Cells must avoid gross alterations of volume in order to survive. Obviously, excessive cell swelling will jeopardize the integrity of the cell membrane, and both cell swelling and cell shrinkage will interfere with cytoskeletal architecture. Moreover, cellular function critically depends on the hydration of cytosolic proteins. Proteins and protein-bound water occupy a large portion of the cell interior (macromolecular crowding), leaving only a small fraction of cellular volume to free water. Abstraction or addition of only a few percentage points of cellular water thus has profound effects on protein function and cellular performance.
Cells must avoid gross alterations of volume in order to survive. Obviously, excessive cell swelling will jeopardize the integrity of the cell membrane, and both cell swelling and cell shrinkage will interfere with cytoskeletal architecture. Moreover, cellular function critically depends on the hydration of cytosolic proteins. Proteins and protein-bound water occupy a large portion of the cell interior (macromolecular crowding), leaving only a small fraction of cellular volume to free water. Abstraction or addition of only a few percentage points of cellular water thus has profound effects on protein function and cellular performance.
In most mammalian cells, the plasma membrane is highly permeable to water, a property in large part due to virtually ubiquitous expression of water channels in the cell membrane. In theory, driving forces for movement of water include hydrostatic and osmotic pressure gradients. However, the cell membrane is too fragile to withstand significant hydrostatic pressure gradients, and if extension of the cell is not prevented by extracellular constraints the movement of water is governed almost exclusively by osmotic gradients. Thus, to avoid swelling or shrinkage, a cell has to achieve osmotic equilibrium across the cell membrane ( Figure 5.1 ). At excessive intracellular osmolarity, water will enter following its osmotic gradient, and the cell will swell. Conversely, at excessive extracellular osmolarity, water will leave, and the cell will shrink. As outlined in this chapter a wide variety of factors modify intra- or extracellular osmolarity, and thus challenge osmotic equilibrium across the cell membrane.

For maintenance of volume constancy, cells employ a number of mechanisms, including metabolism and altered transport across the cell membrane. These mechanisms may also participate in the regulation of cell function. Hormones and mediators may influence cell volume regulatory mechanisms, and thus manipulate cell volume. Alterations of cell volume will in turn modify other cell volume-sensitive functions. The interplay among cell volume regulatory mechanisms, cell hydration, and cell function does not only contribute to physiological regulation of cellular function, but also participates in the pathophysiology of a wide variety of diseases.
In this chapter a description of cell volume regulatory mechanisms will be followed by a synopsis of factors challenging cell volume constancy, and a discussion on the impact of cell volume regulatory mechanisms on the physiology and pathophysiology of cellular function. Due to space constraints, many excellent original papers on cell volume regulation could not be cited, and the reader is referred to reviews instead. For more detailed analysis of the pertinent original literature, the reader is encouraged to consult previous reviews on cell volume regulation.
Cell Volume Regulatory Mechanisms
Cell volume constancy is challenged by alterations of extracellular osmolarity, by intracellular metabolic generation of osmotically active solutes and by transport across the cell membrane. To counteract those challenges, cells employ a variety of cell volume regulatory mechanisms. Following cell swelling, these mechanisms decrease intracellular osmolarity and cell volume, thus accomplishing regulatory cell volume decrease (RVD). Upon cell shrinkage the mechanisms increase intracellular osmolarity and cell volume, thereby accomplishing regulatory cell volume increase (RVI). Upon slow changes of extracellular osmolarity, the volume regulatory mechanisms may be fast enough to prevent significant alterations of cell volume, leading to isovolumetric volume regulation.
The most powerful mechanisms of cell volume regulation are ion transporters in the cell membrane. As outlined below, uneven ion composition of intracellular and extracellular fluid is one prerequisite for the establishment of osmotic equilibrium across the cell membrane. Furthermore, several ion transport systems in the cell membrane are modified upon alterations of cell volume. Following cell swelling they mediate cellular ion release; upon cell shrinkage they allow cellular ion accumulation to re-establish osmotic equilibrium across the cell membrane.
However, the use of ions in cellular osmoregulation is limited, since high inorganic ion concentrations interfere with the stability of proteins. Beyond that, altered ion gradients across the cell membrane interfere with the function of gradient-driven transporters. For instance, an increase of intracellular Na + activity decreases the chemical gradient for Na + across the cell membrane, and reduces the driving force for Ca 2+ extrusion via the Na + /Ca 2+ exchanger, which increases intracellular Ca 2+ activity. To avoid excessive alterations of intracellular ion concentration, cells also utilize organic osmolytes for osmoregulation. Moreover, cells adapt a variety of metabolic functions, and thus modify the cellular generation or disposal of osmotically active organic substances.
It should be pointed out that a single cell does not usually employ all cell volume regulatory mechanisms described in the following text. In most cases, it is not clear why a given cell selects a certain set of ion transporters and osmolytes without using other mechanisms. Presumably, the large repertoire of cell volume regulatory transporters and osmolytes available enables any given cell to regulate its volume with relatively little impairment of its particular function.
Cell Volume Regulatory Ion Transport
To counterbalance the intracellular osmolarity due to osmotically active organic solutes, such as amino acids and carbohydrates, cell volume regulatory mechanisms need to maintain intracellular concentrations of inorganic ions below extracellular ion concentrations. In addition, cell volume regulatory ion transport systems are employed to counteract alterations of cell volume by appropriate transport of ions across the cell membrane.
Ions in Cell Volume Maintenance
To compensate for the cellular accumulation of organic solutes, cells maintain a low intracellular Cl − concentration. In most cells, Cl − may move across the cell membrane through Cl − channels. Cl − movement through those channels is governed by the cell’s negative potential across the cell membrane, which is built up by asymmetric cation gradients : the cells extrude Na + in exchange for K + by the Na + /K + -ATPase. The cell membrane is, on average, less permeable to Na + than to K + . K + tends to leave the cell through K + channels following its chemical gradient. The exit of K + generates a cell-negative potential difference across the cell membrane, and thus establishes the driving force for the exit of anions such as Cl − . At a cell membrane potential of some −18 mV, for instance, Cl − is in equilibrium at a chemical gradient of 1:2. Accordingly, at an extracellular Cl − concentration of 110 mmol/L, the intracellular Cl − concentration is in electrochemical equilibrium at 55 mmol/L. In theory, such a Cl − distribution would allow the excess accumulation of 55 mmol/L of organic solutes. In most cells the potential difference across the cell membrane is higher (more negative) than −18 mV, and intracellular Cl − is even lower than 55 mmol/L.
As long as the cell membrane is perfectly impermeable to Na + , cell membrane potential, cytosolic Cl − concentration, and cell volume could be maintained constant without continued expenditure of energy. Maintenance of the asymmetric cation distribution requires, however, that any Na + entering the cell is subsequently extruded by Na + /K + -ATPase, an ATP-consuming process.
Ion Release Following Cell Swelling
Ion transport is not only crucial for the establishment of osmotic equilibrium across the cell membrane, but accomplishes rapid correction of any osmotic imbalance across the cell membrane. Following cell swelling, cells must release ions to decrease their osmolarity. Most cells release ions by activation of K + channels and/or anion channels ( Figure 5.2 ). Cell volume regulation requires the operation of both ion channel types, since neither K + nor anions can leave the cells without the respective counterion. Several cell volume regulatory ion channels have been identified at the molecular level, including the K + channels Kv1.3, Kv1.5, Kv4.2, 3, IsK or KCNE1/Q1,4,5, TWIK1, TASK2/KCNK5, TREK1/KCNK2, TRAAK/KCNK4, intermediate or MaxiK (Kca), and the anion channels ClC-2, ClC-3, phospholemman, and bestrophins. The role of other ion channels in cell volume regulation, such as I Cln , P-glycoprotein (MDR) , and CFTR, has been a matter of controversy. Clearly, many different ion channels are likely to contribute to cell volume regulation in various tissues, and the molecular identity of some of those channels is still elusive. Some anion channels not only allow the exit of Cl − , but also of HCO 3 − , organic anions, and noncharged osmolytes.

Swelling of some cells activates unspecific cation channels, including some transient receptor potential (TRP) channels. Since the electrochemical gradient favors entry rather than exit of cations, these channels cannot directly serve cell volume regulation. Instead, the channels allow the entry of Ca 2+ , which in turn activates Ca 2+ -sensitive K + channels and/or Cl − channels.
Besides ion channels, the most important mechanism contributing to regulatory cell volume decrease is KCl co-transport by one of the four members of the KCC family, which allows coupled cellular release of both ions.
Some cells release cellular KCl via parallel activation of K + –H + exchange and Cl − –HCO 3 − exchange. The H + and HCO 3 − thus taken up in exchange for KCl react via H 2 CO 3 to produce CO 2 , which can easily leave the cell again.
Water efflux during volume regulatory decrease could be fully accomplished by existing water channels. Nevertheless, aquaporins participate in cell volume regulation by modifying the activity of ion channels.
Ion Uptake upon Cell Shrinkage
Cell shrinkage activates the Na + –K + –2Cl − co-transporters NKCC1, and NKCC2, and/or the Na + /H + exchangers NHE1, NHE2 or NHE4 parallel to the Cl − ⧸HCO 3 − exchanger AE1. The parallel activation of Na + /H + exchangers and Cl − /HCO 3 − exchangers leads to uptake of NaCl, while the H + and HCO 3 − lost in exchange for NaCl are replenished in the cell from CO 2 via H 2 CO 3 ( Figure 5.2 ). The epithelial Na + /H + exchanger NHE3 is inhibited by cell shrinkage. The Na + ions accumulated by either Na + –K + –2Cl − co-transport or Na + H + exchange are extruded by Na + K + -ATPase in exchange for K + . Accordingly, the transporters eventually lead to cellular KCl uptake.
Several cells activate Na + channels or unspecific cation channels following cell shrinkage. The channels may include some members of the transient receptor potential (TRP) channel family or ENaC. The resulting depolarization drives Cl − into the cell, so that the net effect is cellular accumulation of NaCl. Other cells inhibit K + and/or Cl − channels to avoid cellular ion loss.
Osmolytes
The most important osmolytes are polyols such as sorbitol and myoinositol, methylamines such as betaine and glycerophosphorylcholine, as well as amino acids including taurine. In contrast to inorganic ions, organic osmolytes do not destabilize proteins, but rather stabilize them. They counteract the destabilizing effects of inorganic ions, some organic ions (e.g., spermidine), and urea. The stabilizing potency of the diverse organic osmolytes is not identical. The destabilizing effects of urea are counteracted most efficiently by betaine and glycerophosphorylcholine, and less efficiently by myoinositol. The stabilizing effects of osmolytes may protect proteins against excessive ion concentrations, as well as against heat shock, freezing, desiccation, and presumably radiation.
Osmolyte Accumulation by Metabolism
Sorbitol is generated from glucose under the catalytic action of aldose reductase. Stimulation of the transcription rate of the aldose reductase during osmotic cell shrinkage leads to cellular accumulation of sorbitol. The expression of the protein takes many hours, and the appropriate increase of sorbitol concentration requires hours to days.
Glycerophosphorylcholine (GPC) is produced from phosphatidylcholine under the catalytic action of a phospholipase A 2 , which is distinct from the arachidonyl selective enzyme. GPC is degraded by a phosphodiesterase to glycerol-phosphate and choline. Inhibition of the phosphodiesterase during cell shrinkage leads to cellular accumulation of GPC.
Osmolyte Accumulation by Transport
Myoinositol (inositol), betaine and taurine are taken up by specific Na + -coupled transporters SMIT (inositol), BGT (betaine), and NCT (taurine), respectively. The carriers accumulate Na + , the respective organic osmolyte, and in the case of BGT and NCT, Cl − as well. Movement of excess positive charge by the carriers depolarizes the cell membrane, thus favoring entry of Cl − via anion channels. The transporters thus mediate uptake of NaCl parallel to organic osmolytes. The transcription rate of the transporters and the cellular accumulation of the respective osmolytes are stimulated by osmotic cell shrinkage. Expression of the transporters is slow, and full adaptation requires hours to days. Moreover, volume regulation by activation of transport systems depends on the availability of osmolytes in the extracellular fluid.
Analogous to osmolytes, some amino acids are accumulated by cell volume-sensitive, Na + -coupled transport. In addition, amino acids could be generated by autophagic proteolysis, as discussed in the next section.
Osmolyte Release
Cell swelling stimulates the rapid release of GPC, sorbitol, inositol, betaine, and taurine. The mechanisms mediating osmolyte release are still poorly understood. Clearly, several mechanisms are simultaneously operative. At least some of the release mechanisms are thought to be anion channels. As shown for taurine, the osmolyte release could be triggered by oxidative stress.
Metabolic Pathways Sensitive to Cell Volume
Alterations of cell volume influence a variety of metabolic pathways. The effects of cell volume on metabolism are accomplished in part by activation and inhibition, and in part by altered expression of enzymes.
Protein and Glycogen Metabolism
Cell shrinkage stimulates the breakdown of proteins to amino acids, and of glycogen to glucosephosphate. Moreover, cell shrinkage inhibits protein and glycogen synthesis. The sum of amino acids generated during proteolysis is osmotically more active than the osmolarity of the respective protein. Thus, net formation of macromolecules decreases, and net degradation of macromolecules enhances, cellular osmolarity. Cell swelling stimulates protein and glycogen synthesis, and inhibits proteolysis and glycogenolysis, thus decreasing the intracellular concentration of amino acids and glucosephosphate.
Glucose and Amino Acid Metabolism
In addition to affecting protein and glycogen metabolism, alterations of cell volume influence several pathways of glucose and amino acid metabolism. Cell swelling inhibits glucose uptake and glycolysis, stimulates flux through the pentose phosphate pathway, and favors lipogenesis from glucose, effects reversed by cell shrinkage. Transcription of phosphoenolpyruvate carboxykinase, a key enzyme for gluconeogenesis, is decreased by cell swelling. Cell swelling stimulates glycine and alanine oxidation and glutamine breakdown, as well as formation of NH 4 + and urea from amino acids; these effects are reversed by cell shrinkage.
Oxidative Metabolism
The stimulation of flux through the pentose phosphate pathway during cell swelling enhances NADPH production, which favors the formation of glutathione (GSH). Cell shrinkage decreases NADPH production and GSH formation. Accordingly, cell swelling increases and cell shrinkage decreases cellular resistance to oxidative stress. On the other hand, cell swelling stimulates, and cell shrinkage decreases, the activity of NADPH-oxidase, thus modulating cellular O 2 − formation. Accordingly, leukocyte oxidative burst, and thus immune response, is blunted by the high osmolarity of kidney medulla.
Other Metabolic Pathways
Cell swelling stimulates ketoisocaproate oxidation, acetyl CoA carboxylase, and lipogenesis, and inhibits carnitine palmitoyltransferase I. It decreases cytosolic ATP and phosphocreatine concentrations, and increases respiration. Cell swelling stimulates RNA and DNA synthesis. All of these effects are reversed by cell shrinkage.
Cell Volume-Sensitive Genes
Cell volume influences the transcription of a wide variety of genes, leading to differential expression of a wide variety of proteins. Transcription factors involved include AP1, c-FOS, cJUN, tonicity-response enhancer binding protein TONEBP, serum response factor (SRF), and myocardin related transcription factor. Many of the cell volume-regulated genes are related to cell volume regulation. Accordingly, cell shrinkage stimulates expression of enzymes or transporters engaged in cellular formation or accumulation of osmolytes, such as aldose reductase, and the Na + -coupled transporters for betaine (BGT), taurine (NCT), myoinositol (SMIT), and amino acids, as well as Na + ,K + ,2Cl − co-transport (see above). Moreover, cell shrinkage upregulates the expression of the ATPase α1-subunit.
The products of other genes may be involved in the signaling of cell volume regulatory mechanisms, such as the kinases ERK1, ERK2, and JNK-1, which are expressed during cell swelling or the serum and glucocorticoid inducible kinase SGK1 and cyclooxygenase-2, which are preferably expressed in shrunken cells.
Heat shock proteins serve to stabilize proteins, and their expression in shrunken cells may counteract the destabilizing effects of accumulated ions. Several genes expressed in response to altered cell volume do not have obvious roles in cell volume regulation. Cell swelling stimulates the expression of the cytoskeletal elements β-actin and tubulin, the immediate early genes c-jun and c-fos, the enzyme ornithine decarboxylase, and the cytokine TNF-α.
Cell shrinkage stimulates the expression of the channels CIC-K1, the transporter P-glycoprotein, the immediate early genes Egr-1 and c-fos, the GTPase inhibitor α1-chimaerin, the CDβ antigen, the enzymes phosphoenolpyruvate carboxykinase (PEPCK), arginine succinate lyase, tyrosine aminotransferase, tyrosine hydroxylase, dopamine β-hydroxylase, matrix metalloproteinase 9, tissue plasminogen activator, as well as matrix proteins including biglycan and laminin B 2 . Cell shrinkage stimulates both expression and release of ADH, which serves to eliminate water and thus increase extracellular osmolarity.
The mechanisms mediating the altered gene expression are beginning to be understood. The promoter region of the genes encoding aldose reductase, BGT, TAUT, and SGK1 have been ascribed to contain osmolarity responsive (ORE), tonicity responsive (TonE) or cell volume-responsive (CVE) elements, which are required for osmolarity or cell volume-sensitive expression of the respective genes. TonE has been shown to bind a tonicity-responsive element-binding protein (TonEBP) for stimulation of expression. TonEBP is regulated by ataxia teleangiectasia-mutated (ATM) kinase.
Signaling of Cell Volume Regulation
The stimulation of effectors of cell volume regulation requires that alterations of cell volume or osmolarity are perceived and trigger a signaling cascade, eventually leading to stimulation of cell volume regulatory mechanisms.
Little is known about sensors of cell size and hydration. Circumstantial evidence points to the ability of cells to determine cellular protein content or macromolecular crowding. It has been speculated that macromolecular crowding directly or indirectly regulates kinases which in turn influence the activity of cell volume regulatory KCl and Na + –K + –2Cl − co-transport (see below). Alternatively, alterations of ionic strength or the concentration of individual ions such as Cl − modify volume regulatory mechanisms. Moreover, alterations of cell size are thought to impose stretch on the cytoskeleton and/or cell membrane or change the curvature of the cell membrane, again leading directly or indirectly to activation of cell volume regulatory mechanisms. Cell swelling leads to unfolding of the cell membrane and, if this does not suffice, to endomembrane insertion. Alterations of cell volume could be further sensed by cytokine and Ca 2+ sensing receptors, as well as by integrins.
A multitude of signaling pathways link the alterations of cell volume and cell volume regulatory mechanisms. The respective signaling varies considerably between different cells or a given cell in different functional states.
Intracellular Ca 2+
Cell swelling increases intracellular Ca 2+ activity in many, but not all, cells. Ca 2+ enters through Ca 2+ channels in the plasma membrane, and/or is released from intracellular stores triggered by 1,4,5-inositol-trisphosphate. The channels involved include nonselective Ca 2+ channels, Ca 2+ permeable members of the TRP (transient receptor potential) channel family, such as TRPV4, and L-type voltage gated Ca 2+ channels. Ca 2+ in turn activates some cell volume regulatory K + channels and Cl − channels. In addition to its involvement in regulatory cell volume decrease, intracellular Ca 2+ may mediate some of the functional consequences of cell shrinkage.
Cytoskeleton
Alterations of cell volume modify the architecture of the cytoskeleton and the expression of cytoskeletal proteins. Cell shinkage increases and cell swelling decreases actin polymerization. Both microtubules and actin filaments have been implicated in cell volume regulation. In several cells, disruption of actin filaments and/or the microtubule network have been shown to interfere with cell volume regulation.
Protein Phosphorylation
Cell swelling and cell shrinkage have both been shown to modify the phosphorylation of a variety of proteins. Kinases reported to be activated during cell swelling include tyrosine kinases, protein kinase-C, phosphoinositide (PI) 3 kinase, Jun-kinase, and extracellular signal-regulated kinases ERK-1 and ERK-2, as well as focal adhesion kinase (p121 FAK ). Activation of PI3 kinase leads to stimulation of protein kinase B (Akt), and serum and glucocorticoid inducible kinase isoforms, which modify a wide variety of carriers and channels. Expression of SGK1 is, however, downregulated by cell swelling, and thus SGK1 dependent signaling is disrupted. In Jurkat lymphocytes, cell swelling leads to activation of the src-like kinase lck, which in turn activates the cell volume regulatory Cl − channel ORCC.
Osmotic cell shrinkage stimulates WNK (with no lysine kinase) 1 and 4, which in turn activate Ste-20-related proline alanine rich kinase (SPAK) and oxidative stress responsive kinase (OSR1). SPAK and OSR1 activate the Na + -K + -2Cl − co-transporters NKCC1 and NKCC2. Conversely, WNK4 inhibits KCl co-transporters. WNK1 activates SGK1, which in turn inhibits WNK4. Osmotic cell shrinkage further triggers several MAP (mitogen-activated protein) kinase cascades, and activates SAPK, p38 kinase, myosin light-chain-kinase (MLCK), Jun kinase (JNK), p21-activated kinases PAKs Rho kinase, LIM kinase, and casein kinase. MLCK may modulate the cytoskeleton, and thus cell volume regulatory ion transport. Moreover, the kinase cascades lead to activation of transcription factors governing expression of cell volume-regulated genes. Hyperosmolarity activates the tyrosine kinase Fyn-dependent phosphorylation of caveolin, which in turn inhibits volume-sensitive Cl − channels.
Phosphatidylinositol 4,5,-Bisphosphate
Cell swelling decreases and cell shrinkage increases the formation of phosphatidylinositol 4,5 bisphosphate (PtdIns(4,5)P 2 ) by the phosphatidylinositol 4-phosphate 5-kinase beta isoform (PIP5KIbeta). Among other effects, PtdIns(4,5)P 2 stimulates the transient receptor potential channel TRPV1, Na + /H + exchanger NHE1, the Na + /Ca 2+ exchanger NCX, and the Na + channel ENaC, and inhibits the transient receptor potential channel TRPC6. PtdIns(4,5)P 2 may further foster actin polymerization by inhibiting the monomer-binding protein profilin, the severing protein cofilin, the capping protein gelsolin, as well as the Wiskott–Aldrich syndrome protein (WASP).
Phospholipase A 2 and Eicosanoids
Cell swelling activates phospholipase A 2 and subsequently stimulates the formation of the 15-lipoxygenase product hepoxilin A 3 , and the 5-lipoxygenase product leukotriene LTD 4 . Phospholipase A2 is phosphorylated by casein kinases CK1 and CK2, protein kinase A, protein kinase C, and mitogen activated kinases. In some cells the eicosanoids generated following ativation of phospholipase A 2 stimulate cell volume regulatory K + ,Cl − channels, and/or taurine release. Enhanced formation of leukotrienes parallels decreased formation of PGE 2 , with subsequent decrease of Na + channel activity. Conversely, osmotic cell shrinkage may stimulate formation of PGE 2 , with subsequent activation of PGE 2 -sensitive Na + channels. In other cells PGE 2 may activate volume regulatory K + channels. In erythrocytes, activation of phospholipase A 2 by hyperosmotic shock leads to release of platelet-activating factor PAF, which in turn activates a sphingomyelinase, thus stimulating ceramide formation.
pH of Acidic Cellular Compartments
In all cells studied thus far, swelling alkalinizes and cell shrinkage acidifies acidic cellular compartments, presumably including endosomes, lysosomes, and secretory granules. This effect is apparently mediated by the microtubules, since it is abolished by disruption of the microtubule network. The alkalinization of the acidic cellular compartments may contribute to the antiproteolytic action of cell swelling, since the pH optimum of lysosomal proteases is in the acidic range, and lysosomal alkalinization has indeed been shown to inhibit proteolysis.
Others
Several G-proteins have been implicated in cell volume regulation including Rho, Rac, and Ras. Hyperosmotic cell shrinkage further activates ezrin/radixin/moesin (ERM) proteins, which in turn mitigate the shrinkage-induced activation of NHE1, augment Rho activity, and presumably modify actin architecture. Cell swelling may trigger release of ATP, which in turn leads to autocrine stimulation of purinergic receptors with the respective activation of cell volume regulatory ion channels.
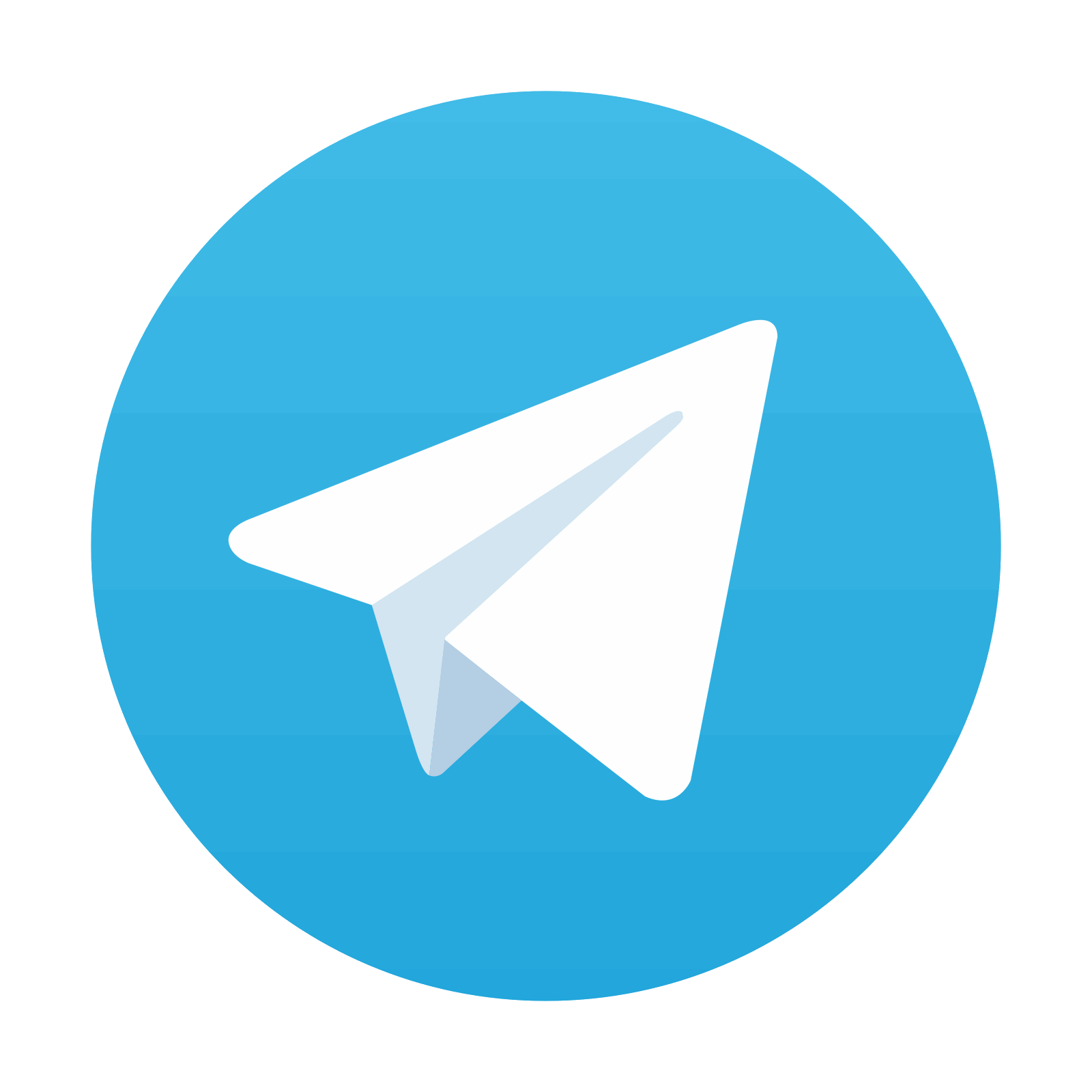
Stay updated, free articles. Join our Telegram channel

Full access? Get Clinical Tree
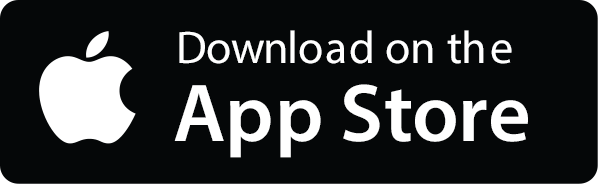
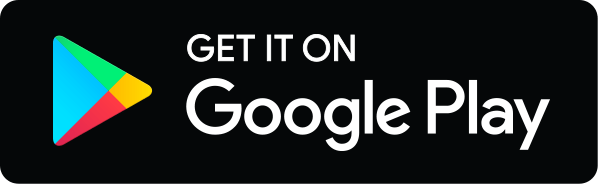