Outline
Introduction 299
Do Adult Bone Marrow-derived Stem Cells Participate in Kidney Repair? 300
Bone Marrow Mesenchymal Stem Cell Therapy Contributes to Renal Repair 300
Mesenchymal Stem Cells 300
Functional Effect of Bone Marrow Mesenchymal Stem Cells in Acute Kidney Injury 302
Paracrine Effect of Bone Marrow Mesenchymal Stem Cells 303
Strategies to Enhance Stem Cell Effects 304
Signals, Receptors and Trafficking of Bone Marrow Mesenchymal Stem Cells 304
Strategies to Increase Bone Marrow Mesenchymal Stem Cell Migration 307
Strategies to Enhance Bone Marrow Mesenchymal Stem Cell Survival and Efficacy 308
Conclusion 309
Acknowledgments 309
Stem cell-based therapies may represent promising therapeutic strategies for several diseases. To date, the safety and effectiveness of ex vivo expanded bone marrow-derived mesenchymal stem cell (BMMSC) therapies have been documented in several preclinical and clinical studies; however, the mechanisms of action during migration, engraftment, survival and paracrine factor release of BMMSCs during tissue repair are still not clearly defined. This chapter present a discussion of the main key players of tissue repair processes that could be modulated to enhance the therapeutic benefits of stem cells.
Introduction
Bone marrow-derived mesenchymal stem cells (BMMSCs) are currently considered one of the cell types with great clinical potential for cell-based therapy . When transplanted systemically, mesenchymal stem cells (MSCs) are able to migrate to sites of injury and exert their therapeutic action via paracrine release of trophic factors instead of a direct repopulation of the damaged tissue. Experimental studies have reported the use of BMMSCs as preferred stem cells for therapy of acutely damaged heart , lung and liver . Some experimental studies using BMMSCs have been translated into clinical trials, with results that are sometimes modest and controversial . Even in the kidney, stem cell therapy has been approached to support the limited renal capacity to recover following acute ischemic or toxic injury. Acute kidney injury (AKI) is a potentially devastating disorder with an extremely serious outcome. Several pharmacological therapies for AKI have been attempted, with disappointing results . BMMSC therapy has been identified as a powerful treatment to regenerate damaged renal tissue in experimental models of AKI . To maximize treatment efficacy and translate these results to patients with highly compromised renal function, we need to understand how to enhance the efficiency of MSCs in terms of migration, engraftment, production of cytokines and growth factors, and differentiation in the pathological environment of kidney failure. These issues will be addressed in this chapter.
Do Adult Bone Marrow-derived Stem Cells Participate in Kidney Repair?
Pioneering preclinical studies have reported the existence of a bone marrow–kidney axis with bone marrow cells contributing to tubular epithelial cells physiological processes of cell turnover or regeneration and to mesangial cells, podocytes and endothelial cells . In mice, Poulsom et al. showed the presence of Y-chromosome-positive cells in kidneys obtained from female recipients of male bone marrow , demonstrating the existence of traffic of these cells into the kidney. Moreover, the percentage of Y-chromosome-positive tubular epithelial cells ( Lens culinaris lectins positive), which ranged from 0.6 to 6.8% , suggested a contribution of bone marrow cells to the renal epithelium. Although the presence of male tubular cells after acute renal injury was a rare event, several reports showed that extrarenal cells could directly participate in the regeneration of tubules. In acute tubular necrosis, male patients receiving a kidney transplant from female donors showed Y-chromosome-positive cells in tubular epithelium and in glomeruli, where they expressed vimentin, suggesting a podocyte phenotype . Similarly, Gupta et al., studying the origin of tubular cells in male patients with resolving acute tubular necrosis who had received a kidney transplant from a female donor, documented 1% of the tubular cells positive for the Y-chromosome and negative for the hematopoietic marker CD45 in the kidneys after injury . Another study in gender-mismatched transplantation, with male patients receiving a female renal allograft, demonstrated that stable tubular epithelial chimerism (from 2.4 to 6.6%) occurred regularly in renal allografts, in 72% of the patients . However, this study does not clarify whether the Y-chromosome-positive cells found in the transplanted kidney came directly from the bone marrow, represented circulating stem cells or derived from other organs. After acute tubular injury induced by folic acid treatment in mice, bone marrow-derived cells recruited to the kidney proliferated and generated tubular cells and glomerular mesangial cells . Little evidence for cell fusion between indigenous renal tubular cells and bone marrow-derived cells was found . Others have obtained conflicting results . Recently, enhanced green fluorescent protein (eGFP)-labeled bone marrow-derived stem cells (BMSCs) were transplanted in sublethally irradiated syngeneic recipient mice. Four weeks after transplantation, ischemia–reperfusion injury was induced. On day 7 postdamage eGFP-positive BMSCs were clearly detectable in ischemic kidney tissue and contributed towards 10% of regenerated proximal tubular cells . None of these reports discriminated whether cells from bone marrow which home to the kidney were hematopoietic stem cells (HSCs) or MSCs.
Studies on bone marrow-derived HSC mobilization with a cocktail of cytokines showed conflicting results with potentially negative effects on kidney regeneration , whereas others exhibited renoprotection in an AKI model induced by folic acid . In addition, transplantation studies with GFP-positive hematopoietic lineage cells or male cloned cultured BMMSCs into irradiated female mice have shown that both populations can engraft the bone marrow; however, only hematopoietic cells could transdifferentiate into renal tubular epithelial cells, playing a role not only in normal turnover but also in the repair of HgCl 2 -induced tubular injury . In different animal models bone marrow-derived cells were able to differentiate into podocytes, mesangial and endothelial cells . However, the contribution of extrarenal cells to kidney repair remains controversial, with studies indicating that repair of injured nephrons is mainly accomplished by intrinsic, surviving tubular epithelial cells . In chimeric mice expressing GFP or β-gal in bone marrow-derived cells, subjected to ischemic injury, some interstitial cells (mainly leukocytes) but not tubular cells expressed GFP, whereas β-gal-positive signals were attributed only to endogenous cells , excluding a direct contribution of bone marrow-derived cells to renal repair .
Bone Marrow Mesenchymal Stem Cell Therapy Contributes to Renal Repair
Mesenchymal Stem Cells
MSCs are currently considered one of the cell types with good clinical potential for cell-based therapy because of their relative ease of expansion, immunoprivileged and immunosuppressive character, great tropism for damaged organs and the spectrum of growth factors which regulate crucial cellular biological function at the place of engraftment . MSCs, also defined as stromal cells, represent a heterogeneous cell population of multipotent stem cells that mostly resides in the bone marrow. It has been estimated that MSCs represent 0.01–0.0001% of the nucleated cells of adult human bone marrow. These cells can differentiate into the mesodermal cell lineage as adipocytes, osteocytes and chondrocytes, but they also have endodermic and neuroectodermic potential . However, the evidence that BMMSCs can transdifferentiate into functional tissue-specific cell types both in vitro and in vivo to promote tissue repair is still controversial. To understand more about BMMSC biology, the key questions to address are: where are they anatomically located, and what is their natural function? In the bone marrow of the trabecular bone, mesenchymal/stromal progenitor cells at different stages of maturation are functional components of the HSC niche. In the endosteal niche, stromal progenitor cells work together with osteoblasts in maintaining HSCs in a quiescent state, while in the vascular niche perivascular stromal cells and sinusoidal endothelial cells regulate the proliferation, differentiation and recruitment of HSC to the systemic circulation via local release of specific cytokines. In vitro data document that human BMMSCs are able to release several factors , among them stem cell factor (SCF), leukemia inhibitory factor (LIF), stromal cell-derived factor-1 (SDF-1), oncostatin M (OSM), bone morphogenetic protein-4 (BMP-4), fms-like tyrosine kinase-3 (Flt-3), transforming growth factor-β (TGF-β) ( Table 20.1 ) , supporting self-renewal rather than differentiation of immature HSCs in the bone marrow. Moreover several interleukins ( Table 20.1 ) [28,35], act on committed hematopoietic cells toward blood cell lineages. Recent studies indicate a new niche for MSCs, showing the presence of perivascular cells coexpressing markers of pericytes and MSCs in multiple organs including the kidney . These findings may suggest a functional role for MSCs in the control of microvascular stability.
Cytokines and growth factors | Ref. |
---|---|
IL-6, IL-7, IL-8, IL-11, IL-12, IL-14, IL-15 | |
IL-1, IL-10, IL-27 | |
LIF, M-CSF, Flt-3, SCF | |
HGF, PGE 2 | |
TGF-β, MMP-2, TSP-1 | |
PDGF, Ang-1, PA, MMP-9 | |
HO-1 | |
VEGF, MCP-1, IGFBP-2, IGFBP-4 | |
EGF, IGF-1, bFGF, SDF-1, FGF-2 |
The use of these cells for clinical protocols are of interest because BMMSCs can be easily isolated from a small aspirate of bone marrow, rapidly expanded in vitro, maintaining their genetic stability , and collected or dispatched from the laboratory to the bedside. Moreover, cultured BMMSCs have been successfully infused in humans for safety and early clinical testing for the treatment of genetic disorders , and in ischemic cardiomyopathies , hematological diseases , Crohn’s disease and spinal cord injury . There is a general consensus that cultured human BMMSCs express variable levels of CD105 (endoglin), CD73 (ecto-5′-nucleotidase), CD44, CD90 (Thy1), CD71 (transferrin receptor), the ganglioside GD2 and CD27 (low-affinity nerve growth factor receptor), whereas they do not express hematopoietic markers CD45, CD34 and CD14. Owing to the low constitutive expression of major histocompatibility complex (MHC) class II and the absence of costimulatory molecules such as CD80, CD86 and CD40/CD40L, BMMSCs escape the immune system and therefore can be injected into allogeneic host without rejection , in the absence of conditioning regimens. In vivo data obtained in humans , baboons and rodents showed that BMMSCs inhibit T-cell responses to cellular and to non-specific mitogenic stimuli, targeting both naïve and memory CD4 + and CD8 + T cells . There is also evidence that BMMSC-induced T-cell suppression occurs independently of MHC matching with either stimulatory cells or responder lymphocytes in the mixed lymphocyte reaction (MLR) . BMMSC-mediated immunomodulation is promoted by close cellular contact but also by several soluble factors, including hepatocyte growth factor-1 (HGF-1), TGF-β, indoleamine 2,3-dioxygenase (IDO) and prostaglandin E 2 (PGE 2 ) ( Table 20.1 ) . More recently, nitric oxide and insulin-like growth factor (IGF) binding protein have also been shown to play a key role. Immunosuppressive effects of BMMSCs are increased by inflammatory cytokines such as interferon-γ (IFN-γ) and tumor necrosis factor-α (TNF-α) . Scanty and conflicting data are available on the immunogenicity and immunomodulatory effects of BMMSCs in vivo. Allogeneic BMMSC infusion in rats with AKI significantly ameliorated renal dysfunction, although the effect on animal survival was very modest . Intramyocardial injection of BMMSCs from allogeneic donors in pigs 3 days after infarction resulted in long-term engraftment, decreased scar formation and near-normalization of cardiac function . A recent study demonstrated long-term allogeneic BMMSC survival, engraftment and restoration of cardiac function following transplantation into chronically scarred myocardium . Based on their immunosuppressive properties, allogeneic BMMSCs were used as a promising therapeutic approach to enhance skin engraftment in immunocompetent baboons and reduced graft-versus-host disease in mice and humans . In addition, pretransplant infusion of BMMSCs prolonged the survival of semi-allogenic heart transplant through the generation of regulatory T cells in unconditioned recipient mice . At variance, conflicting results showed that BMMSC injection failed to prevent graft rejection and slightly prolonged graft survival in fully MHC-mismatched vascularized heart transplant in rodents.
The great potential of BMMSCs for cell therapy may rest on their ability to migrate to the site of acute tissue damage or inflammation injury, promoting tissue repair. This peculiar property of BMMSCs has been demonstrated in the setting of bone fracture , cerebral ischemia and infarcted heart , as well as in the damaged kidney , where BMMSCs can promote tissue regeneration through the local delivery of mitogenic and vasculotropic factors , as described below.
Functional Effect of Bone Marrow Mesenchymal Stem Cells in Acute Kidney Injury
A number of reports highlights that MSCs of bone marrow origin have a role in regeneration after acute renal failure. The renoprotective effect of BMMSCs was first documented by this group in the experimental model of AKI induced by the nephrotoxic drug cisplatin. Male BMMSCs, transplanted in female mice 1 day after cisplatin injection, restored renal function and tubular structures . The engraftment of BMMSCs into the damaged kidney was investigated by tracking Y-chromosome-positive cells, which were detected, although to a very low extent, in tubuli displaying binding sites for L. culinaris lectins. This study also showed that BMMSCs significantly accelerated proliferation of tubular cells to a remarkable extent, as proven by the presence of Ki-67 signal in the cells. The effect of human BMMSCs was also investigated in immunodeficient non-obese diabetic/severe combined immunodeficiency (NOD/SCID) mice with cisplatin-induced AKI. Renal functional recovery, associated with a therapeutic effect on survival, was observed in these animals accompanied by an increase in cell proliferation, and reduction of apoptosis and tubular lesions . These cells were found predominantly in tubulointerstitial areas ( Fig. 20.1 ), with the rest equally distributed in tubuli and glomeruli . Human BMMSC treatment also induced protection against AKI-related peritubular capillary changes consisting of endothelial cell abnormalities, inflammatory cell infiltration, and low endothelial cell and lumen volume density as assessed by morphometric analysis. The present group has reported even more pronounced positive results by using human MSCs obtained from cord blood, with improvement of renal function and significant prolongation of survival . This renoprotective effect appeared to be mediated by a complex action of human cord blood MSCs on tubular cells involving lowering oxidative stress, apoptosis and inflammation counterbalanced by the activation of prosurvival and mitogenic signaling . In another mouse model of glycerol-induced AKI, mouse BMMSCs differentiated into tubular epithelial cells (intravenously injected GFP-positive BMMSCs were positive to cytokeratins), and promoted epithelium proliferation and renal function . In rats, administered BMMSCs had highly renoprotective capacity and enhanced the recovery from ischemia–reperfusion AKI . Here, iron-dextran-labeled BMMSCs were located in the kidney cortex after injection, as demonstrated by magnetic resonance imaging . In the same rat model, Tögel et al. studied intracarotid administration of fluorescence-labeled BMMSCs and, despite no differentiation occurring, they found kidney protection . Another study by the same authors reported that both autologous and allogeneic BMMSCs were safe and effective in an ischemia–reperfusion model of AKI in rats, and reduced late renal fibrosis by ameliorating renal function in surviving animals .

The effectiveness of BMMSCs in the treatment of acute kidney damage has been here clearly highlighted; however, it is important to know the extent of cell recruitment and engraftment in the target organ, in order to manage patient treatments and for the success of the therapy. Cell delivery methods and the intrinsic capacity of the cells to migrate into the kidney could affect the results and make a difference. Several studies have made use of cell-tracking methodologies to quantify administered stem cells in target tissues, but mainly provided no evidence that BMMSCs enter the renal parenchyma in significant numbers . Tögel et al., using fluorescently labeled MSCs injected in ischemic rats, showed that fluorescent cells were detected only exceptionally at 24 h in scarce numbers in the kidney and in the renal microvasculature for only 1–2 days after infusion . In an ischemia–reperfusion model in rats, iron-dextran-labeled BMMSCs intravenously injected through the carotid artery were found on day 3 at a frequency of 30,000 cells per kidney . Recent data showed that in cisplatin-induced AKI, injected murine BMMSCs predominantly localized in peritubular areas and to a lesser extent in the tubular epithelium, with scattered BMMSCs found in glomeruli . In the same AKI model, 84% of human BMMSCs engrafted peritubular areas, whereas only 8% were found in tubules and 8% in glomeruli ( Fig. 20.1 ) . In these animals, PKH-26-labeled human BMMSCs localized in renal tissues at a frequency of 3.4 ± 0.76 per 10 5 renal cells . In agreement with these findings are data in a model of glycerol-induced AKI showing that injected murine BMMSCs were recruited largely in peritubular areas, but still resulting in kidney recovery . Nevertheless, eGFP-fluorescent BMMSCs injected into mice subjected to ischemia–reperfusion were not found in the kidney parenchyma at 24 h, 48 h, 7 days or 15 days after ischemic injury .
Paracrine Effect of Bone Marrow Mesenchymal Stem Cells
The very low engraftment of infused BMMSCs in the damaged renal tissue and the preferential localization of stem cells to peritubular interstitial areas would exclude a direct repopulation of the tubules by BMMSCs being responsible for the renoprotective effects. The evidence supports paracrine action of BMMSCs once engrafted in the damaged kidney, via the local release of soluble factors with regenerative potential for renal cells ( Fig. 20.2 ). Relevant to the former interpretation are data showing that in the experimental model of ischemia–reperfusion injury in rats, the protective effect on renal function and structure by BMMSCs was due to their intrinsic ability to produce high levels of growth factors such as HGF, vascular endothelial growth factor (VEGF) and IGF-1 . Kidneys of rats, 24 h after BMMSC infusion, showed a significant reduction in the expression of proinflammatory genes coupled with an increased gene expression of basic fibroblast growth factor (bFGF), TGF-β, and of the antiapoptotic and anti-inflammatory B-cell lymphoma-2 (Bcl-2) and interleukin-10 (IL-10) compared with kidneys of untreated animals. More recently, the administration of conditioned medium derived from murine BMMSCs to cisplatin mice with AKI ameliorated renal dysfunction, enhanced tubular regeneration and limited cell apoptosis . That IGF-1, a mitogenic and prosurvival factor, could represent a powerful regenerative agent in MSC-mediated renal repair rests on data showing that administration of IGF-1 gene-silenced BMMSCs in mice with cisplatin-induced AKI limited their protective effect on renal function and tubular injury . Similarly, VEGF knocked down by small interfering RNA reduced the effectiveness of BMMSCs and decreased animal survival . It has also been shown that suppression of HGF production impairs the ability of administered adipose-derived MSCs to promote revascularization in a mouse ischemia model . Altogether, these data support the paradigm that growth factors delivered locally by BMMSCs and by resident renal cells could act in concert to mitigate renal injury and hasten repair ( Fig. 20.2 ). The combined actions of several factors that decrease inflammation, oxidative stress and apoptosis at the same time would result in a greater renoprotective action than any single growth factor treatment, which could manifest systemic side-effects . Stem cell release, at the site of tubular injury, of HGF, IGF-1 and VEGF which possess mitogenic and chemotactic activity, could be instrumental to the recruitment of circulating or resident stem cell/progenitor cells which contribute to the reparative processes of the kidney. Of note, HGF, IGF-1 and FGF-2 have been shown to induce resident progenitor cell activation and migration within the infarcted border zone of the heart .

Together, these data have set the basis for initiation of clinical trials of AKI. However, several critical points need to be addressed, including the long-term risk of maldifferentiation into unwanted cells and the potential contribution of stem cells to malignancy .
Strategies to Enhance Stem Cell Effects
At present, converging pieces of evidence suggest that BMMSC-mediated renal regeneration passes through a paracrine secretion of mitogenic and vasculotropic factors. Therefore, it is evident that potentiating renal migration, engraftment, survival and paracrine effects of administered stem cells, using preconditioning or genetic modification strategies, could contribute towards optimizing the final beneficial effect of the therapy. This is particularly true when we approach pathologies such as chronic renal failure, featuring severely compromised renal function and histology.
Signals, Receptors and Trafficking of Bone Marrow Mesenchymal Stem Cells
Migration of systemically administered BMMSCs, endogenous stem cells or resident tissue stem/progenitor cells is a prerequisite to exert any type of effect at sites of injury. Recruitment of BMMSCs occurs through a complicated multistep process ( Fig. 20.3 ). As a first step, BMMSCs sensing the chemotactic factors from the remote injured tissue are released from their storage niche into circulation in a process known as mobilization. Then, stem cells migrate to the site of injury, where they interact with resident renal cells.

Knowledge about the nature of factors secreted from the injured tissue to mobilize BMMSCs from the bone marrow and guide them to the target tissue is relatively limited. However, it is believed that growth factors, cytokines and chemokines, which are upregulated under injury conditions and released into the circulation, can stimulate BMMSCs to downregulate the adhesion molecules that hold them in their niche. Many of these secreted mediators serve as chemotactic signals that guide BMMSCs to the appropriate microenvironment.
Chemokines and their receptors play a critical role in regulating the mobilization, trafficking and homing of BMMSCs. Chemokines are a family of chemotactic cytokines that were first identified on the basis of their ability to stimulate the migration of diverse cell types, particularly those of lymphoid origin . They are distinguished from other cytokines by being the only members of the cytokine family that bind to the superfamily of G-protein-coupled seven-transmembrane domain receptors. There are approximately 50 human chemokines, which segregate into four categories based on the position of the cysteine residues within the primary amino acid sequence: CC chemokines, CXC (C–X3–C motif) chemokines, the CX3C family, and lymphotactin as the sole member of its family . Cells are able to produce and release chemokines in a cell- and stimulus-specific manner and their expression is upregulated in response to a range of diseases . Consequently, expression of the appropriate chemokine receptors on BMMSCs is crucial for migration and engraftment in the damaged tissue. BMMSCs express a unique set of chemokine receptors : seven CC chemokine receptors, five CXC chemokine receptors and CX3CR1 ( Table 20.2 ). In cell biological assays, all of the tested chemokines, including CXCL12 (C–X–C chemokine ligand 12), CXCL13, CXCL16, CCL19 (C–C chemokine ligand 19), CCL5 and CCL25, stimulated chemotaxis of BMMSCs, and CXCL12 induced cytoskeleton F-actin polymerization . In a recent report, Ponte et al. demonstrated expression of CCR2, CCR3, CCR4 and CXCR4 in human BMMSCs and found that TNF increases CCR2, CCR3 and CCR4 expression .
Ref. | |
---|---|
CHEMOKINE RECEPTORS | |
CCR1, CCR7, CCR9, CXCR4, CXCR5, CXCR6 | |
CCR1, CCR4, CCR7, CCR10, CXCR5 | |
CCR1, CCR7, CXCR4, CXCR6, CX3CR1 | |
CCR6, CXCR4 | |
CXCR4 | |
CCR2, CCR3, CCR4, CXCR4 | |
CXCR1, CXCR2, CCR2 | |
ADHESION MOLECULES | |
ALCAM | |
ICAM-1, ICAM-2, vitronectin R β-chain | |
ICAM-3 | |
L-selectin | |
LFA-3, VLA-α 1 , VLA-α 2 , VLA-α 3 , VLA-α 5 , VLA-α 6 β 4 integrin | |
NCAM | |
HCAM, VCAM, hyaluronate receptor, VLA-β chain |
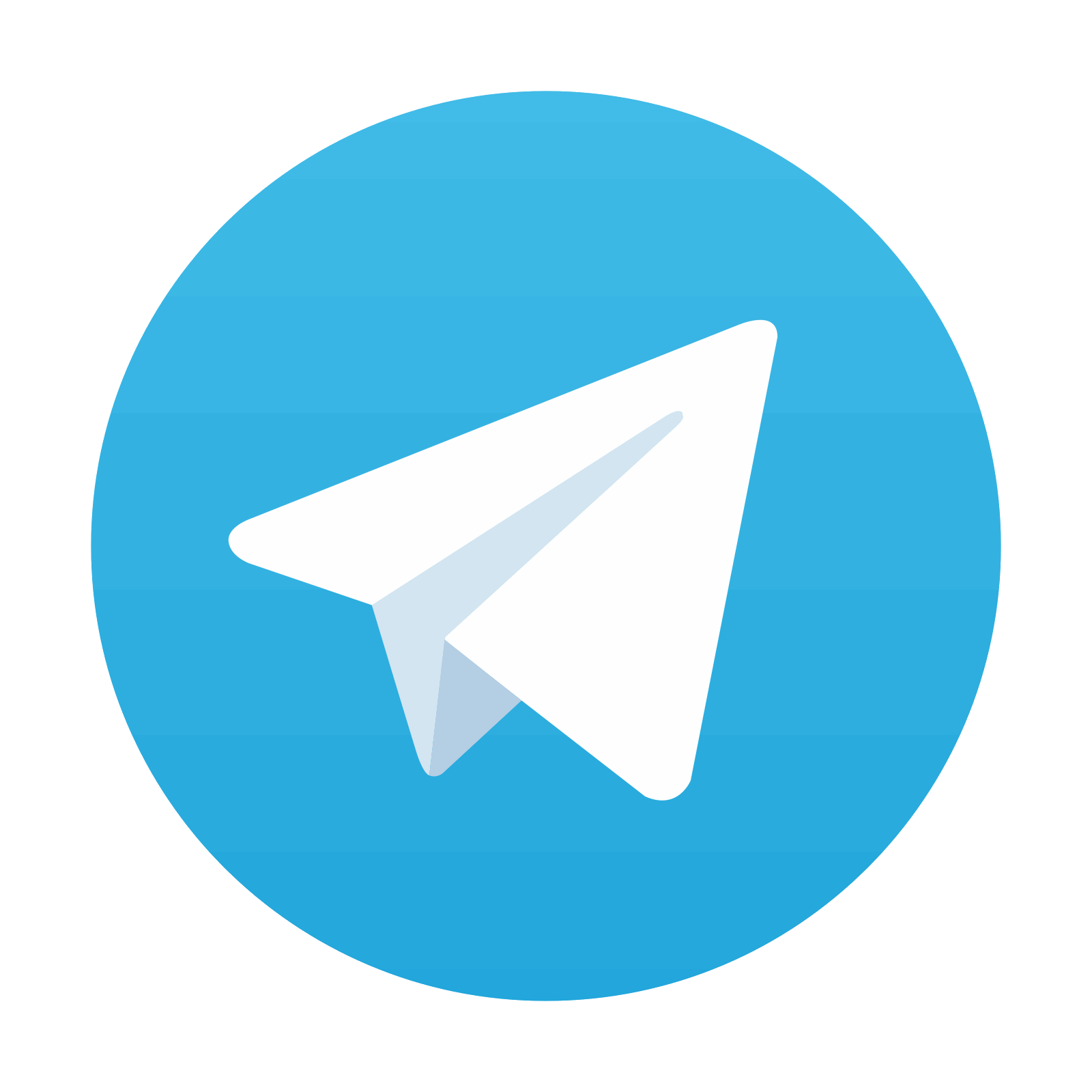
Stay updated, free articles. Join our Telegram channel

Full access? Get Clinical Tree
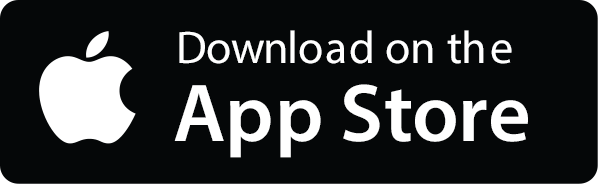
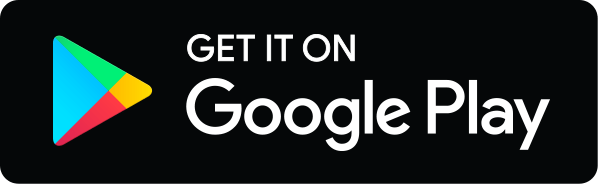
