(1)
Department of Surgery, Harvard Medical School, Boston, MA, USA
(2)
Stem Cells and Regenerative Medicine, UCL Institute of Child Health, London, UK
(3)
Division of Neurogastroenterology and Motility, Department of Paediatric Gastroenterology, UCL Institute of Child Health and Great Ormond Street Hospital, London, UK
Keywords
EntericHirschsprung’s diseaseNeuropathiesNeural crestStem cellsAdult stem cellsTransplantationCurrently the therapeutic options for many gastrointestinal (GI) motility conditions, especially the most severe, remain woefully inadequate. For these disorders, treatments are limited to palliative interventions such as surgery and the provision of artificial nutrition. This highlights the fact that current treatments aim to prevent the mortality and limit morbidity associated with the most significant complications of the diseases but are not designed to be curative.
Although it is clear that both surgery and parenteral nutrition (PN ) have revolutionised the management and overall survival of children suffering from severe intestinal motility disorders, most of whom would otherwise not have survived beyond the neonatal period [1–3], these conditions continue to be associated with high levels of morbidity and mortality. Mortality rates still remain in the order of 8–20 % and mostly relate to iatrogenic complications of central venous catheter-related sepsis and PN-related liver failure [1–6].
The poor outcome of gut motility disorders is perhaps best exemplified by Hirschsprung’s disease (HSCR) where despite substantial surgical expertise and relatively rare use of PN, the post-operative morbidity data is compelling [7–12]. A long-term follow-up study of 48 HSCR patients with total colonic aganglionosis (TCA) by Tsuji et al. showed that 94 % survived. Among the survivors, faecal incontinence was present in 82 % of patients at 5 years, 57 % at 10 years and 33 % at 15 years follow-up. On anthropometric follow-up, 63 % of patients with TCA were failing to thrive at 15 years [7]. These findings are supported by a recent systematic review [12]. Other studies suggest that such problems occur irrespective of the extent of aganglionosis [8] and persist in more than 50 % of HSCR patients into early adulthood [9].
Such data highlights the need for improved, more curative, therapies for gut motility disorders, including those designed to definitively restore missing components or rescue dysfunctional ones. With particular attention to enteric neuropathies, this chapter summarises the tremendous progress that has been made, and the challenges that remain, in the development of new curative cellular therapies for gut motility disorders.
Stem Cell Therapies for ENS Disorders: Background and Concepts
Recent advances in molecular biology and genetics have significantly enhanced our understanding of the development and function of the gut neuromusculature, especially its intrinsic innervation, the enteric nervous system (ENS ). This has not only facilitated our appreciation of the pathogenesis of gut motility disorders but has also allowed the identification of novel tools and targets for therapy [13, 14]. Stem cells, defined by their unique ability to self-renew, proliferate extensively and differentiate into multiple lineages, provide one such tool. For the purposes of this review, the term ‘stem cell’ has been used to denote both progenitor cells, with limited self-renewal and differentiation capacities, and stem cells in the truest sense.
Successful stem cell therapy has already been performed for many years in the form of bone marrow transplants, and there is currently enormous interest in the potential of stem cell therapy to treat diseases of both the central nervous system (CNS) [15] and ENS [16–18]. Compared with other systems, the use of stem cell therapy for treating diseases of the ENS has some potential advantages including accessibility to source and deliver cells, as well as the possibility of autologous transplantation.
Sourcing Stem Cells for ENS Therapy
In the quest to develop cellular therapies for ENS disorders, a number of tissue sources have been explored to identify a cell type capable of generating ENS components upon transplantation. These are discussed below and summarised in Table 49.1.
Table 49.1
Possible sources of stem cells to generate a putative ENS
Source | Selection/propagation | Recipient or host tissue | Differentiation in host tissue | Function | References |
---|---|---|---|---|---|
PSC (ES/iPS) | |||||
Mouse ES cells | EB | Mouse renal capsule | N, M, ICC and EP | Regular slow wave activity and spontaneous spike potentials | |
Mouse ES cells | Sox10 | Aneural hindgut explant from mouse embryo in vitro | N | ND | [23] |
Human PSC | SOX10, CD49D | Colon of Ednrb−/− mouse | N+G | Prolonged survival of mice with HSCR | [24] |
CNS | |||||
Embryonic mouse brain | NS | nNOS−/− mice stomach in vivo | N+G | Improved gastric function | |
Embryonic rat brain | NS | Chemically denervated rat rectum in vivo | N+G | Restored rectoanal inhibitory reflex | [27] |
Embryonic rat neural tube | NS | Chemically denervated rat colon in vivo | N+G | Improved colonic motility | [28] |
Neural crest ENS | |||||
Embryonic mouse gut | Sorted Ret+ cells | Aganglionic gut explant from Ret−/− mouse embryo in vitro | N+G | ND | |
Embryonic/postnatal mouse gut | NS | Aganglionic gut explant from Ret−/− mouse embryo in vitro | N+G | ND | [31] |
Postnatal/adult rat gut | Sorted p75+/α4 integrin+ cells | Aganglionic gut explant from Ednrb−/− mouse embryo grown on chorioallantoic membrane of chick embryos | N | ND | |
Embryonic rat gut | Sorted p75+/α4 integrin+ cells | Ednrbsl/sl rat bowel in vivo, i.p. | N+G | ND | [35] |
Embryonic/postnatal human gut | NS | Human gut explant in vitro | N | ND | [36] |
HSCR patient gut | NS | Aneural hindgut explant from mouse embryo in vitro | N+G+ICC | Restored motility patterns to hindgut | |
Postnatal human gut mucosa | NS | Explant from aganglionic region of HSCR patient in vitro | N | ND | [39] |
ENS cell line from immortomice | Sorted p75+ cells | Piebald or nNOS−/− mice colon in vivo | N | Improved colonic motility | [40] |
Embryonic mouse gut | Sox2 | Aneural hindgut explant from mouse embryo in vitro | N | ND | [41] |
Postnatal mouse gut | Sorted ENCCs (Wnt1-Cre/YFP mice) | Wild-type mouse colon in vivo | N+G | Functional integration with host neurons by Ca2+ imaging | [42] |
Sorted ENCCs (Ednrb Kik mice) | Wild-type mouse colon in vivo | N+G | Functioning neurons by intracellular recording | [43] | |
Other NCCs | |||||
Embryonic mouse neural tube | Neural tube explant | Dom/+ mouse colon in vivo, i.p. | N+G | ND | [44] |
Embryonic rat peripheral nerve | Sorted p75+/α4 integrin+ cells | Into migratory pathway of embryonic chickens in ovo | Gut; no, peripheral nerve; N+G | ND |
Embryonic stem (ES) cells derived from the inner cell mass of the blastocyst are pluripotent and capable of giving rise to all the cell types in the body [45]. Their initial discovery [46, 47] and subsequent isolation from human embryos [48] led to significant interest for their use in regenerative medicine, especially given their potential to generate ‘unlimited’ quantities of cells for replacement therapies. ES cells from both mouse (mES) and human (hES) are capable of producing a range of neural cell types [49–55], including enteric neurons [23, 56, 57]. Kawaguchi et al. demonstrated that neural crest (NC) progenitors (Sox10 expressing)) derived from mES cells can colonise and give rise to neurons (Hu and TuJ1 expressing) within explants of aneural hindgut of mouse embryos [23]. Neural progenitors derived from hES cells also appear capable of generating NC-like cells that migrate along normal NC migratory pathways in quail embryos in vivo and colonise explants of embryonic mouse gut in vitro where they give rise to neurons [56, 58].
Apart from neurons, mES cells also appear capable of generating ‘gut-like’ structures [19–21, 59–62]. These structures are 0.2–1.5 mm in diameter and contain an endodermal epithelium, intestinal epithelial stem cells, a layer of smooth muscle cells and interstitial cells of Cajal (ICCs); they also exhibit spontaneous contractions [19–21, 59–62]. Although they show some similarities to normal gut organogenesis [21], the requirement for brain-derived neurotrophic factor (BDNF) for neuron development differs from normal enteric neuron development, which does not require BDNF [63]. It is still unclear whether gut-like structures derived from ES cells will be useful for cell therapy, whereas generation of functioning gut epithelial tissue in vitro will provide a platform to study a wide spectrum of GI research. It has been shown that these ‘organoids’ can be manipulated to mimic human GI diseases, including Menetrier disease; hence they can be used as disease models [62].
CNS-Derived Stem Cells
Although it had long been believed that the CNS in mammals is incapable of regenerating after birth, adult neurogenesis is now well established, including in humans [64–70]. This neurogenesis appears to be affected by a population of self-renewing, multipotent progenitors known as neural stem cells (NSCs) [71, 72]. CNS–NSCs were one of the first cell types tested for ENS therapy as several features were thought to make them suitable [17]. Transplanting CNS–NSCs into the pyloric wall of an animal model of gastroparesis (nNOS−/− mice), Micci et al. showed that these cells predominantly gave rise to neuronal nitric oxide synthase (nNOS) expressing neurons, which resulted in significant improvements in gastric emptying and in electric field stimulation-induced relaxation [25]. Although the mechanisms underlying such improvement of gastric function were unclear, the study provided the first demonstration that NSCs transplanted into the bowel were able to ameliorate a motility disorder [16]. More recently, transplantation of foetal cerebral cortex-derived CNS–NSCs into the rectum of adult rats, where enteric neurons had been destroyed chemically, resulted in the generation of neurons and glial cells, an increase in both the expression of nNOS and choline acetyltransferase (ChAT) and restoration of the rectoanal inhibitory reflex [27].
Cells isolated from the mid-embryonic rat neural tube or ‘neuroepithelial stem cells’ have also been shown to give rise to enteric neurons in vivo in experimental animals similar to that described above [28, 73]. Transplantation of these cells appeared to result in nNOS- and ChAT-expressing neurons and improvements in colonic motility in recipient colons in which the ENS had been chemically destroyed [28, 73].
Neural Crest Stem Cells
Perhaps the most attractive tools for ENS therapy are derivatives of those neural crest (NC) cells that initially gave rise to the ENS itself. This phenomenon is described in detail in earlier chapters. Briefly, during embryogenesis NC cells emigrate from the NC, a transient structure that forms at the dorsolateral surface of the developing neural tube, and migrate along defined pathways to give rise to diverse structures including the ENS [74, 75]. Vagal (hindbrain) NC cells arising adjacent to somites 1–7 [76, 77] enter the foregut and migrate along the developing gastrointestinal tract to give rise to the majority of the ENS [78–80]. The capacity to rescue the ENS appears to be limited to NC cells fated to give rise to the ENS itself [32], and although there is some data to suggest that vagal NC have some therapeutic potential [44], the most promising avenue appears to be the use of NC derivatives isolated from the gut.
Enteric Neural Crest Stem Cells (ENS Stem Cells )
Non-human studies: Several studies have demonstrated that multipotent cells, with the ability to form the ENS when transplanted to uncolonised or aganglionic gut, are present within the gastrointestinal tract during development and into postnatal life [29, 30, 33, 34, 43, 81, 82], including from the ganglionic portion of the gut from an HSCR mouse model (miRet51) [31, 83, 84]. The methodology used to isolate such cells is the culture of dissociated gut to give rise to neurospheres or neurosphere-like bodies (NLBs), akin to stem cell-containing CNS neurospheres. In addition to differentiated neurons and glia, NLBs also contain proliferating undifferentiated cells that not only express putative stem cell markers (e.g. Sox10) but also are capable of self-renewal and giving rise to both enteric neurons and glia. Grafting of postnatal NLBs into aganglionic embryonic mouse gut revealed that donor cells were able to colonise the gut and differentiate into appropriate enteric phenotypes, at the appropriate locations [31].
Recent in vivo studies have shown that ENS stem or progenitor cells have the potential to migrate, proliferate and differentiate into appropriate phenotypes when transplanted into the colon of postnatal mice [35, 43, 85–89]. Such cells can be isolated from the embryonic (E14.5) and postnatal mice gut survived for at least 16 weeks and formed enteric ganglion-like clusters containing neurons and glia. Graft-derived neurons expressed some enteric neuron subtype markers, including NOS, ChAT, calbindin and calretinin. Importantly, intracellular electrophysiological recordings from graft-derived neurons showed that they fired action potentials and received fast excitatory postsynaptic potentials (fEPSPs) demonstrating that the graft-derived neurons had incorporated into the enteric circuitry [43].
Human Studies
A number of groups including ours have reported the harvesting of ENS stem cells from postnatal human gut [36–39, 87, 90–92]. Although initial studies suggested this required full-thickness tissue, our most recent work has shown that gut mucosal biopsies obtained by routine endoscopic procedures can be used as a source of stem cells [39]. Neurospheres were generated in cultures of mucosal tissue from endoscopic biopsies obtained from children from the neonatal period up to 16 years, including HSCR patients (Fig. 49.1). The neurospheres were equivalent to those generated from human embryonic and full-thickness postnatal gut tissue and contained putative ENS stem cells. When transplanted into segments of aganglionic gut, including human HSCR maintained in vitro, the neurosphere-derived cells colonised the recipient gut and generated neuronal phenotypes. These studies highlight a significant advance by identifying a regenerating source of tissue to generate ENS stem cells and confirming the feasibility of autologous transplantation . Although there is data suggesting that transplanted human cells are capable of influencing mouse embryonic gut function [38], it is still unclear if recipient postnatal gut exhibit functional rescue following human ENS stem cell transplantation in vivo [91].


Fig. 49.1
Enteric neural stem cell-containing neurospheres can be harvested from postnatal gut. (a) Fluorescent immunostaining of day 14 cell cultures generated from postnatal mouse gut showing the presence of spherical multicellular aggregates of cells, termed neurospheres. These contain cells positive for Sox10 (red) and for S100 (green). Positivity for both markers (arrow) suggests the presence of glial cells, whereas the presence of cells positive for Sox10 only (arrowheads) suggests neural crest-derived undifferentiated progenitors or stem cells. (b and c) Low-power (b) and high-power (c) bright field images of cell cultures (day 21) generated from dissociated human colonic mucosal biopsies obtained from a 6-year-old patient by conventional endoscopy. The cultures show numerous characteristic neurospheres, which have been shown to contain enteric neural stem cells and can be transplanted into recipient gut
Practical Challenges in Developing Cell Therapies
Although there has been much progress in the sourcing of cells with potential for therapy for gut motility disorders, some key challenges still need to be addressed before effective clinical application. These have recently been discussed in a ‘white paper’ produced by an international consortium of scientific and clinical experts in the field [93].
What Is the Ideal Target Disease?
HSCR has provided the archetypal disease for ENS stem cell therapy. The ENS deficiency (distal intestinal aganglionosis), however, is absolute and extensive, and it is unclear whether replenishment of the complex ENS circuitry is truly achievable. In view of this, perhaps disorders with a less severe anatomical or functional phenotype may be more amenable to therapy.
In oesophageal achalasia , in the early stages of disease, functional and presumably neuronal loss appears more restricted to the lower oesophageal sphincter presenting a smaller therapeutic target. The underlying immunologically mediated pathogenic processes [94], however, may need to be controlled prior to transplantation to prevent destruction of a neo-ENS. In intestinal pseudo-obstruction and slow transit constipation, the overall ENS ‘scaffold’ appears intact but is clearly dysfunctional possibly due to deficiencies of particular elements of the neuromuscular circuitry [95–97]. These elements, once identified, may be easier to replenish than the entire ENS. Generalised involvement of the long gastrointestinal tract may, however, limit success, as would potential limitations in migration of transplanted cells [98, 99]. It is clear that all these potential disease targets need more detailed characterisation of their specific defects and aetiology prior to the development of any tailored replenishment strategies. Recent international initiatives to address these hold promise [100].
It should be noted that complete ENS restitution may not be necessary. Studies of the ageing gut, where despite substantial neuronal loss, a scanty surviving ENS functions in the absence of any overt functional obstruction, suggest that partial ENS reconstitution may be sufficient to restore some balance between inhibitory and excitatory influences within the neuropathic gut [101, 102]. This suggests that delivery of smaller number of appropriate cells may be an acceptable therapeutic goal.
What Is the Ideal Cell Type?
It is likely that the therapeutic requirement for individual disorders will determine which cell source is most suitable, e.g. whether to use multipotent stem cells (e.g. from hES, iPS) or more committed neuronal precursors (e.g. ‘adult stem cells ’ or precursors sourced from gut). Limitations exist for each source ranging from uncontrolled proliferation and tumour formation (ES cells) to restricted harvesting and differentiation potential (adult stem cells).
The production of unlimited quantities of enteric neurons by direct induction of ES cells remains an exciting possibility, but there remains concern about their potential to form tumours [48, 103] and unwanted cell types. Strategies have been proposed to prevent this including partially differentiating ES cells, enriching for appropriate cell types and then screening for undifferentiated cells [103–105]. Certainly it would be advantageous to differentiate them into specific neuronal subtypes before transplantation. Protocols for such specific differentiation from each stem cell type have yet to be established although some progress has been made. Stem cells from foetal brain (CNS–NSCs) also have the ability to divide, form neurospheres and differentiate into neurons and non-neuronal cells [71, 106]. Micci et al. have reported that CNS–NSCs preferentially differentiate into nNOS neurons [25, 26], which may be promising for conditions such as oesophageal achalasia. For many patients, clinical practitioners and the general public at large, however, there are ethical problems associated with the use of hES cells and CNS–NSCs from fertilised human eggs and aborted foetal brain tissues, respectively.
Much focus has therefore shifted onto ‘adult’ stem cells, especially given their presumed role in maintaining and repairing the tissue in which they are found and restricted potential to generate only those cell types (e.g. neurons and glia) of the required tissue (e.g. ENS), which limits the need for cell programming and reducing the risk of generating ‘ectopic’ cell types and malignancy. Such cells, however, are present within much smaller number and appear to have a reduced potential to proliferate. Kruger et al. reported that NC stem cells comprise only <0.2 % of cells within the gut wall of postnatal day 22 rats [34], and human studies have suggested that the generation of ENS stem cell-containing neurospheres declines with increasing postnatal age [39]. Although it is possible to enrich and expand neural stem cells obtained from the ENS [31, 36–39], it is not known whether the therapeutic potential is compromised with prolonged in vitro propagation. The paucity of specific markers for stem cells presents a further potential obstacle for the field. ENS stem cell harvesting has largely been restricted to their isolation within neurospheres , structures composed of a heterogeneous mix of cells consisting of, in addition to the stem cells, differentiated cells including neurons, glia and smooth muscle cells [31, 39]. It may be argued that pure isolation of stem cells is perhaps not necessary as neurospheres exist as potential ready-made stem cell niches and complete therapeutic packages capable of colonising aganglionic gut [31, 37, 39]. However, unless specific isolation is possible, the manipulation of cells within, and generation of targeted cell types from, the heterogeneous cellular pool within neurospheres is likely to be a major problem.
One of the most exciting developments in stem cell science has been the generation of induced pluripotent stem (iPS) cells by the reprogramming of mouse embryonic or adult fibroblasts back to a pluripotent state by introducing four transcriptional factors—Oct4, Sox2, Klf4 and c-Myc [107]. Successful reprogramming of differentiated human somatic cells into a pluripotent state raised the possibility of creating patient-derived stem cells [108], which would bypass both immunological problems and bioethical issues associated with hES cells or those obtained from foetal brains. In terms of the gastrointestinal tract, iPS cells can produce intestinal tissue and gut-like structures in vitro. Three-dimensional intestinal organoids were derived from human iPS cells using activin A treatment to induce endoderm formation, followed by FGF4 and WNT3A manipulations to develop hindgut and intestinal specification [109]. Gut-like structures can also be derived from mouse iPS cells that contain a lumen with three distinct layers (epithelium, connective tissue and muscle layer), neuronal networks and ICCs, and which exhibited spontaneous contractions [110]. It is unknown whether iPS cell-derived gut-like structures or neurons will have any therapeutic relevance for the treatment of enteric neuropathies. Studies will be required to elucidate the mechanisms of reprogramming of somatic cells into enteric neurons using exogenously delivered transcription factors and to establish a method of purifying desired cells with 100 % frequency in vitro. Interestingly, in recent years, protocols have been developed in vitro whereby both human embryonic stem cells (hESCs) and human pluripotent stem cells (hPSCs) can be converted into neural crest cells (NCC ) [111–113]. This was either by the addition of small molecules (SB431542) and Noggin [111] or by activating Wnt signalling (using CHIR99021 (Chir), which works by selectively inhibiting glycogen synthase kinase 3β (GSK-3β) [113, 114] or using the stromal-derived inducing activity of PA6 fibroblast coculture [112]. These culture conditions favour generation of neural crest cells (NCC). The hESC or hPSC-derived NCC could aid in the study of disease pathogenesis, such as defects in cell fate specification, migration and differentiation potential, which in turn could pinpoint area of therapy needed. Most recently seminal work by Fattahi et al. [24] demonstrated that enteric neural crest (ENC) cells can be derived from hPSC. These authors induced neural crest cells from hPSCs followed by treatment with retinoic acid to obtain ENC cells. They showed that hPSC-derived ENC cells gave rise to functioning enteric neurons in vitro and improved survival in Ednrb-null mice (mouse model for HSCR) following transplantation. This work provides significant validation for the use of hPSCs for the treatment of enteric neuropathies. However, it remains unclear how transplanted cells were able to elicit the rescue in this study [24]. It also remains to be determined whether transplanting a slightly mixed population of ENS–NCC would be better than a specific pure population as other cell types may provide factors for maintenance and differentiation. Overall, studies such as the above suggest that the ideal cell type for replacement therapies is likely to be a neural crest cell phenotype rather than a generic stem cell population, and deriving them from stem cells may have the advantage of generating adequate cell numbers. However, much work is needed to investigate the function and safety aspects of these transplants.
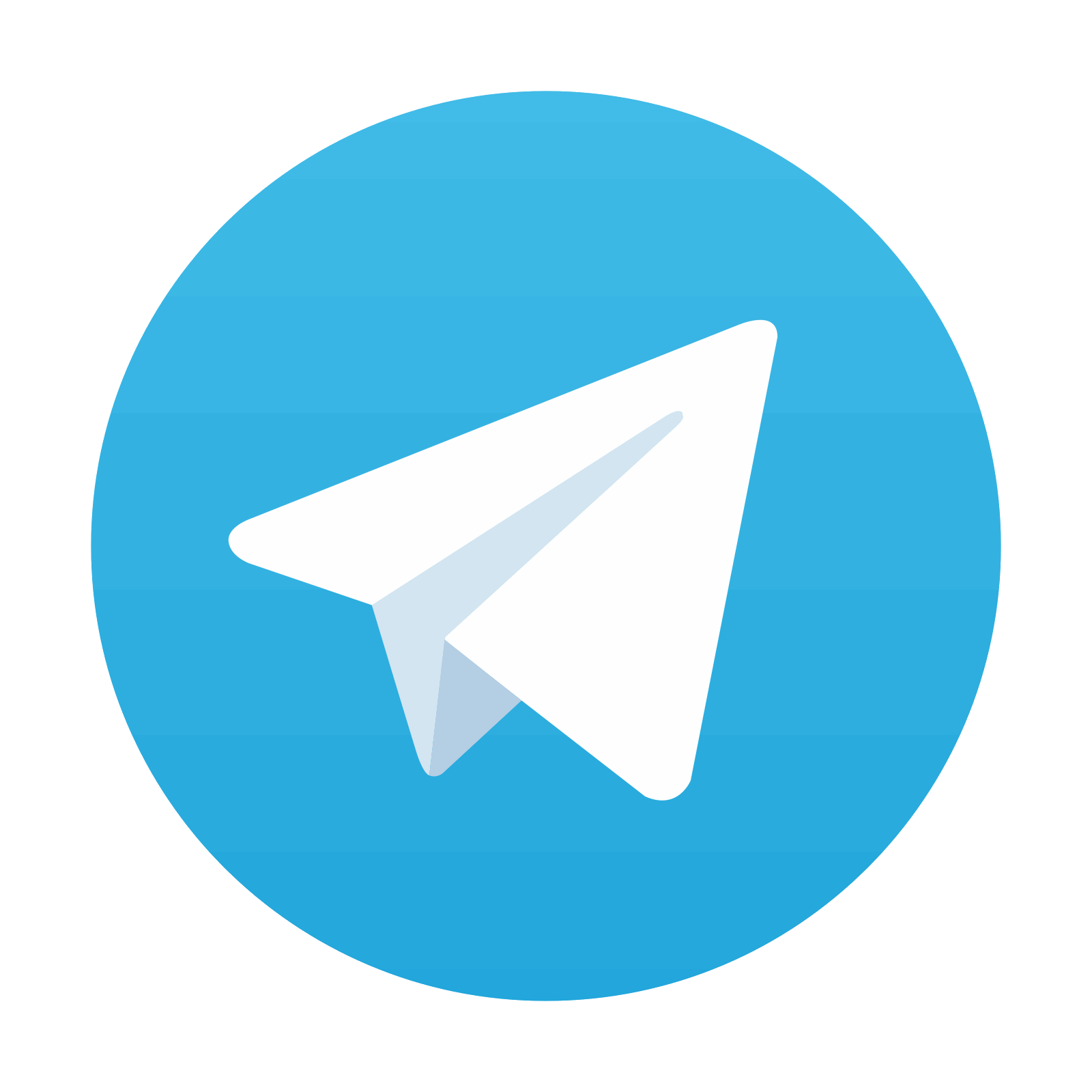
Stay updated, free articles. Join our Telegram channel

Full access? Get Clinical Tree
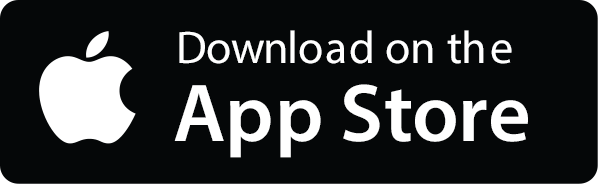
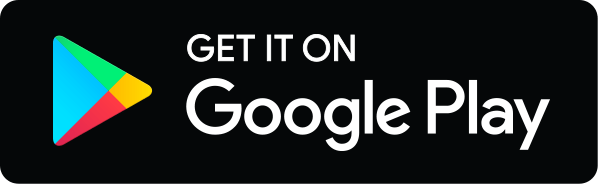