Fig. 11.1
Bar charts of five bile acids that are differentially expressed among patients with Child–Pugh A, B, and C cirrhosis. Serum levels of GCA, GCDCA, TCA, TCDCA, and GUDCA are gradually increased among Child–Pugh A, B, and C. CP A Child–Pugh A, CP B, Child–Pugh B, CP C, Child–Pugh C
11.2 Viral Hepatitis B
11.2.1 Etiology
The most common causes of viral hepatitis are persistent hepatotropic viruses such as hepatitis B virus (HBV) and hepatitis C virus (HCV). HBV and HCV infections are a global health problem and the leading causes of chronic hepatitis, which may develop into LC with/without HCC.
The advent of sensitive assays for the detection of HBV and the availability of potent antiviral agents have improved the management of patients with chronic hepatitis B; however, current treatment cannot eradicate the virus. An estimated 350–400 million people are chronically infected with hepatitis B (defined as hepatitis B surface antigen positivity for ≥6 months). More than 780,000 people die every year as a result of complications of hepatitis B, including cirrhosis and HCC (World Health Organization report; http://www.who.int/immunization/diseases/hepatitisB/en/).
Chronic hepatitis B infection can be treated with drugs, including oral antiviral agents or interferon. Treatment can slow the progression of cirrhosis, reduce the incidence of liver cancer, and improve long-term survival. However, in most people, the treatment does not cure hepatitis B infection but only suppresses replication of HBV. In addition, lamivudine, adefovir, entecavir, telbivudine, tenofovir, and tenofovir alafenamide (prodrug of tenofovir) are all nucleoside/nucleotide analogs targeting HBV reverse transcriptase. Hepatic uptake of tenofovir alafenamide is facilitated by organic anion-transporting polypeptide (OATP)1B1 and 1B3, and these transporters also uptake bile acids [7].
11.2.2 HBV Entry Receptor
Na+/taurocholate cotransporting polypeptide (NTCP) is an HBV entry receptor. NTCP (also known as SLC10A1) is a member of the solute carrier family 10 and localizes to the basolateral membrane of hepatocytes. The key function of NTCP is the Na+-dependent uptake of bile acids, allowing maintenance of enterohepatic circulation of bile acids. HBV interacts with NTCP through the pre-S1 domain of HBV-encoded large envelope protein [8]. The pre-S1 domain of an HBV-encoded large surface envelope protein plays a role in virus particle entry [9].
siRNA-mediated knockdown of NTCP reduces HBV infection in primary human hepatocytes [10]. NTCP can serve as a therapeutic target. Indeed, myrcludex B, cyclosporin A, and some NTCP inhibitors can inhibit HBV entry by targeting NTCP (Fig. 11.2). Myrcludex B binds to NTCP and inactivates its receptor function for HBV. Cyclosporin A is an immunosuppressant that is classified as a calcineurin inhibitor and inhibits HBV infection by targeting NTCP [11].


Fig. 11.2
Schematic life cycle of HBV [11]. Myrcludex B, cyclosporin A, and some NTCP inhibitors inhibit the viral entry process by targeting NTCP
11.2.3 Bile Acids Promote HBV Replication
Nuclear receptors regulate HBV promoters and enhancers. Liver-enriched nuclear receptors perform a pivotal role in the regulation of HBV transcription by binding to both HBV enhancer I and enhancer II. The farnesoid X receptor (FXR) is a metabolic nuclear receptor expressed in the liver via regulation of the expression and function of genes involved in bile acid synthesis, uptake, and excretion. FXRα/retinoid X receptor (RXR)α genes have emerged as key factors involved in the maintenance of bile acid and cholesterol homeostasis. FXRα and c-Jun N-terminal kinase (JNK)/c-Jun signal transduction pathway mediate the regulatory effect of bile acids. FXRα/RXRα heterodimeric nuclear receptors can also mediate ligand-dependent HBV transcription and replication when activated by bile acids. CDCA treatment leads to JNK/c-Jun and HBV enhancer I activation that also results in HBV enhancer II induction and enhances the level of HBV biosynthesis [12], through FXRα, which is implicated in the metabolic regulation of HBV transcription [13]. Small heterodimer partner (SHP) is also involved in the bile acid-mediated regulation of HBV gene expression [14]. Bile acids induce SHP, which mediates the inhibitory effects on HBV replication [15, 16].
HBV infection alters the expression of genes in bile acid metabolism, most notably CYP7A1, which encodes a key enzyme involved in bile acid synthesis. Hepatic cytochrome P450 (CYP)7A1 expression is increased in chronic HBV patients. FXR nuclear translocation decreases, and expression of its transcriptional target, SHP, is reduced in the liver of HBV-infected patients. SHP is a transcriptional repressor of CYP7A1, and its decrease could explain CYP7A1 induction [17] (Fig. 11.3).


Fig. 11.3
In HBV infection, bile acid synthesis is increased via upregulation of CYP7A1 and downregulation of SHP, which is a transcriptional repressor of CYP7A1. CYP7A1 is a key enzyme involved in bile acid synthesis. NTCP is an HBV entry receptor
11.3 Viral Hepatitis C
11.3.1 Etiology
Chronic HCV infection is one of the most common chronic liver diseases and accounts for 8,000–13,000 deaths each year [18]. HCV can cause both acute and chronic hepatitis. Fifty to 85 % of patients with HCV infection develop chronic hepatitis C. Five to 30 % of chronically infected individuals develop cirrhosis over a 20–30-year period. Chronic HCV infection often follows a progressive course over many years and can ultimately result in cirrhosis and HCC. Current treatments such as direct-acting antivirals for chronic HCV infection achieve high sustained virological response rates, lower the incidence of side effects, have few drug–drug interactions, and/or shorten the duration of treatment. In the near future, in HCV hepatitis, only acute hepatitis will remain, and chronic hepatitis will disappear and become a rare disease by 2036 [19].
11.3.2 Bile Acids Promote HCV Replication
Bile acids impair interferon (IFN)-α and IFN-β signaling in hepatocytes, natural killer cells, macrophages, and lymphocytes. Hydrophobic bile acids blunt IFN-α-dependent Janus kinase 1 and tyrosine kinase 2 (Tyk2) activation. These effects of bile acids on IFN signaling may limit the therapeutic use of IFNs in hepatitis B and C. The success of HCV therapy with IFN is dependent on serum bile acid levels. In patients with high serum levels of bile acids, IFN therapy shows higher failure rates [20]. Low concentrations of unconjugated bile acids (DCA/CDCA) and high concentrations of conjugated bile acid (GCDCA) increase HCV RNA replication in HCV genotype 1b replicon [21], [22]. Bile acids (CA and CDCA) also increase HCV RNA replication in HCV genotype 2a replicon [23].
11.3.3 Ursodeoxycholic Acid (UDCA) and Viral Hepatitis
Direct-acting antivirals are outstandingly successful in the eradication of HCV, and anti-HBV drugs achieve sustained suppression of HBV. In addition, we have palliative treatment with UDCA for chronic viral hepatitis. In patients with chronic viral hepatitis, including HBV and HCV, UDCA improves serum liver transaminase levels without serious adverse events. However, UDCA does not eradicate viral markers. In addition, there is no compelling evidence showing that these bile acids beneficially affect viral markers, mortality, cirrhosis development, need for liver transplantation, or liver histology in patients with acute or chronic hepatitis B and chronic hepatitis C [24]. UDCA has a direct protective effect on hepatocytes against apoptosis induced by endogenous bile acids and stimulates bile acid secretion, hence reducing retention of toxic bile acids and, therefore, cell injury [25, 26]. The mechanisms of UDCA action include reduction of toxic endogenous bile acids, membrane-stabilizing activity, and immunomodulatory effect. At the mitochondrial level, UDCA inhibits JNK-dependent Fas trafficking to the plasma membrane and activates survival signals such as epidermal growth factor receptor and mitogen-activated protein kinase. In addition, UDCA inhibits apoptosis mediated by endoplasmic reticulum stress [27].
11.4 HCC
11.4.1 Etiology
Liver cancer includes two major types: HCC and cholangiocellular carcinoma (CCC). HCC results in between 250,000 and 1 million deaths globally each year. Worldwide, HCC is the sixth most prevalent cancer and the second leading cause of cancer-related death [28]. Many insults that lead to chronic liver damage, such as intoxication, viral infection, cholestasis, or metabolic diseases, increase the risk of HCC. HBV and HCV infections appear to be the most significant causes of HCC worldwide. Chronic HBV infection is the leading cause of HCC in Asia and Africa, and HCV infection is the leading cause of HCC in North America, Europe, and Japan [29]. The annual incidence of HCC in HBV carriers is 0.5–1 % in patients without cirrhosis and 2.5 % per year in patients with cirrhosis. The annual incidence of HCC in HCV-related cirrhotic or pre-cirrhotic liver is reported as 4–8 %.
11.4.2 Bile Acids and Development of HCC
Abnormally high levels of bile acids induce hepatocyte DNA damage, which can increase the mutation rate of tumor suppressor genes and oncogenes. Furthermore, bile acids can induce cell death and inflammation to promote carcinogenesis. Bile acid homeostasis is disrupted in HCC patients with an elevated serum bile acid level as a proposed marker for HCC. However, the underlying mechanisms remain largely unknown. In HCC patients, serum CA, CDCA, and DCA levels are elevated, but serum UDCA level is not elevated [30]. The concentration of CDCA is increased in human HCC tissues [31]. GCDCA contributes to the development of HCC in a mechanism that enhances the antiapoptotic function of myeloid leukemia cell differentiation protein-1 (Mcl-1) [32]. Mcl-1 is a major antiapoptotic member of the Bcl2 family, which has survival and oncogenic properties. In HCC, this is characterized by a marked increase in the CDCA/CA ratio in both serum and urine [33]. This indicates predominant synthesis of CDCA in HCC tissues.
11.4.3 Bile Acid Transporters and HCC
Mutations in the bile salt export pump (BSEP), ABCB11, result in liver tumor formation [34]. BSEP is an efflux transporter that plays an important role in the disposition of bile salts from the liver, and it is predominantly expressed at the apical (canalicular) membranes of hepatocytes [35]. Expression of BSEP is downregulated in HCC cell lines [36]. Furthermore, expression of bile acid uptake transporter NTCP is reduced in most cases of HCC [37].
The elevated bile acid concentrations play a role as an endogenous promoter in hepatocarcinogenesis in rats. mdr2 gene deletion mice, which have accumulation of bile acids in the liver, display progressive HCC [38]. Multidrug resistance (Mdr)2 (MDR3 in humans) is localized at the canalicular membrane of hepatocytes and is responsible for the ATP-dependent translocation of phosphatidylcholine [35]. Bile acids may directly contribute to the development of HCC in humans.
The absence of FXR and its downstream target SHP results in unsuppressed bile acid synthesis, and the injured liver may fail to complete normal regeneration, leading to repeated cycles of cell necrosis and compensatory proliferation of hepatocytes. This irregular proliferation of hepatocytes is an important factor in promoting hepatocarcinogenesis [39]. FXR, which is necessary for maintaining bile acid homeostasis, prevents bile acid-induced hepatocyte DNA damage and transformation. Therefore, the role of FXR in the promotion of liver regeneration could be an intrinsic mechanism for the prevention of liver carcinogenesis [40].
Bile acids are also ligands for G-protein-coupled bile acid receptor 1 (GPBAR1, also known as TGR5 or membrane-type receptor for bile acids). The receptor is implicated in the suppression of macrophage functions and regulation of energy homeostasis by bile acids. In HCC patients with HBV, serum TGR5 promoter methylation is detected [41]. TGR5 also modulates the activation of signal transducer and activator of transcription (STAT)3. STAT3 is a transcription factor and is traditionally considered to be an oncogene in HCC; however, recent reports demonstrate the antioncogenic functions of STAT3 in HCC development [42, 43]. TGR5 may be a potential tumor suppressor and biomarker in liver cancer.
Fibroblast growth factor (FGF)19 is also implicated in the development of HCC. FGF19 (also called FGF15 in rodents) is an endocrine hormone of the FGF family that regulates bile acid, carbohydrate, and lipid and energy metabolism. EGF19 selectively binds to FGFR4, which can be further enhanced by co-receptor FGFR4–β-Klotho. FGF19–FGFR4 signaling is also implicated in hepatocellular tumorigenesis. In rodents, an engineered FGF19 (M70) fully retains the biological activity of FGF19 and inhibits FGF19-mediated liver tumor formation [44].
OATP1B3 is expressed on the basolateral membranes of hepatocytes and is encoded by the SLCO1B3 gene. OATP1B3 is a drug transporter mediating the active hepatic uptake of bile acids and gadolinium–ethoxybenzyl–diethylenetriamine pentaacetic acid (Gd–EOB–DTPA). Magnetic resonance images acquired 10–20 min after Gd–EOB–DTPA injection, a period known as the hepatobiliary phase, are useful for HCC detection. HCC shows low signal intensity in the hepatobiliary phase of Gd–EOB–DTPA-enhanced magnetic resonance imaging. OATP1B3 expression is often decreased (or absent) in HCC relative to that of the liver parenchyma [45, 46] (Fig. 11.4).


Fig. 11.4
Hepatic OATP1B3 expression in an HCC patient. No OATP1B3 expression was observed in the area of HCC, whereas OATP1B3 expression was observed in the non-tumor area in an immunohistochemical study observed by a laser scanning microscopy (Original magnification: ×200)
11.4.4 Senescence-Associated Secretory Phenotype (SASP)
Secondary bile acids, particularly DCA, cause DNA damage through production of reactive oxygen species [47] and are a major risk factor for promoting colon tumorigenesis. In the liver, DCA provokes SASP in senescent hepatic stellate cells [48], which in turn secrete various inflammatory and tumor-promoting factors, thus facilitating HCC development.
Cellular senescence is now recognized as a potent tumor-suppressive mechanism that arrests the growth of cells at risk for malignant transformation. However, recent studies showed that senescent cells develop altered secretory activities that may induce changes in the tissue microenvironment, relaxing its control over cell behavior and promoting tumorigenesis [49]. SASP represents cellular senescence changes in gene expression that result in secretion of a signature profile of inflammatory cytokines, chemokines, and proteases associated with inflammation and malignancy. SASP is also known as the senescence-messaging secretome [49].
11.4.5 UDCA and HCC
UDCA is a relatively hydrophilic bile acid and is well known to have a hepatocellular protective effect in a variety of liver diseases. In HCV cirrhosis patients, UDCA decreases the risk of HCC [50]. UDCA treatment reduces hepatocarcinogenesis by inducing apoptosis [51]. Moreover, UDCA induces DLC1, which is a tumor suppressor gene for HCC, by inhibiting proteasomal DLC1 (deleted in liver cancer 1) degradation in a ubiquitin-independent manner [52]. DLC1 induction participates in UDCA-induced suppression of HCC cellular growth. In HepG2 cells, combined treatment with UDCA and oxaliplatin suppresses carcinogenesis, shifting oxaliplatin-induced necrosis to apoptosis. UDCA also triggers the necrosis-to-apoptosis switch when combined with other platinum-based chemotherapeutic drugs including cisplatin and carboplatin [53].
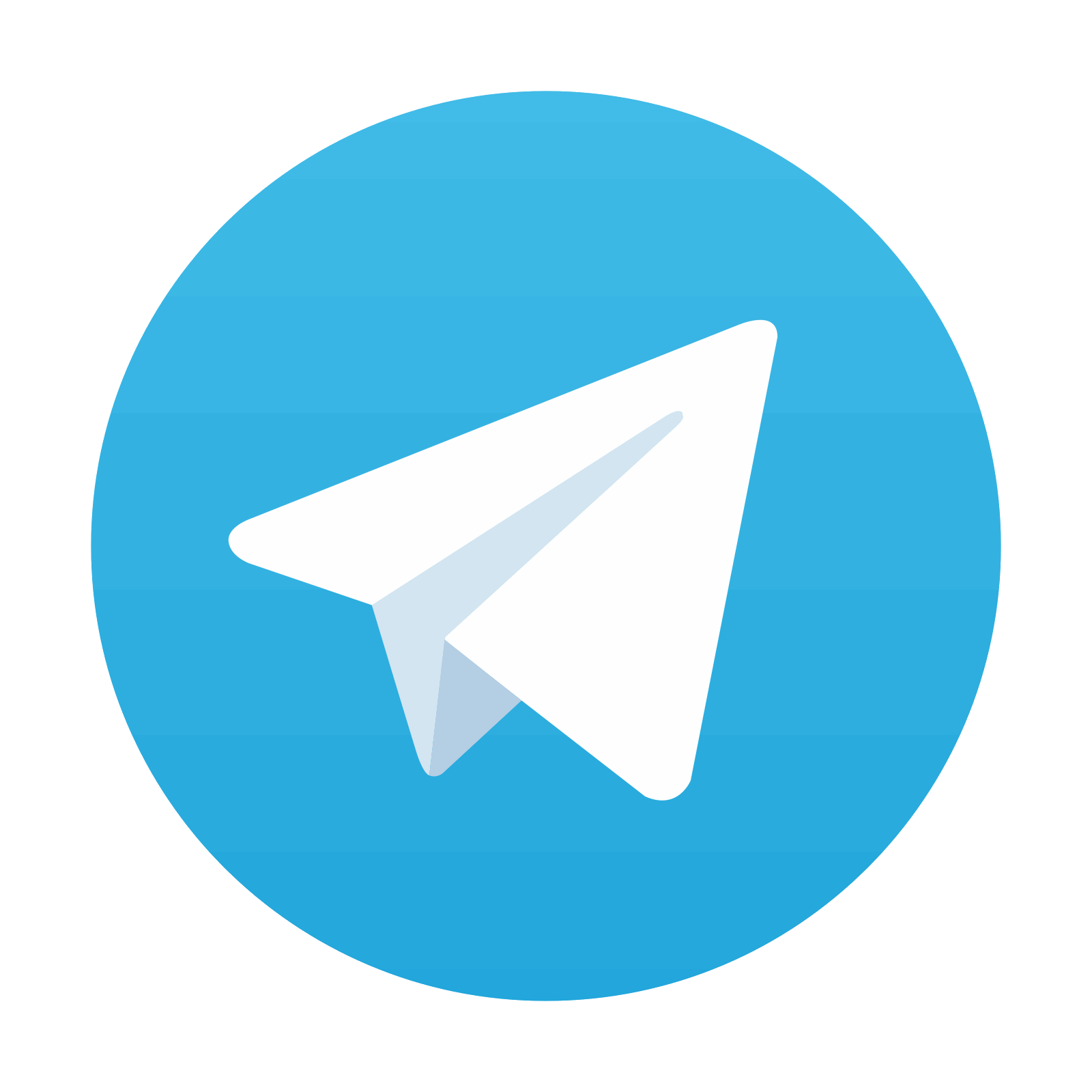
Stay updated, free articles. Join our Telegram channel

Full access? Get Clinical Tree
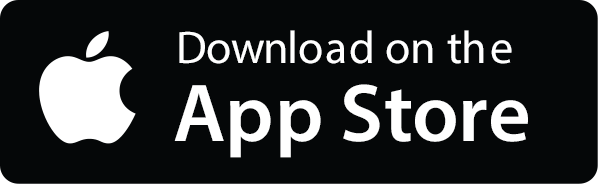
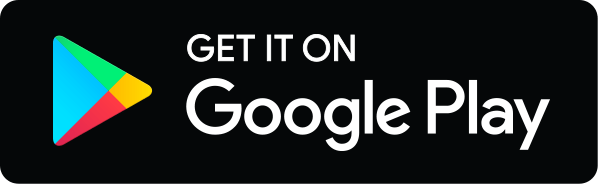