17 Maike F. Eylert1 and Arjun K. Nambiar2 1 Department of Urology, University Hospital of Wales, Cardiff, UK 2 Department of Urology, Freeman Hospital, Newcastle‐upon‐Tyne, UK The embryology and anatomy of the bladder and urethra are well understood; however, the details of physiological function are an area of active research. We outline the essential points in our current understanding of bladder and urethral anatomy and physiology. Keywords: bladder anatomy; physiology; muscarinic receptors; adrenergic receptors; bladder contraction The bladder is an extraperitoneal pelvic organ. Only the dome and part of the posterior wall of the bladder are covered by parietal peritoneum, with coils of small bowel and sigmoid above. However, when full, the bladder rises out of the pelvis and may reach the level of the umbilicus. The bladder has no anatomical capsule. The bladder lies posterior to the symphysis pubis, separated from it by the space of Retzius or retropubic space. In the male, the bladder lies anterior to the rectum, with the fused fold of peritoneum in between (known as Denonvilliers fascia). Infero‐posteriorly on each side lies the ureter, the seminal vesicle, and the vas deferens (Figure 17.1). In the female, the bladder lies anterior to the vagina and uterus, with the vesicouterine pouch (of Douglas) in between. Again, the ureter lies infero‐posteriorly on either side. The female urethra lies within the anterior vaginal wall (Figure 17.2). Figure 17.1 (a) Sagittal section through the male pelvis showing main anatomical relations; (b) Coronal section. Figure 17.2 (a) Sagittal section through the female pelvis showing main anatomical relations; (b) Coronal section. Laterally to the bladder are extraperitoneal fat and connective tissue, containing venous plexuses, superior and inferior vesical vessels, obturator vessels, obturator nerves, and prostatic, vaginal, or uterine vessels. Further laterally are the obturator internus muscles covered by the obturator fascia. Inferiorly, in the male, is the prostate. Below the prostate or directly below the bladder (in the female) is the levator ani complex covered by pelvic fascia. The levator ani comprises of the pubococcygeus, iliococcygeus, ischiococcygeus, and the coccygeus, supporting all pelvic organs and contributing to sphincteric function. Additional support is derived from various ligaments and fasciae: pubourethral ligaments in the female, puboprostatic ligaments in the male, urethropelvic ligaments running laterally, and pubocervical fascia and cardinal ligaments and uterosacral ligaments in the female. Structurally the bladder is made up of interwoven fibres of smooth muscle (detrusor) that make up the body of the bladder (Figure 17.3). Distinct layers can only be defined at the trigone and around the bladder neck. Each detrusor muscle cell is separated from its neighbour by a basal lamina, but ‘pegs and sockets’ permit electrical excitation to pass from one cell to another. There is a rich lymphatic plexus within the detrusor muscle. Figure 17.3 Arrangement of the smooth muscles fibres of the bladder, they swoop from superficial to deep layers and curl around the internal meatus, more like a basket than a series of layers. The muscle layers are lined internally by the lamina propria and an inner urothelium that acts as a protective layer. The bladder urothelium is distensible along with the bladder muscle and forms an effective blood–bladder barrier to prevent uraemia [1]. In the empty bladder, the urothelium is some six cells thick, but when distended it is only one or two cells thick. A superficial layer of umbrella cells with numerous tight junctions results in separation of solutes in the urine from the intracellular and intravascular compartments. The lamina propria lies deep to the urothelium and consists of connective tissue and microvasculature of varying thickness. It contains the muscularis mucosae, a thin layer of myofibroblasts or suburothelial ‘interstitial cells’ distinct from the detrusor muscle. The base of the bladder forms the trigone, outlined by the interureteric bar, the ureteric orifices (2–3 cm apart), and the bladder neck. The ureters enter the bladder through oblique tunnels, approximately 1.5 cm in length, which prevent vesicoureteric reflux. These tunnels have a lubricated sleeve of connective tissue allowing movement up and down through the detrusor. Outside this sleeve is a sheath of muscle – Waldeyer’s sheath – whose muscle fibres run on the outside of the trigone down to the urethra (Figure 17.4). They have fewer cholinergic nerves and more adrenergic ones than the rest of the detrusor. During micturition they contract to elongate the ureters and prevent reflux (Figure 17.5). Figure 17.4 A special sheath of smooth muscle surrounds the lower end of the ureter, Waldeyer’s sheath. This is an important part of the antireflux mechanism. Figure 17.5 The action of Bell’s muscle is to draw the ureter down towards the trigone to elongate the antireflux tunnel of the ureter. In the male, the bladder neck is a collar of smooth muscle which merges distally with the muscle fibres of the prostate. Both are adrenergic and contract on stimulation of the sympathetic nerves (Figures 17.6 and 17.7). There are a few elastic fibres around the bladder neck. Figure 17.6 The efferent and afferent nerves of the bladder. Figure 17.7 The male bladder neck: there is an adrenergic internal sphincter which is normally kept closed. In the female, the bladder neck fibres merge obliquely into the wall of the urethra. They are cholinergic rather than adrenergic and play no active part in continence; indeed, in women with normal continence, the bladder neck is often found to be slightly open (Figure 17.8). Figure 17.8 The female bladder neck: the bladder neck is not usually closed at rest. The bladder is primarily supplied by branches of the internal iliac artery which divides into an anterior and posterior division (Figure 17.9). The posterior division gives the iliolumbar, lateral sacral, and superior gluteal, while the anterior division supplies three branches to the bladder (i.e. superior vesical, obliterated umbilical, and inferior vesical arteries), three to the viscera (i.e. middle rectal, vaginal, and uterine), and three parietal branches (i.e. obturator, inferior gluteal, and internal pudendal). Branches to the prostate and seminal vesicles are from the inferior vesical arteries Figure 17.9 Arterial supply of the bladder. The veins of the bladder drain into the vesicle plexus, which communicates with the prostatic, vaginal, or uterine plexus, and the internal iliac, ovarian, and superior haemorrhoidal and sacral veins, providing a direct route to the bones of the pelvis and vertebrae. The lymphatics of the bladder drain to the external and internal iliac nodes and obturator groups of nodes. The female urethra is 4 cm long and is made up of an epithelial lining, spongy submucosa full of microvasculature, a smooth muscle layer, and an outer connective tissue layer. Closure is largely achieved by the combined occlusive pressure of all four layers. The striated sphincter creates a ‘horseshoe shape’ or ‘signet ring configuration’ around the urethra at the level of the pelvic floor; it is deficient posteriorly. The male urethra measures about 20 cm in length, divided into posterior urethra and anterior urethra. The posterior urethra comprises of the prostatic urethra and membranous urethra. The anterior urethra comprises of the bulbar urethra, penile (or pendulous) urethra, and fossa navicularis. The anterior urethra is surrounded by the corpus spongiosum along its entire length. Closure of the male urethra is achieved at the level of the bladder neck, prostate, and at the distinct external sphincter just below the apex of the prostate gland. This will be considered in more detail in another Section (17.2.2), but by means of a summary, regions within the central nervous system which are relevant to bladder and urethral function are the frontal cortex, the pontine micturition centre (PMC) and Onuf’s nucleus (sacral micturition centre) (S2–4). The PMC is subdivided into a medial (M) and lateral (L) region. The sympathetic (T10‐L2), parasympathetic (S2–4), and somatic nervous system (S2–4) all interact in the control of urine storage and voiding function. The parasympathetic supply originates from nerve cells from the intermediolateral columns of the second to fourth sacral spinal segments (S2,3 mainly), pass through the spinal cord through the anterior primary division, called the ‘nervi erigentes’, which join the pelvic plexus. Fibres pass through the splanchnic nerves to the inferior hypogastric plexus and supply the bladder and urethra. These nerves supply cholinergic excitatory input to the smooth muscles. The parasympathetic ganglia for the bladder are located in the adventitia of the bladder and bladder base (50%) and within the bladder wall itself (50%) [2]. The sympathetic supply originates from nerve cells from the intermediolateral columns of the 10th thoracic to the 2nd lumbar segments. Fibres pass through the sympathetic chain as the hypogastric nerve and supply the bladder, prostate, and urethra. Afferent fibres convey painful stimuli such as that seen with bladder overdistention. Hence the vasovagal effect seen with a full bladder. These nerves supply inhibitory input to the smooth muscles. The hypogastric plexus are autonomic nerves lying inferior to the bifurcation of the aorta. It is a continuation of the intermesenteric plexus of nerves. Fibres from the superior hypogastric plexus enter the pelvis and descend in front of the sacrum and merge with the pelvic splanchnic nerves on either sides of the rectum, forming the inferior hypogastric plexuses and continue descent as the pelvic plexus. The pelvic splanchnic nerves supply parasympathetic fibres. Pelvic nerves can be damaged during pelvic operations, especially bowel surgery. The somatic innervation is via the pudendal nerve (S2,3,4) originating from the second to fourth sacral spinal segments, specifically from Onuf’s nucleus, which lies in the medial part of the anterior horn of the spinal cords, in addition to nerves from the inferior hypogastric plexus. These nerves supply the skeletal muscles of the pelvis and the muscles of the sphincter mechanism. Afferent sensory fibres from the urethra travel via the pudendal nerve. Normal bladder function is divided into storage and voiding. However, this is a purely academic division. The urinary bladder is a quintessential organic ‘machine’; once trained, it usually goes about its business with very little fuss. A ‘normal’ bladder will come to its owner’s attention for little more than one or two minutes a day. The main purpose of the urinary bladder is to receive urine from the kidneys and act as a compliant pouch to store that urine, until such time as it is socially appropriate and convenient to void. The normal bladder fills with urine at a rate determined by input via food and drink, and fluid loss in sweat, faeces, and respiration. As it fills, there is no increase in pressure within normal bladder capacity. This is due to the compliance of the bladder wall, which is the relationship between change in bladder volume by change in detrusor pressure (expressed by the formula ΔDV/ΔDP). The bladder is highly compliant (minimal change in pressure despite large changes in volume) due to the bladder connective tissue’s elasticity and the smooth muscle’s ability to increase their length without any change in tension. A sensation of filling is appreciated by most people when the bladder contains about 250 ml. At this stage, micturition can normally be postponed for a considerable time. Intra‐detrusor pressure does not normally rise until the bladder contains 500 ml or more, and only then ‘urgency’ is perceived. The exact volume at which this takes place varies considerably from one patient to another. Although the lining of the bladder is virtually waterproof, some ions and small molecules can be absorbed. Absorption by the bladder may also be altered in some diseases (e.g. bladder pain syndrome). There are a number of reflexes controlling urine storage and voiding, including essential coordination from the PMC. Higher centres in the frontal cortex play a largely inhibitory role during storage and activate the micturition reflex at a socially convenient time and place. The input from higher centres can override reflex activity to store or to void (i.e. micturition can be postponed despite reaching the person’s individual usual bladder capacity, and equally they can choose to void despite a fairly empty bladder). At least four functionally relevant reflexes are known [3], all of which start with afferent impulses originating from the urothelium, the lamina propria, or the detrusor (Figure 17.10): Figure 17.10 Muscarinic and adrenergic pathways to muscular contraction. Reflexes 1 and 2 ensure complete bladder emptying. Reflexes 3 and 4 create a gating mechanism, whereby small amounts of afferent input are prohibited from being transmitted to efferent fibres. The origin of afferent impulses and the neurotransmitters involved are an area of active research [3]. As a summary, the urothelium and myofibroblasts are linked by a nervous plexus, which together act as a stretch receptor releasing adenosine triphosphate (ATP). The majority of these afferent impulses are carried along parasympathetic nerves to the PMC as well as the cerebral cortex and communicate normal bladder filling. Afferents from the trigone travel along sympathetic nerves to the PMC and cerebral cortex, communicating definite fullness. Afferents from the urethra travel in the pudendal nerve to the same centres communicating urgency. However, M2 and M3 muscarinic receptor activity of myofibroblasts have also been found to correlate with urgency scores [4], indicating a much more complex situation in disease. Smooth muscles contractions result from an increase in Ca2+ in the sarcoplasm from either within cellular stores or entrance from extracellular channels. The increase in Ca2+ combined with calmodulin (abbreviation for calcium‐modulated protein) forming the Ca2+4‐calmodulin complex, activates myosin light chain kinase (MLCK) leading to the phosphorylation of myosin and leading to myosin contraction (i.e. smooth muscle contraction). Relaxation occurs by dephosphorylation of myosin by myosin light chain phosphatase (MLCP), which is induced by protein kinase A (PKA). Bladder contraction is thought to be mainly mediated by acetylcholine (ACh) neurotransmission through the parasympathetic and somatic motor nerves. Muscarinic receptors are targets for ACh and are expressed throughout the urinary tract. Five muscarinic receptor subtypes are identified (m1–5); however, in the bladder M2 and M3 are predominantly expressed. M2 receptors are expressed nearly nine times as much as M3, but M3 seem to have a more pivotal role in normal bladder contractions, and M2 may have a more active role in pathological bladder contractions [5]. Similarly, adrenergic receptors (namely β receptors) mediate bladder relaxation, with β3 being the driving receptor. M3 receptors are coupled with Gq/11 proteins, which activate phospholipase C enzyme (PLC). This, in turn, converts phosphoinosites (PIP2) to inositol triphosphate (IP3) and diacylglycerol (DAG). DAG activates protein kinase C (PKC) leading to opening of the gated calcium channels and inhibits relaxation, while IP3 binds to receptors on the sarcoplasmic reticulum releasing Ca2+ from intracellular stores leading to an overall increase in intracellular Ca2+ which binds with calmodulin leading to activation of MLCK leading to myosin light chain (MLC) contraction (Figure 17.11). In the urethra, α‐adrenoceptors share a common final pathway with the muscarinic receptors to lead to muscular contractions. Figure 17.11 Muscarinic and adrenergic pathways to muscular relaxation. M2 receptors are coupled with Gi protein, reduce cAMP production by influencing adenylate cyclase activity. However, β‐receptors are the main drivers behind bladder smooth muscle relaxation. Stimulation of these receptors leads to increase in conversion of ATP to cAMP by activating adenylyl cyclase. Adenosine 3’5’‐cyclic monophosphate (cAMP) drives calcium back into the sarcoplasmic reticulum leading to muscular relaxation (Figure 17.12). Purinergic receptors (P2 receptors) also contribute to smooth muscle function in response to release of ATP. Although contraction is mainly through ACh release, in pathological bladders, purinergic receptor‐mediated contraction increase. P2 receptors are classified into families of metabotropic G protein coupled (P2Y) and ionotropic ligand‐gated nonspecific cation channels (P2X) [6]. The metabotropic receptors cause bladder relaxation of smooth muscles, via cAMP‐dependent PKA activity, while the ionotropic receptors cause contraction by increasing Ca2+ influx into the cell through Ca2+ channels. Nitric oxide synthase (NOS) is formed in three tissue types, neuronal NOS, inducible NOS from macrophages, and endothelial NOS, all present in the lower urinary tract and lead to increase in nitric oxide (NO). The production of NO leads to muscle relaxation, by stimulating nitrergic receptors leading to an increase in cyclic GMP (cGMP) leading to a decrease in intracellular calcium and the ensuing smooth muscle relaxation. The trigone, bladder base and neck, and urethra (especially in men, including the prostate) are mainly regulated by adrenergic receptors to cause muscular contractions with relative absence of muscarinic and β receptors.
Bladder and Urethra Structure and Function
Abstract
17.1 Anatomy
17.1.1 Gross Anatomy of the Bladder
17.1.2 Bladder Wall
17.1.3 Trigone, Ureters, and Bladder Neck
17.1.4 Arteries, Veins, and Lymphatics
17.1.5 Female Urethra
17.1.6 Male Urethra
17.1.7 Neuroanatomy Relevant to the Bladder and Urethra
17.2 Physiology
17.2.1 Overview
17.2.2 Neurological Control of Urine Storage and Micturition
17.2.2.1 Smooth Muscle Contraction in the Urinary Tract
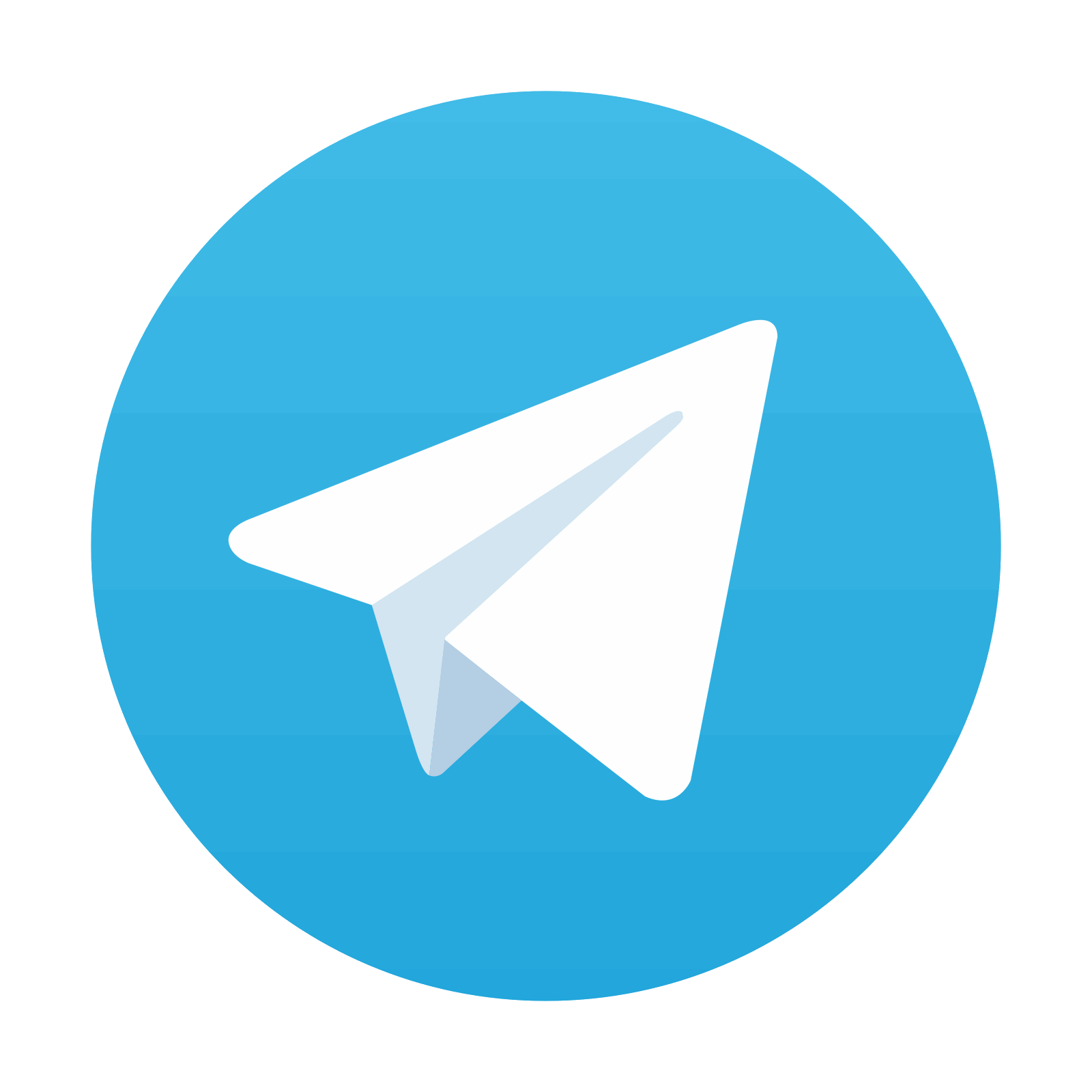
Stay updated, free articles. Join our Telegram channel

Full access? Get Clinical Tree
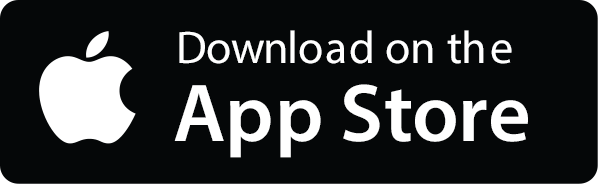
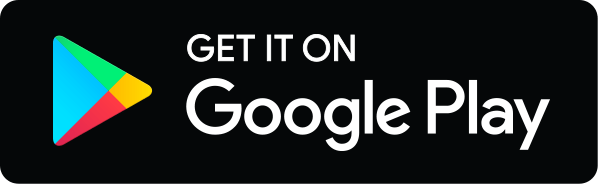