The most common complications of cardiac catheterization include groin complications (0.43 percent) such as hematomas, retroperitoneal bleeding, pseudoaneurysm, and arteriovenous fistulas. Other complications include contrast reactions, renal failure, perforation, dissection, hypotension, and bradycardia.
When performing coronary angiography, at least two orthogonal views are needed for each vessel to assess the severity of eccentric lesions, and at least five views are recommended to adequately evaluate the left coronary system.
By coronary angiography, a lesion is considered to be severe if there is a greater than 70 percent narrowing of the luminal diameter.
For intermediate lesions of uncertain significance on coronary angiography, intravascular ultrasound (IVUS) and fractional flow reserve (FFR) can be useful. IVUS delineates and quantifies the atherosclerotic burden and consistently demonstrates that lesions are underestimated using angiography alone. FFR is probably the best test to determine both physiologic ischemia (i.e., disease severity) and the need for revascularization.
Balloon angioplasty alone is associated with a 5 percent risk of abrupt closure and a 50 percent risk of angiographic restenosis within 6 months. Bare metal intracoronary stents decrease restenosis by nearly 50 percent compared to balloon angioplasty with a 20 to 30 percent risk of in-stent angiographic restenosis after 6 months.
Drug-eluting intracoronary stents are associated with lower in-stent restenosis rates as low as 5 percent at 12 months, but also carry a higher risk of late and very late stent thrombosis. Patients receiving drug-eluting stents (DESs) must take aspirin for life and clopidogrel for at least 12 months after implantation to mitigate the risk of stent thrombosis.
Cardiac output measurements can be obtained during right-heart catheterization by the Fick and/or thermodilution method. The thermodilution method is more accurate at high-output states, whereas the Fick method is more accurate with low-output conditions and atrial fibrillation.
Aortic valve gradients can be estimated by several methods: (1) Peak-to-peak gradient, (2) “pull-back” method, and (3) simultaneous pressure measurements in the left ventricle (LV) and aorta using a double-lumen catheter (most accurate). The modified Hakki equation can be used to measure the aortic valve area: aortic valve area = cardiac output/square root of the peak-to-peak pressure gradient.
Balloon aortic valvuloplasty (BAV) rarely increases aortic valve area greater than 1.0 cm2 and, although it improves symptoms, clinical deterioration frequently occurs within 6 to 12 months.
Percutaneous patent foramen ovale (PFO) closure devices are associated with high procedural success and low complication rates. Complication rates are around 5 percent and include device migration, pericardial tamponade, and arrhythmias.
The first coronary angiogram was performed by Dr. F. Mason Sones, Jr. at the Cleveland Clinic in 1958, and he published the results of approximately 1000 procedures in 1962.1 With the development of improved catheter designs by pioneers such as Drs. Kurt Amplatz and Melvin Judkins, the number of diagnostic cardiac catheterizations performed annually began to expand exponentially. The rapid growth of cardiac catheterization coincided with Dr. Rene Favaloro’s introduction of coronary artery bypass grafting (CABG) in 1968.1 Presently, almost 2 million cardiac catheterizations are performed each year in the United States.1
The most common indication for cardiac catheterization is the diagnosis of coronary artery disease (CAD). Accepted indications for cardiac catheterization include the evaluation for known or suspected CAD, chest pain, myocardial infarction (MI), wide complex tachycardia, cardiac arrest, shortness of breath, cardiomyopathy, valvular disease, congenital heart disease, aortic dissection, pericardial disease, and heart transplantation.1 Coronary arteriography determines the severity of obstructive CAD and identifies other etiologies of ischemia and infarction, including thrombosis, spasm, dissection, and myocardial bridges.1
Cardiac catheterization plays an integral role in the evaluation and management of patients with cardiomyopathy and heart failure by quantifying the degree of left ventricular (LV) dysfunction with ventriculography and by measuring both right- and left-sided filling pressures. Cardiac catheterization differentiates systolic from diastolic dysfunction, distinguishes pericardial constriction from restriction, and determines the severity of valvular disease. In patients with valvular disease, left-heart catheterization is indicated when there is a disparity between echocardiographic and physical exam findings, and coronary angiography is recommended prior to valve surgery in patients greater than 35 years of age.1
Although there are no absolute contraindications for cardiac catheterization, there are several relative contraindications such as severe contrast allergy, coagulopathy (INR greater than 1.8), renal failure, gastrointestinal bleeding, electrolyte imbalance (hypokalemia and hyperkalemia), acute stroke, infection, fever, severe anemia, uncontrolled hypertension, decompensated heart failure, high-grade heart block, uncontrolled arrhythmias, thyrotoxicosis, and medication toxicity.1
Cardiac catheterization is safe even in critically ill patients, but indications for catheterization should always be based on the risk–benefit ratio.1 Major complications infrequently occur during catheterization. According to an analysis of approximately 60,000 cases, death occurred in only 0.11 percent of patients, and patients with either severe aortic stenosis or critical left main (LM) disease were at the highest risk.1 Incidence of MI, stroke, and arrhythmias is 0.05, 0.07, and 0.38 percent, respectively.1 The most common complications of cardiac catheterization include groin complications (0.43 percent) such as hematomas, retroperitoneal bleeding, pseudoaneurysm, and arteriovenous fistulas.1 Obesity, anticoagulation, female gender, and advanced age are associated with an increased incidence of vascular complications, especially when performed from a femoral artery access site.1 Other complications of catheterization include contrast reactions, renal failure, perforation, dissection, hypotension, and bradycardia.
Cardiac catheterization is an invasive procedure and should only be performed by well-trained clinicians who understand the basic fundamentals of catheterization. The patient must be NPO after midnight for 6 to 8 h prior to catheterization. It is imperative to establish continuous electrocardiogram (ECG) monitoring and intravenous (IV) access for the administration of sedation, fluids, and medications. Patients with a contrast allergy should be treated with prednisone and Benadryl, and IV fluids are administered to those at risk for contrast-nephropathy to achieve greater than 200 mL/h of urine output. Acetylcysteine (Mucomyst) is also frequently administered prior to angiography in individuals at risk for contrast dye nephrotoxicity, although the efficacy of this drug in preventing this remains unproven. A crash cart and defibrillator must be charged and readily available during the procedure.
After the patient is draped in a sterile fashion and comfortably sedated, percutaneous vascular access is most frequently achieved via cannulation of the right common femoral artery. The femoral artery crosses the inguinal ligament, which is located beneath the inguinal skin fold, and divides the ligament into two-thirds lateral and one-third medial.2 Needle entry should be 1 to 3 cm below the inguinal ligament, and the inguinal skin crease should not be used as a landmark as it can be misleading, especially in obese patients.3 After palpation of the femoral pulse, a hemostatic clamp is placed over the presumed access site and fluoroscopy should be performed to ensure that the puncture site will be 1 cm lateral to the medial edge of the middle of the femoral head in the anteroposterior projection (Fig. 55-1). This increases probability of an infrainguinal puncture and cannulation of the common femoral artery. A more cranial puncture increases risk of retroperitoneal bleeding, while cannulation below the bifurcation into the superficial femoral artery is associated with an increased likelihood of a pseudoaneurysm.1 Next, local anesthesia using 1 percent lidocaine should be administered with a 25-gauge needle superficially and then a 22-gauge needle into the deep tissue planes. An 18-gauge beveled needle is introduced into the artery at a 30° to 45° angle resulting in pulsatile bleeding, and slight and careful manipulation of the needle (shallower angle) might be required to improve or restore flow. Single anterior wall puncture is preferred over the Seldinger double-wall technique, and many operators use a micropuncture kit to minimize groin complications.2 After cannulation of the femoral artery, a soft J-tipped 0.035-in guide wire is gently advanced through the needle and into the artery without resistance. If there is any resistance, the wire should be removed, and the needle should be repositioned if arterial flow is compromised. Hemostasis should be maintained for at least 2 to 3 min if the needle is withdrawn from the artery. Once the guide wire is easily passed through the needle, fluoroscopy is performed confirming wire placement in the abdominal aorta, which is left of the anatomic spine. At this point, the needle is removed, and typically a 5F or 6F sheath is inserted over the wire to ensure constant arterial access.
Figure 55-1
A. The fluoroscopic landmark for access in the anteroposterior projection is approximately 1 cm lateral to the medial edge of the middle of the femoral head, which is marked by a hemostat. B. An iliofemoral angiogram in the right anterior oblique projection shows the sheath entering the right common femoral artery.

The most widely accepted technique for left-heart catheterization and coronary angiography is the Judkins method, which is associated with enhanced efficiency and low complication rates.3 The LM coronary artery is usually engaged with the Judkins Left 4 (JL 4) catheter, while the Judkins Right 4 (JR 4) catheter is commonly selected for the right coronary artery (RCA). Engagement of the ostia is performed in the left anterior oblique (LAO) projection. In patients greater than 6 ft tall or in those with dilated aortic roots, a JL 5 or JL 6 may be necessary whereas a small aortic root might need a JL 3.5. Amplatz catheters are helpful when the ostia of the LM or RCA is more superior, anterior, or posterior than the typical aorta, but these catheters increase the risk of ostial dissection secondary to deep engagement.3
After coronary angiography, a pigtail catheter is introduced into the LV, and LV systolic and end-diastolic pressures are obtained. If the LV end-diastolic pressure is less than 30 mm Hg, left ventriculography is then performed in the 30° right anterior oblique (RAO) projection for a single-plane projection or 30° RAO and 60° LAO for biplane projection, using the pigtail catheter and a power injection of 30 mL of contrast media at 10 mL/s. A greater volume of contrast up to 60 mL or even 72 mL can be used if the LV is dilated or in the setting of chronic mitral regurgitation. The pigtail is then pulled back across the aortic valve while pressure is recorded on the hemodynamic monitoring system, and an aortic gradient is measured to assess for aortic stenosis. During catheterization, the patient’s hemodynamics, oxygenation, ventilation, and level of consciousness must be continuously monitored. Once the catheterization is completed, the vascular sheath is removed, and hemostasis is achieved with manual compression for at least 10 to 15 min. Bed rest is mandatory for at least 3 to 4 h, and the patient must keep his or her leg straight to prevent hematoma.
Several vascular closure devices such as suture closure (Perclose, Abbott Laboratories, Abbott Park, Illinois), collagen plugs (Angio-Seal, St. Jude Medical, St. Paul, Minnesota), metallic clips, and hemostatic patches are available.3 Closure devices minimize compression time, allow for early sheath removal in patients who have received anticoagulation, and enable patients to ambulate within 1 to 2 h following the procedure.3 Prior to deployment, an iliofemoral angiogram must be performed in an oblique view to verify sheath entry above the femoral bifurcation (Fig. 55-1). Closure devices should not be used in patients with peripheral arterial disease, compromised immunity, or before valve surgery. A closure device is also discouraged if the vessel is less than 4 mm in diameter.1 Although vascular access complications are probably not decreased with vascular closure devices they facilitate earlier ambulation and improve patient comfort. Use of vascular closure devices should be guided by proper patient selection and single anterior wall puncture.4
The femoral artery is the most common site of arterial access for cardiac catheterization, but brachial and radial arteries can also be used. Morbid obesity, severe debilitating back pain, peripheral claudication, previous femoral arterial grafting surgery, excessively tortuous aortic or iliac disease, femoral bruits, and absent lower extremity pulses are indications for selecting an alternative site for vascular access.2 Radial artery access is more widely utilized outside of the United States for cardiac catheterization, but in light of studies demonstrating lower associated access site bleeding complications with radial compared to femoral access, there is increased interest in employing radial access in the United States.
Sones described the first cardiac catheterization using a brachial artery cutdown, but this technique is infrequently used today.3 Rather, a percutaneous brachial artery technique is preferred without the need for cut down or repair.3 The brachial artery should be cannulated several centimeters proximal to the elbow crease, because 10 percent of patients have a brachial bifurcation before the crease.1 After a 4F to 6F sheath is introduced into the brachial artery, IV heparin should be given to prevent thrombus formation. Nitroglycerin is also recommended, because the brachial artery has the propensity for spasm. Advantages of using brachial over femoral arterial access include quicker time to ambulation and improved patient comfort.3 Although it is easier to achieve hemostasis via the brachial approach, complication rates between brachial and femoral arterial access are indistinguishable.3 Expanding hematomas and compression syndrome (forearm and hand) are more common after brachial access, because there is less space in the arm.2 The brachial approach compromises image quality, increases radiation exposure, and impairs procedural efficiency as catheter manipulation can be quite challenging.3 As a result, this technique should be reserved for patients who are not candidates for either femoral or radial access.2
Radial artery cannulation was first described by Campeau in 1989 and emerged as an alternative to the brachial approach in an effort to minimize vascular complications.3,5 It has become increasingly popular to perform catheterization via the right radial artery secondary to markedly reduced time to ambulation and easier vessel compressibility, which controls bleeding.1,2 In fact, patients may sit up and ambulate immediately after the procedure, facilitating outpatient cardiac catheterization.3 Prior to cannulating the right radial artery, an Allen’s test must be performed to verify dual circulation to the palmar arch. The left radial artery is frequently used as access for catheterization in the presence of a left internal mammary arterial (LIMA) graft. After the usual sterile prep and drape, the right radial site is locally anesthetized, and a 5F or 6F sheath is inserted into the radial artery using a micropuncture kit. Unfractionated heparin, intra-arterial nitroglycerin, and a nondihydropyridine calcium channel blocker should be administered to prevent thrombosis and vasospasm.
A recent meta-analysis of 12 randomized trials, including 3224 patients, showed that radial artery cannulation markedly decreased vascular complications, but it required greater technical skills and had slightly lower success rates.5 Catheter manipulation from the wrist is more challenging than from the groin.1 Other limitations of this approach include the small size of the radial artery, its increased likelihood for vasospasm, and the risk of radial artery occlusion from clot or dissection.3 The radial approach increases the operator’s exposure to radiation as the operator stands closer to the radiation source.1 Radial artery access limits both guide selection and size if a complex percutaneous intervention is warranted, and an additional access site is often required if a right-heart catheterization is needed.3 However, right-heart catheterization can be performed from the forearm with conversion of a peripheral IV catheter to a 6F sheath.
It is essential that the entire catheterization team be cognizant of the risks of radiation. Cumulative doses of radiation can result in skin erythema, hair loss, and necrosis, and these injuries occur in a dose dependent manner after a certain limit is exceeded (deterministic effects).1,3 Radiation can also cause genetic defects and malignancies, and these feared complications of radiation are not dose dependent; rather, the probability of an effect is increased with radiation use (stochastic effects).1,3 All catheterization personnel must understand the basic principles of minimizing radiation exposure. Radiation exposure is limited by the use of lead aprons, thyroid shields, and lead glasses. The catheterization table must have a lead shield to protect the operator’s lower body and an adjustable glass lead shield for his or her upper torso and head. Radiation is also limited by reducing fluoroscopic and especially cineangiographic time, minimizing image magnification, and through the use of collimators (decrease field size) and less angled views.6 Projections performed in the lateral, LAO, and steeply angulated caudal or cranial view increase radiation exposure to the patient and operator and are also associated with reduced image quality.1 Minimizing distance between the patient and x-ray source reduces radiation exposure, and doubling the distance between the x-ray entrance and the operator decreases radiation dose to the operator by as much as 75 percent.1 Catheterization personnel must wear radiation badges at all times; the maximum amount of whole body radiation dose per year and per lifetime is 5 and 50 rems, respectively.3
The LM coronary artery arises from the left sinus of Valsava usually at an acute angle, and it travels between the left atrium and the pulmonary artery and is partially covered by the left atrial appendage.7 The LM gives rise to the left anterior descending (LAD) artery, which supplies the anterior wall of the LV, and the left circumflex (LCx) artery, which supplies the lateral wall. In one-third of patients, the LM trifurcates into the LAD, ramus intermedius artery, and the LCx.7 The ramus intermedius has a similar course as the first diagonal branch of the LAD and the first or high obtuse marginal branch of the LCx and provides blood supply to the high lateral wall of the LV. The LAD gives off several diagonal and septal perforator branches as it courses along the anterior interventricular groove before either tapering into a small caliber vessel ending at the cardiac apex or wrapping around the apex to supply the distal one-third of the inferior wall (Fig. 55-2). The septal perforators supply two-thirds of the anterior portion of the interventricular septum and the majority of the conduction system including the His bundle.8 The LAD diagonal branches supply the anterolateral aspect of the LV.7
The LAD takes an intramyocardial course in approximately 12 percent of patients,7 and myocardial bridges almost always involve the mid-LAD but can infrequently affect a septal branch, the posterior descending artery (PDA), or an obtuse marginal branch. Bridging is easier to appreciate angiographically after administration of nitroglycerin.1 Systolic narrowing of coronary arteries rarely causes ischemia as the majority of coronary flow occurs during diastole. Although myocardial bridging is benign in the majority of cases, it can result in critical luminal compression during systole in 2 percent of patients and also impair early diastolic coronary flow.9 Myocardial bridging can make bypass surgery more challenging, especially during minimally invasive approaches.1
As the LCx courses along the left atrioventricular (AV) sulcus, it gives rise to small-caliber left atrial branches and several obtuse marginal branches, which supply the lateral wallbefore terminating in the AV groove (Fig. 55-3). The first obtuse marginal branch is frequently called the high lateral branch, and subsequent marginal branches may be referred to as left posterolateral branches.
The RCA originates from the right aortic sinus and supplies the inferior wall of the LV and right ventricle (RV). The first branch arising from the RCA is the conus branch. The proximal RCA then gives origin to small caliber right atrial branches and several acute marginal branches that supply the free wall of the RV.7 In 85 percent of patients, the RCA is dominant and the distal RCA crosses the crux and bifurcates into the right posterior descending artery (RPDA) and right posterolateral ventricular (RPLV) branches distally (Fig. 55-4). The origin of the PDA defines coronary dominance as it determines the source of blood supply to the inferior wall of the LV, and it can arise from a dominant LCx in a minority of patients.9 The length of the RCA along the inferior wall of the heart tends to be inversely related to the length of the LCx.7 The inferior wall of the heart is supplied by both the RCA and the LCx in 20 percent of patients, and this is referred to as a mixed, shared, or codominant system.7 The conus branch supplies the RV outflow tract and arises 50 percent of the time from a separate ostium in close proximity and anterior to the origin of the RCA.1,9 Although the atrial branches of the RCA provide blood supply to the sinus node 60 percent of the time, a minority of patients depend on an atrial branch of the LCx.9 In approximately 90 percent of patients, the AV node receives blood supply from the AV nodal branch off of the distal RCA.1
The PDA courses along the posterior interventricular groove to supply the posterior and inferior free walls of the LV. The PDA gives off several small septal branches which supply the inferior portion of the interventricular septum, the posteromedial mitral papillary muscle, as well as the right and a portion of the left bundle branch.7,8 The posteromedial muscle of the mitral valve is supplied by the PDA while the LAD and LCx provide dual supplies to the anterolateral mitral papillary muscle. As a result, an inferior wall MI involving the RCA or a dominant LCx is the most common cause of acute ischemic mitral regurgitation.
Coronary angiography was performed in 126,595 patients, and an anomalous coronary artery was present in 1.3 percent.10 The most common coronary anomaly is the LAD and LCx arising from separate ostia (30.4 percent), and the second most common anomaly is the LCx originating from the right sinus or the proximal RCA (27.7 percent).10 Although 81 percent of coronary anomalies are benign, there are several potentially serious anomalies such as the LM arising from the pulmonary artery (Bland–White–Garland Syndrome) and the LM originating from the right aortic sinus and taking an interarterial course (between the pulmonary artery and aorta).1 This variant is considered benign if the LM arises from the right sinus and takes a septal, anterior, or retroaortic course. Coronary fistulas account for 10 percent of all coronary anomalies, and while small fistulas are relatively benign, patients with a large coronary fistula may present with angina or heart failure.9 Patients with congenital heart disease, such as a bicuspid aortic valve or tetralogy of Fallot, frequently have an anomalous coronary artery.1
Coronary arteries communicate via anastomotic channels, and in patients with normal or minimal CAD, these collaterals are not usually seen due to their small size (50–200 μm in diameter).7,11 However, collaterals gradually develop and enlarge when there is severe obstructive CAD secondary to a pressure gradient generated across the channel.7,11 Collaterals provide approximately 50 percent of coronary flow in occluded vessels and are sufficient to protect the myocardium from infarction and ischemia at rest.11 However, during provocative testing such as exercise stress testing, a collateral-dependent territory will generally show evidence of inducible ischemia.
When performing coronary angiography, at least two orthogonal views are needed for each vessel to properly assess the severity of eccentric lesions, and at least five views are recommended to adequately evaluate the left coronary system. Traditionally, images were acquired at 30 frames/s, but most labs today obtain images at 15 frames/s. Thirty frames/s is recommended if the patient is tachycardic or has a high-flow state.1 Image quality in some projections, such as LAO cranial, is improved by acquiring images when the patient takes a deep breath, and vessel overlap is decreased by using angulated views.1 Contrast injection by hand should start slowly with a gradual augmentation in force to prevent catheter disengagement, and the injection must be strong enough to cause a reflux of contrast into the sinus of Valsalva, enabling adequate visualization of the ostium.1 Contrast injections should be sufficient to fully opacify the vessel lumen. Hand controlled automated injectors are becoming more popular in catheterization laboratories and facilitate adequate opacification of the coronary arteries especially when using 4F or 5F catheters. Extreme caution is required to avoid injecting air into the coronary circulation.
The LM is usually approached in the 30° LAO projection with a JL 4 catheter. Slight counterclockwise rotation might be warranted to move the catheter in an anterior direction to properly engage the LM. Conversely, clockwise rotation may be required to move the catheter in a posterior direction if it is not coaxial with the LM.1 Clockwise rotation of a JL 5 or even JL 6 catheter may be required to engage the LM in the setting of a dilated aorta.1 Forceful injection of contrast into the LM might result in dissection if there is damping or if the catheter is oriented upward against the roof of the LM and not coaxial with the LM.1 Although the JL 4 normally engages the LM without manipulation, an Amplatz catheter might be necessary if the LM is anomalous or has an unusual orientation. Amplatz catheters are associated with a higher risk of catheter induced coronary dissection.1
Severe LM disease is extremely dangerous and suggested by damping or ventricularization of the pressure wave form upon catheter engagement. The best views to visualize the ostial LM are either the shallow LAO or the LAO cranial projections. A distal LM stenosis is best seen in an AP caudal or shallow RAO caudal view. RAO caudal and LAO cranial projections should be used to view the proximal LAD, and the AP, LAO, and RAO cranial views are recommended to visualize the mid and distal LAD as well as the diagonal branches (Fig. 55-2). Although the lateral view increases radiation exposure to the operator, it is helpful in evaluating mid LAD lesions. The LCx and obtuse marginal branches are best seen in the AP caudal and shallow RAO and LAO caudal projections (Fig. 55-3). The LAO caudal view is recommended for visualizing the origin of the ramus intermedius and the LAO cranial projection is the best for evaluating a left PDA.
The RCA is engaged in the 30° LAO projection with a JR 4 catheter. After advancing the JR 4 catheter to the aortic valve, the catheter is pulled back approximately 2 to 3 cm and turned clockwise in an anterior direction. The proximal and mid-RCA are best visualized in the LAO view (Fig. 55-4A). The 30° cranial AP projection is used for evaluating the distal RCA bifurcation and the origin of the RPDA and RPLV branches, and the midsegment of the RPDA is best seen using a shallow RAO view (Fig. 55-4B). The right lateral projection can help visualize the ostial and mid segments of the RCA and the origin of the acute marginal branches.
Coronary artery bypass grafts should be visualized in the same projections recommended for visualization of the native target vessels. The distal anastomosis of the bypass graft and the coronary artery is a frequent site of disease and must be evaluated carefully. Bypass grafts to the RCA should be engaged in the LAO projection and are usually more anterior and more caudal than grafts to the left coronaries. Left coronary bypass grafts should be engaged in the RAO view. Frequently, the sequence of the ostia of venous grafts in a cranial to a caudal direction is the second obtuse marginal branch, first obtuse marginal, ramus intermedius, diagonal, and then the LAD.1 Most saphenous vein grafts can be engaged with a JR 4 catheter and, if the graft is occluded, an injection is performed to verify the stump. An aortogram in the 30° LAO projection using approximately 40 to 50 mL of contrast might be necessary to locate grafts.1
Caution should be taken when engaging the RIMA and LIMA grafts due to the risk of dissection. The brachiocephalic or left subclavian artery should be cannulated by a JR 4 catheter, and then an exchange length J-tipped guide wire should be passed carefully into the respective distal subclavian artery. Catheters should not be advanced into the right or left subclavian artery unless over a guide wire. After the JR 4 catheter is removed, the IMA catheter is advanced slowly over the exchange guide wire. The IMA catheter is then slowly pulled back and torqued in the appropriate direction to engage the RIMA and LIMA grafts. The LIMA is generally engaged by pulling the IMA catheter back and by turning it counterclockwise or in an anterior direction. The catheter should be maintained in a coaxial orientation with the IMA during contrast injection to avoid causing a catheter-induced dissection.
The magnitude of the impact of CAD is extensive. Ischemic heart disease is the number one cause of death both in the United States and worldwide, and its incidence and prevalence continue to increase secondary to an aging population and an increase in obesity and diabetes.12 Approximately 13 million Americans are affected by CAD, and slightly more than 50 percent of these patients have suffered from a MI.12 The economic burden of CAD exceeded US$142.5 billion in the United States in 2006. After the age of 40, the lifetime risk for developing ischemic heart disease is 53 percent for men and 32 percent for women.12
The most common etiology of MI is plaque rupture and subsequent thrombus formation, but angina usually results from supply and demand mismatch. Supply is a function of coronary flow and arterial oxygen saturation, and demand is affected by heart rate, contractility, and LV wall stress. Classically, angina presents as substernal or left-sided crushing or squeezing chest pressure with radiation to the left arm and is frequently associated with dyspnea, diaphoresis, nausea, and vomiting. Angina is usually precipitated by exertion and relieved by rest and nitroglycerin. Ischemia can also manifest as dyspnea on exertion secondary to elevated left-sided filling pressures.
The initial evaluation of patients with chest pain includes a thorough history and physical exam, serum markers of myocardial necrosis, and a resting ECG. The resting ECG is normal in approximately 50 percent of patients with angina. Although cardiac enzymes such as troponin and creatine kinase rise within 3 h of myocardial injury and provide significant prognostic information, these biomarkers are usually normal in patients with angina.12 Although a patient with ischemic ECG changes and/or elevated cardiac enzymes should be referred for cardiac catheterization, noninvasive stress testing is recommended in patients with an intermediate pretest probability of having CAD.12 Exercise electrocardiography has a sensitivity and specificity of 68 and 77 percent, respectively.12 The test is limited by the patient’s ability to achieve their target heart rate and the requirement of a normal ECG at baseline. In patients who are unable to exercise and in those with abnormal resting ECGs, myocardial imaging is recommended, which markedly increases the prognostic power of the test. Myocardial perfusion imaging using adenosine has a sensitivity of 90 percent and a specificity of 82 percent.12 Adenosine is very safe but contraindicated in patients with severe bronchospasm, high-grade heart block, and in those taking dipyridamole. Stress echocardiography using dobutamine has a sensitivity of 81 percent and a specificity of 79 percent, but its use is limited in patients with poor echocardiographic windows, ventricular arrhythmias, and left bundle branch block.12
Although noninvasive testing plays an integral role in diagnosing CAD, coronary angiography remains the gold standard. By coronary angiography, a lesion is considered to be severe if there is greater than a 70 percent narrowing of the luminal diameter, and a mild stenosis is defined as less than a 30 percent blockage. Results of coronary angiography in patients with stable angina are single-vessel disease in 25 percent, double-vessel disease in 25 percent, triple-vessel disease in 25 percent, significant LM disease (>50 percent stenosis) in 10 percent and 15 percent have nonobstructive CAD.12 For intermediate lesions of uncertain significance, other techniques such intravascular ultrasound (IVUS) and fractional flow reserve (FFR) can be used to assess disease severity and to guide therapy. IVUS delineates and quantifies the atherosclerotic burden and consistently demonstrates that lesions are underestimated using angiography alone.12 Although minimal luminal area and area stenosis as measured by IVUS provide additional information to angiography, FFR is probably the better test for determining both physiological ischemia (disease severity) and the need for revascularization.13,14 However, IVUS might be preferable for evaluating LM disease and transplant vasculopathy.15 FFR is performed by crossing the lesion of interest with a 0.014-in pressure wire and, after the administration of adenosine, the pressures distal and proximal to the stenosis are obtained. A ratio of distal pressure to proximal pressure of less than 0.75 to 0.80 is deemed abnormal. The sensitivity of FFR for detecting reversible ischemia is 88 percent, and the specificity is 100 percent.14
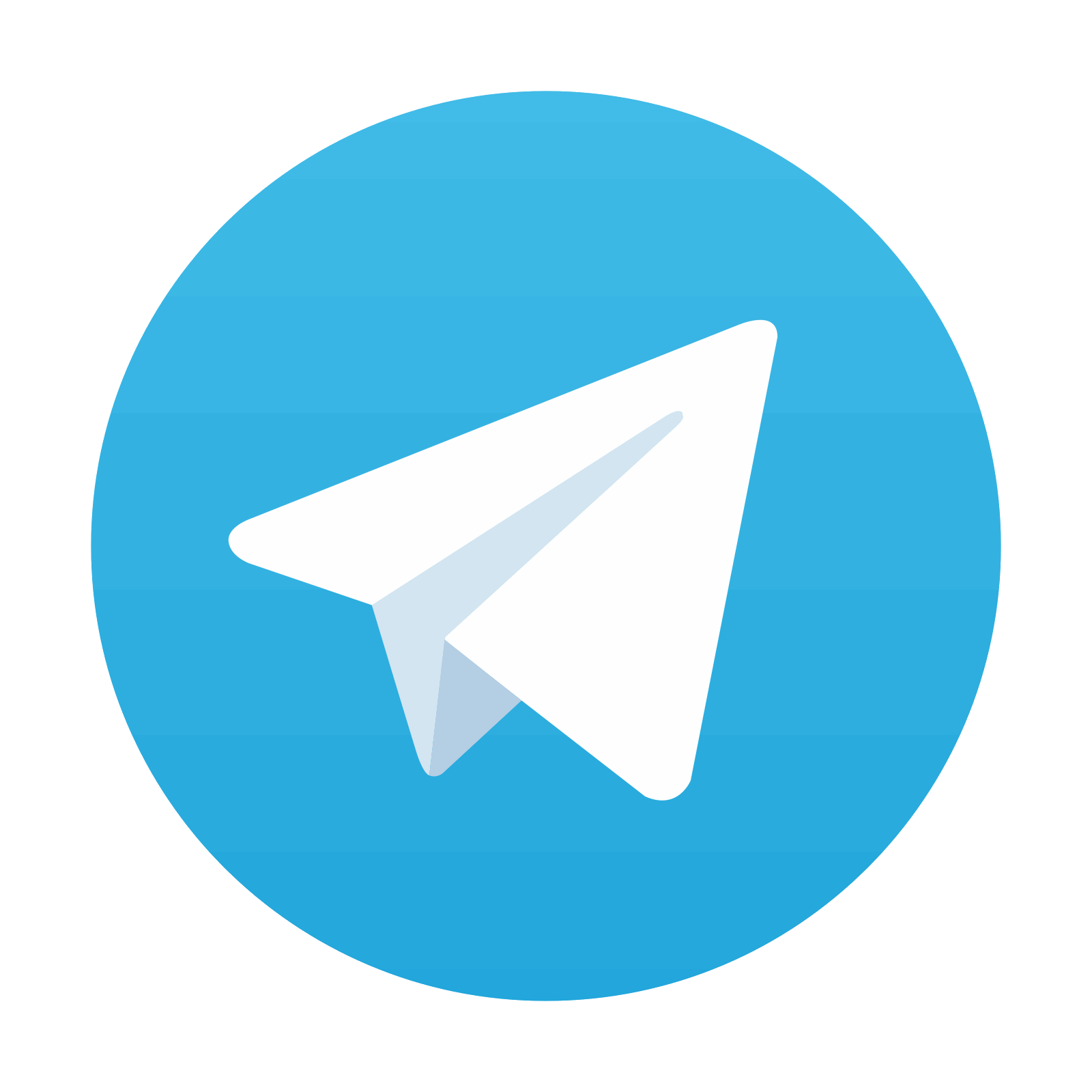
Stay updated, free articles. Join our Telegram channel

Full access? Get Clinical Tree
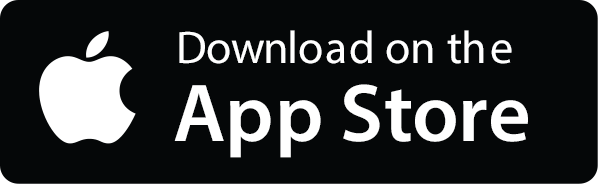
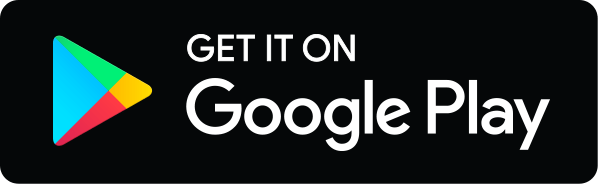