The availability of high-field-strength magnets, high-performance-gradient hardware, and ultrafast sequence technology in recent years has transformed cardiac magnetic resonance imaging (MRI) into a multifunctional tool. Cardiac MRI can provide three-dimensional analysis of cardiac anatomy, viability, motion, and function with high accuracy and reproducibility.1,2 Because of the complexity of cardiac anatomy and motion, cardiac MRI can be challenging and previously had been performed primarily at specialized centers. More recently, a comprehensive cardiac MRI exam has become a reliable clinical tool in a wide range of healthcare centers. In this article, we briefly outline imaging techniques and illustrate the various applications of cardiac MRI.
Communication between the surgeon and the MRI center is essential for efficient and accurate diagnosis. The examination will then be tailored to the specific clinical question. Instructing patients as to the nature of the examination will likely significantly improve the quality of the test. During the MRI examination, patients will need to perform multiple breath-holds of about 10- to 20-s duration. Patients with severe shortness of breath or who cannot lie flat on the MRI table are often poor candidates for cardiac MRI. Such patients should be discussed with the MRI center prior to referral. Overall, a directed examination requires about 20 to 30 min, whereas a comprehensive examination will require 45 to 60 min. There are no restrictions on eating or drinking before the MRI examination except if stress examinations are performed (see below).
Compatibility of a patient’s devices with the MRI scanner is a frequent issue and changes frequently with technology development. In general, all prosthetic cardiac valves are MRI compatible. Pacemakers and implantable cardioverters/defibrillators (ICDs) have previously been considered as strict contraindications to MRI. However, there have been recent reports at our institution and others of patients who have safely undergone MRI at 1.5 T magnetic field strength with certain types of these devices in place.3,4 In these instances, imaging information from other techniques, such as computed tomography or echocardiography, was incomplete. Currently, MRI scanning with pacemakers or ICDs in place remains investigational. Older devices manufactured before the year 2000 and certain manufacturers’ devices are less likely to be MRI compatible. Lower magnetic field strength (i.e., 1.5 T instead of 3 T) should also improve safety in conjunction with implanted devices. Thus, consultation with the MRI center should be performed when an MRI examination is deemed essential to patient care.
Coronary and other vascular stents are increasingly common. Most stents have not been explicitly tested by the manufacturer for MRI compatibility. Package labels from the manufacturer may indicate that MRI may be performed 6 weeks after implantation, with the rationale that the stent is endothelialized at that time and less likely to move in the magnetic field. Our approach is to evaluate these devices on a case-by-case basis. In many instances, the risk of performing the MRI examination with such a device may be outweighed by the benefit of performing the examination. Lists of devices that have been tested for MRI compatibility have been published in the literature as well as listed on websites, such as www.MRIsafety.com.
There is no known adverse effect of MRI on the fetus; MRI examination is frequently preferred compared to imaging examinations using x-rays. The decision to scan during pregnancy should be made on an individual basis. The gadolinium-based contrast agents used for MRI cross the placenta, so deferring the examination to a later trimester or after the pregnancy would also reduce any potential risk.5 If an MRI exam has to be performed during pregnancy, we usually can avoid giving MRI contrast agents to answer a specific clinical question in most patients at our center.
Patients with renal failure should not undergo gadolinium- enhanced MRI, because they are at risk of developing nephrogenic systemic sclerosis (NSF). NSF is a rare but potentially debilitating or fatal fibrosing condition that has recently been linked to gadolinium-based contrast agents. For patients with chronic renal failure, the FDA has determined that the risk is greatest when the estimated glomerular filtration rate (GFR) is less than 30 mL/min/1.73 m2. The policy at our institution is therefore generally not to administer intravenous gadolinium-based contrast agents in patients with chronic severe renal failure (GFR less than 30 mL/min/1.73 m2), acute renal failure or in patients on dialysis.6
If cardiac stress imaging is performed with dobutamine, adenosine, or dipyridamole, the patient should be questioned about relative contraindications to these agents and specifically counseled to avoid theophylline-containing drugs or foods for at least 24 h prior to the exam. A gadolinium-based contrast agent is frequently used in association with cardiac MRI. Reactions to the MRI contrast agent are very rare, but a prior severe reaction would constitute a contraindication to another contrast injection.
Cardiac MRI makes use of electrocardiographic (ECG) gating to suppress cardiac motion artifacts and arterial pulsation. The objective of cardiac gating is to acquire an R wave that is taller than the S or T wave. Even after obtaining a strong ECG signal initially, the signal may become obscured by additional noise from the magnetic field or regurgitant fraction (RF) pulses. Therefore optimal electrode-to-skin contact is necessary and skin shaving and cleansing is often necessary at the electrode placement sites. Nevertheless, imaging with an impaired ECG signal (i.e., pericardial effusion) or cardiac arrhythmias can be challenging.7 For patients with arrhythmias who can tolerate β-blockade, a short-acting agent (e.g., metoprolol) may be administered 30 min before the MRI examination.
Terminology in MRI can be quite confusing to both the cardiovascular imager and the cardiovascular surgeon. The method of creating the images is termed the “pulse sequence,” and pulse sequence names often change in order to give a manufacturer a perceived marketing advantage over competitors. Therefore a brief review of some principles underlying the terminology is worthwhile.
Images may be rather simply classified by the relative brightness of blood in the vessels or in the ventricular chambers. The blood can be made to be dark (“black-blood images”) when the ventricular or arterial walls are the primary focus of the examination (Fig. 57-1). For example, to visualize a myocardial infarction, it is useful to make the blood in the ventricle very dark so as not to obscure the ventricular wall. Although it is entirely acceptable to refer to such images as “black-blood images,” more specific terminology can be used to indicate how the image was generated. Thus, the term black-blood image is a very generic way of describing more specific terminology such as fast spin-echo or turbo spin-echo images.
The most important type of black-blood image generation for the cardiovascular system is termed a double-inversion recovery fast spin-echo sequence. These images are acquired in a breath-hold and are used to image myocardial edema, tumors, or complex anatomic regions. The method involves a specific pulse (“inversion pulse”) that is used to make the blood dark relative to the myocardium. Advantages of the method include very high soft tissue contrast images, similar to those seen in brain or musculoskeletal imaging. Spatial resolution is usually quite good, between 0.5 and 2 mm. The disadvantage is a relatively long imaging time. In addition, the images are static rather than cine images.8
Black-blood magnetic resonance (MR) images can be further categorized. “T1-weighted” MR images display myocardium and fluid as intermediate signals and fat as bright signals. T1-weighted MR images are also used to show enhancement of abnormal tissues after intravenous administration of a gadolinium-based MR contrast agent (discussed further below). “T2-weighted” MR images show fluid and edema as bright signals. These are most commonly used to depict inflammation, tumor edema, or edema from myocardial infarction.
Besides black-blood images, the most commonly used MR images are “bright-blood images” (Fig. 57-2). Typically, these are viewed as cine images of the ventricular or valvular planes. These methods have the advantage of taking less time to acquire than the black-blood images; thus visualization of motion is possible. The temporal resolution of these images is 25 to 50 ms or less if needed.
Figure 57-2
By imaging in the plane through the left ventricle and left atrium prescribed by the white bar on the transverse view (A), an oblique vertical long-axis two-chamber view of the left ventricle and left atrium is obtained (B). The long-axis view is acquired with the bright-blood (FIESTA) MRI technique (B). LA, left atrium; LV, left ventricle.

The bright-blood cine images are generated using a method generally known as gradient echo (GRE) imaging. The newest GRE methods have trade names such as balanced fast-field echo, FIESTA, or TrueFISP, depending on the vendor of the MR scanner. These sequences carry specific advantages for cardiac MR and represent a substantial improvement in image quality compared to older methods of cardiac cine imaging.9 The spatial resolution of the images is about 2 mm, which is substantially greater than the resolution possible with nuclear techniques (6 mm). The blood pool in the ventricle has a very bright signal, so that the border between the ventricle and blood pool is easily distinguished. Turbulent jets at sites of stenosis or regurgitation are dark and readily distinguished using the newest sequences. GRE images are also used for imaging myocardial infarctions.
Disadvantages of bright-blood GRE images include large amounts of image distortion around metal, including sternal wire implants, stents, and embolization coils. The most frequent problem we have observed arose in patients with congenital heart disease who had embolization coils placed and required later evaluation with MRI. These coils distort large areas of anatomy, with the area of distortion centered on the device.
Other techniques—such as flow-sensitive MRI, myocardial viability techniques, and vascular imaging—are discussed later in this chapter.
Since the cardiac axes differ significantly from the axial, sagittal, and coronal planes of the body, specific imaging planes are necessary for adequate cardiac visualization and evaluation. Recently, three-dimensional acquisitions have been developed, so that the heart can be sliced and viewed from any desirable angle at postprocessing. This further shortens the acquisition time. A brief illustration of key imaging planes and how these images are derived is provided below.
The first imaging plane is usually a transverse image of the chest with black-blood techniques (Fig. 57-1). Radiologists and surgeons are most familiar with this plane; it not only permits evaluation of the anatomic position of the cardiac chambers, coronary artery origins, and great vessels, but also detects extracardial intrathoracic pathology. Further sagittal and coronal planes can be obtained. The coronal plane is useful in evaluating the left ventricular outflow tract, aortic valve anatomy, diaphragmatic portion of the left ventricular wall, and the extension of the pericardium over the proximal portion of the great vessels. The plane parallel to the aortic arch on axial images is called the double-oblique or oblique sagittal plane. This plane is used to evaluate the aortic arch and aortic dissection.
The vertical long-axis plane, sometimes referred to as a two-chamber view, is named based on visualization of the left ventricle and left atrium (Fig. 57-2A and B). The mitral valve is readily depicted in this plane.
The horizontal long-axis plane, sometimes referred to as a four-chamber view, is oriented 90 degrees to the vertical long-axis view. This view is obtained from a line drawn through the posterior wall of the left atrium, the middle of the mitral valve, and the left apex on the two-chamber view (Fig. 57-3A and B). The horizontal long-axis view depicts the right and left atria, both ventricles, and the mitral and tricuspid valves. This view also displays the relationship of the four chambers on a single image. Cine GRE images in this plane can evaluate mitral and tricuspid valve function, atrial or ventricular septal defects (VSDs), and bilateral ventricular contractions.
Images in the short-axis plane are perpendicular to the left ventricular long axis seen on the horizontal or vertical long-axis views (Fig. 57-4A and B). The short-axis images show the cross-sectional anatomy of the right and left ventricle. Short-axis cine images are ideal to evaluate left and right ventricular mass, volumes, and function in order to obtain estimates of end-diastolic volume (EDV), end-systolic volume (ESV), and ejection fraction (EF). Differences in stroke volume from the left heart compared to the right heart quantify shunt volumes or valvular regurgitation. Furthermore, this plane is suitable for identifying focal wall motion abnormalities.
Left ventricular mass is a strong independent predictor of fatal and nonfatal cardiovascular events.10,11 Cardiac MRI is currently the most accurate and reproducible method for the assessment of cardiac volumes, mass, and function.12 To evaluate the left ventricular mass, short-axis cine MR images are used. The endocardial and epicardial contours of the left myocardial wall are automatically or semiautomatically traced on each slice using special software. The contours of the cardiac chambers are then summed and multiplied by the slice thickness and slice gap (Simpson method) to obtain the contour volumes (Fig. 57-5). Finally, left ventricular wall mass is calculated from the volumes based on a myocardial density of 1.05 g/cm3. By tracing ESV and EDV, cardiac function parameters like EDV, ESV, and EF can be calculated according to the same principle.
Cardiac MRI using bright-blood cine imaging is used to assess cardiac wall motion. Most centers grade wall motion visually, as either normal, hypokinetic, akinetic, or dyskinetic at the base, middle, and apex of the left ventricle. Qualitative assessment of wall motion by MRI, like that of echocardiography, depends on the use of experienced readers for greatest reproducibility.
A limited number of centers have experience using “tagged MRI” to quantitatively measure regional cardiac wall motion. These methods, developed by Zerhouni and colleagues,13 represent the most precise way for measuring both systolic and diastolic function. The method involves placing a magnetic strip on the myocardium at end diastole. As the heart contracts, the magnetic strip moves and bends along with the motion and contraction of the myocardium. Measuring the distances between the magnetic strips on each image assesses regional contractility. By using these methods, the contraction of the heart in any direction (circumferential, radial, and longitudinal) may be accurately measured (Fig. 57-6). Currently the primary use of quantitative methods to measure regional cardiac contraction has been in specific research protocols. Given the nature of cardiovascular imaging, however, it is likely that these methods will gain further importance in the future. Tagged MRI has other specialized uses, such as the detection of pericardial to myocardial adhesions in constrictive pericarditis.
Conventional and transesophageal echocardiography are still the most widely applied methods to evaluate valvular cardiac anatomy, such as leaflet quantity and thickness, vegetations, and valvular function. Recent developments in cardiac MRI techniques demonstrate that valve evaluation by MRI can be an attractive alternative or complementary to echocardiography. MRI evaluation is noninvasive and provides three-dimensional anatomic and functional data, which are potentially more accurate and reproducible than that of echocardiography.14,15
Black-blood sequences are used to analyze valvular anatomy (e.g., valve thickening or bicuspid valves). Cine MRI bright-blood techniques are used to evaluate abnormal flow patterns as seen in valvular stenosis or regurgitation, which cause loss of signal and appear as a dark signal void (Fig. 57-7). This signal void is caused by turbulent flow and the acceleration of flow within the normal bright-blood pool, similar to aliasing in Doppler ultrasonography. This signal void also depends on technical factors, which may vary in appearance depending on the MRI scanner. Thus, this signal void is usually evaluated only qualitatively. The degree of valvular regurgitation is measured by the area of the signal void volume and the maximum length of the signal void in the receiving chamber.
A method to quantitate valvular regurgitation is to calculate the RF with ventricular volumetric measurements. As described above, end-systolic and end-diastolic volumetric measurements of the blood pool are first performed. In the normal heart, the right and left ventricular stroke volumes (EDV – ESV) are equal. If there is unilateral valvular regurgitation, the EDV is higher on the affected side than on the unaffected side of the heart; therefore the stroke volume on the affected side is also higher. The difference in stroke volume between a regurgitant and a normal ventricle is the regurgitant volume.
The RF can be calculated by dividing the regurgitant volume by the stroke volume of the regurgitant ventricle. An RF of 15 to 20 percent corresponds to mild valvular regurgitation; an RF of 20 to 40 percent to moderate regurgitation; and an RF of more than 40 percent to marked regurgitation. In the presence of unilateral combined valvular insufficiency (e.g., mitral and aortic regurgitation), this technique can calculate only the combined volume of regurgitation. In cases of bilateral valvular regurgitation (e.g., pulmonary and aortic valves), this technique will not provide accurate results. The method, however, may be used to assess the functional consequences of a VSD.
Flow-sensitive or velocity-encoded MRI is the best way to quantify valvular regurgitation. In this method, the velocity of blood flow is linearly related to the brightness of the MR image. Stationary tissue appears gray, whereas antegrade or retrograde flow along the phase-encoding axis appears as black or bright pixels. Velocity-encoded MRI makes it possible to quantify blood-velocity profiles at different time points of the cardiac cycle. Blood velocity can be encoded for imaging planes perpendicular to (through plane velocity measurement) or parallel to the direction of blood flow (in-plane velocity measurement). Flow–volume curves can map the absolute stroke volume and regurgitant volume in different phases of the cardiac cycle.
Compared to nuclear medicine techniques such as single photon emission computed tomography (SPECT) or positron emission tomography (PET), cardiac MRI is capable of visualizing subendocardial or even papillary muscle infarctions owing to its superior spatial resolution.16 Furthermore, cardiac MRI is more cost-effective than PET imaging.
Viable myocardium is myocardium that may recover function following coronary revascularization. Hibernating myocardium (with reduced blood flow) will recover function, but scar/fibrosis in an area of infarction will not. After myocardial infarction, accurate assessment of myocardial viability is crucial for optimal clinical decision making. Cardiac MRI is capable of accurately delineating nonviable or infarcted myocardium from potentially salvageable myocardium. The advantages of cardiac MRI as compared with nuclear, echocardiographic, or PET methods include (1) high spatial resolution, (2) lack of ionizing radiation, and (3) short examination time. Disadvantages include (1) reduced image quality in patients with arrhythmias and (2) patient safety exclusions, as discussed under “Contraindications,” above.
For identifying viable versus nonviable myocardium, an intravenous injection of a gadolinium-based contrast agent is used. On delayed images, obtained 10 to 20 min after contrast injection, myocardial scar (“nonviable”) retains the gadolinium contrast agent, while washout of the contrast agent occurs in viable myocardium (Fig. 57-8). MR images are optimized so that normal myocardium is “suppressed” on GRE images. Another commonly used term for MRI imaging of this type is myocardial delayed enhancement (MDE), or “viability” imaging. This terminology refers to the characteristic 10- to 20-min delay during which the contrast agent in scar/fibrosis distributes differently to normal, viable myocardium.
In viability imaging, scar/fibrosis is depicted as an area of high signal intensity that is typically subendocardial or transmural in a coronary artery distribution. Images are readily interpreted at the base, middle, and apex of the heart on short- and long-axis views. The concept that “bright is dead” is that myocardium with high signal on these MR images corresponds to noncontracting scar/fibrosis. The basis for this relates to both animal models following myocardial infarction and human studies that show lack of contraction of the enhanced myocardium following coronary revascularization.17,18
Because of the high spatial resolution of MRI, subendocardial and transmural infarction can be readily identified. In chronic myocardial infarction, myocardial scar may also show thinning of the ventricular wall, particularly in transmural infarction. In addition, thrombus in regions of aneurysm formation may be identified (Fig. 57-9). In general, myocardial scar/fibrosis involving more than 50 percent of myocardial wall thickness by cardiac MRI is unlikely to recover contractile function following coronary revascularization.18
Figure 57-9
Bright-blood (FIESTA) long-axis view demonstrates focal thinning and outpouching of the anterior left ventricular wall (arrows), compatible with aneurysm formation after myocardial infarction.
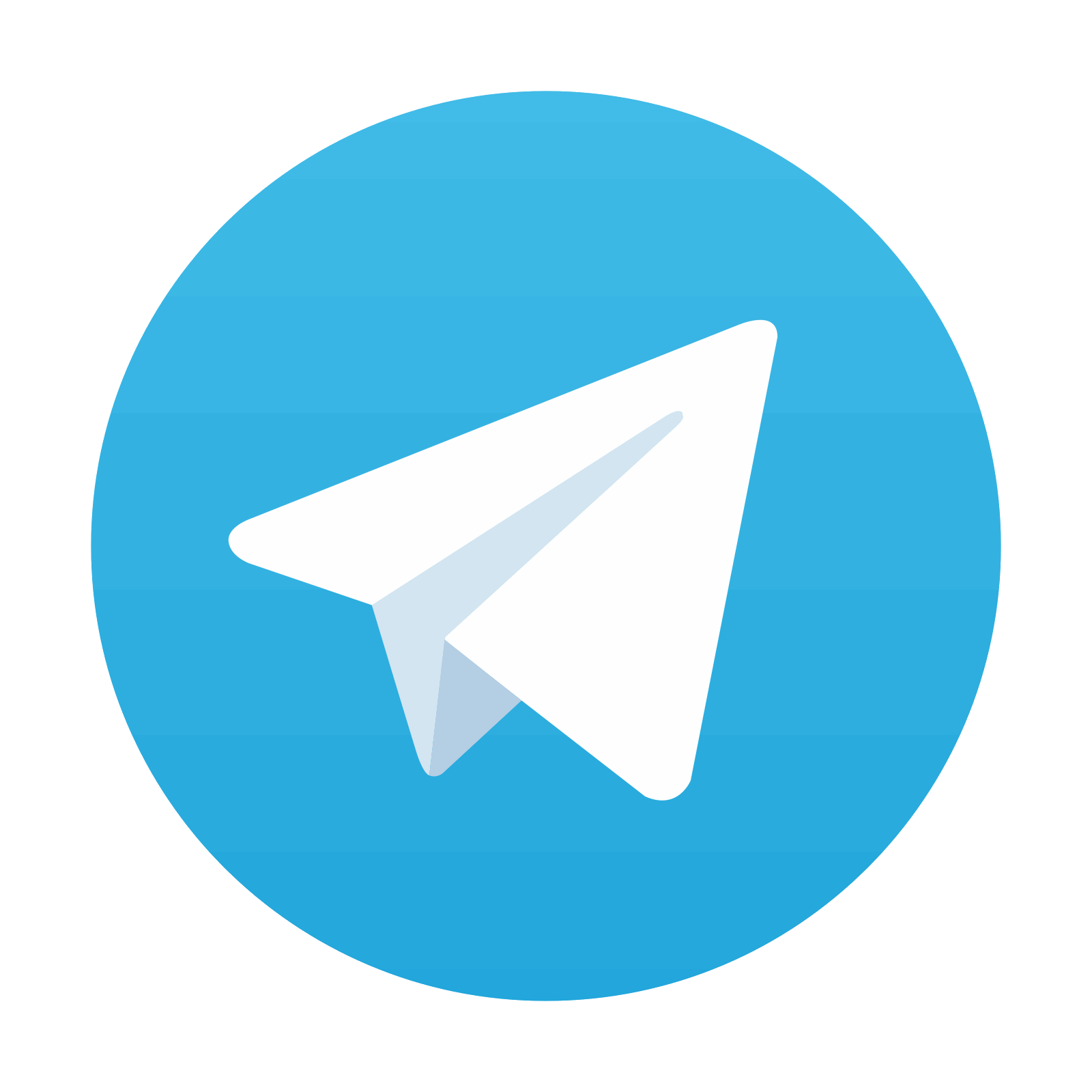
Stay updated, free articles. Join our Telegram channel

Full access? Get Clinical Tree
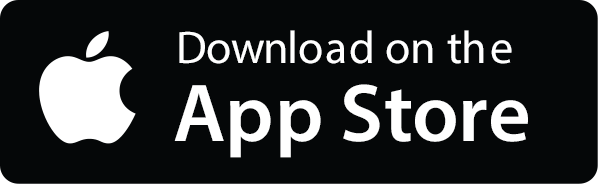
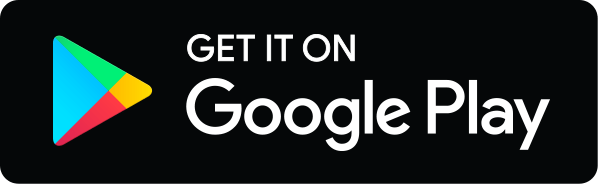
