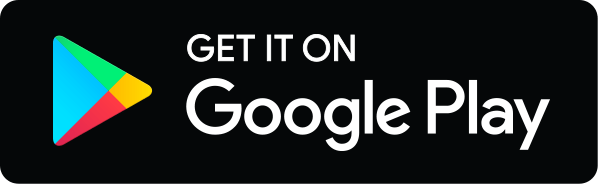