Fig. 15.1
FXR-dependent metabolic regulation. FXR signaling regulates lipid and glucose metabolism. FXR signaling reduces lipogenesis (SREBP1c) and induces fatty acid β oxidation (PPARα) and plasma TG clearance (LPL and VLDL-R), resulting in decreased plasma VLDL levels. Plasma HDL-C uptake is also increased by FXR and SRB1 activity. FXR signaling upregulates glycogenesis and glucose uptake, downregulates glycogenolysis, and reduces blood glucose levels. Hepatic FXR signaling is also associated with gluconeogenesis, but the controlling mechanism is still unclear. Angptl3 angiopoietin-like protein 3, ApoCII/CIII apolipoprotein CII/CIII, FXR farnesoid X receptor, G6Pase glucose-6-phosphatase, HDL-C high-density lipoprotein cholesterol, LPL lipoprotein lipase, PEPCK phosphoenolpyruvate carboxykinase, PPARα peroxisome proliferator-activated receptor α, SR-B1 scavenger receptor B1, SREBP1c sterol regulatory element-binding protein 1c, TG triglyceride, VLDL-R very low-density lipoprotein receptor
15.2.2 Lipid Metabolic Regulation of FXR
BAs are associated with regulating triglyceride (TG) metabolism as well as cholesterol metabolism. The relationship between BAs and TG was first reported in the treatment of gallstones with CDCA. CDCA treatment decreased the serum TG level in patients with gallstones [26]. In fact, BAs or a synthetic FXR agonist affected TG metabolism via several mechanisms including the FXR-mediated pathway. SHP, the target of FXR, suppressed the upregulation of sterol regulatory element-binding protein-1c (SREBP-1c), the master regulator of fatty acid and TG synthesis, to reduce the expression of the lipogenic genes such as acetyl-CoA synthetase, acetyl-CoA carboxylase, stearoyl CoA desaturase 1, and fatty acid synthase [7, 27]. In addition, the TG-lowering effects were attenuated in SHP knockout mice, indicating that SREBP-1c-mediated lipogenesis is inhibited in an FXR-SHP-dependent manner [7]. Additionally, FXR activation by BAs increases the expression of apolipoprotein (Apo) CII to activate lipoprotein lipase, which stimulates TG hydrolysis in very low-density lipoprotein (VLDL) and chylomicrons and facilitates TG clearance from the serum [28]. The expressions of ApoCIII and angiopoietin-like protein 3 (Angptl3), inhibiting lipoprotein lipase activity, were repressed by FXR stimulation with BAs [29–31]. In addition, FXR induces the gene expression of the VLDL receptor, which is responsible for plasma TG clearance [32].
BAs also suppress the expression of microsomal triglyceride transfer protein (MTP) and ApoB by FXR-independent mechanisms to inhibit the formation of VLDL and chylomicrons [33]. Not only VLDL but also high-density lipoprotein (HDL) clearance is suggested to be under the modulation of BAs. The expression of scavenger receptor B1 (SRB1), a molecule in charge of hepatic uptake of HDL, is reduced, and HDL-C is elevated in FXR knockout mice [34]. In addition, the administration of an FXR ligand increases hepatic SRB1 expression and decreases HDL-C levels [35].
BAs control other major regulators of lipid metabolism such as PPARα and pyruvate dehydrogenase kinase-4 (PDK4). The nuclear receptor PPARα, which is activated by free fatty acids (FFA), decreases serum TG levels and exerts an important role for controlling enzymes participating in fatty acid oxidation [36]. A study suggested that BAs regulate PPARα directly via FXR in humans, although not in mice [37]. PDK4 is also upregulated by BAs in an FXR-dependent manner, resulting in the inactivation of pyruvate dehydrogenase, a decrease in glycolysis, and an increase in fatty acid β oxidation (Fig.15.1) [38].
15.2.3 Anti-atherosclerotic Effect of FXR
Some researches show that FXR signaling is associated with onset of atherosclerosis. ApolipoproteinE (apoE)-FXR double-knockout mice fed with atheroma-induced diet developed severe atherosclerosis [39]. On the other hand, foam cell formation and atherosclerosis development are inhibited in both low-density lipoprotein receptor (LDLR)-FXR double-knockout mice and apoE-FXR double-knockout mice [40, 41]. FXR activation by the synthetic FXR agonist of INT-747 reduced formation of aortic plaque [42]. And more, treatment of synthetic FXR agonist of WAY-362450 also inhibited diet-induced hypertriglyceridemia and aortic lesion formation [43].
15.2.4 Intestinal FXR and Metabolic Regulation
Intestinal FXR has been recently identified as a possible target for improving metabolic syndrome. Intestinal FXR activation induces the expression of fibroblast growth factor (FGF)15/19, and several studies have demonstrated that FGF15/19 affects glucose and energy homeostasis. FGF19 transgenic mice showed increased hepatic β oxidation, reduced adipose tissue weight, and improved glucose tolerance and insulin sensitivity [44]. In mice, hepatic acetyl-CoA carboxylase 2 (ACC2) mRNA was decreased, and the mass of the BAT was increased. ACC2 exists at the mitochondrial membrane and converts acetyl-CoA to malonyl-CoA. ACC2 activation results in an elevation of malonyl-CoA levels, which inhibit carnitine palmitoyl transferase-1 (CPT-1) activation [45]. CPT-1 transfers FFA from the cytoplasm to the mitochondria and induces fatty acid β oxidation. In addition, hyperglycemia is improved upon administration of FGF19 protein in obese mice [46]. Furthermore, activation of intestinal FXR by administration of fexaramine, an FXR agonist, improved obesity and insulin resistance by inducing FGF15, changing the serum BA composition and stimulating systemic TGR5/M-BAR [47]. These results suggest the possibility that metabolic disease is improved through the intestinal FXR-FGF15/19 signaling pathway.
The primary BAs excreted into the intestine become deconjugated BAs and are converted into various secondary BAs by microbial enzymes [48]. In germfree (GF) mice, reduction of gut microbiota facilitating BA deconjugation leads to increased tauro-beta-muricholic acid (T-β-MCA) and decreased beta-muricholic acid. In comparison to conventionally raised mice, FXR-dependent BA synthesis is reduced in GF mice by increasing T-β-MCA of FXR antagonist. Therefore, the microbiota is associated with regulating BA homeostasis via the inhibition of intestinal FXR signaling by changing the BA composition [49]. In contrast to previous reports, recent studies have noted that alteration of the BA composition by microbiota and inhibition of intestinal FXR activity improved lipid and glucose metabolism. Increased T-β-MCA reduced intestinal FXR activation and decreased serum ceramide levels through repression of ceramide synthesis, which reduced expression of hepatic SREBP-1c and resulted in an improvement of obesity and nonalcoholic fatty liver disease (NAFLD) [50–52]. Additionally, intestinal FXR deactivation may also improve glucose metabolism as well as lipid metabolism. FXR activation in L cells decreased glycolysis, proglucagon expression, and cAMP levels [22]. Thus, GLP-1 production and secretion were inhibited [22]. Conflicting opinions suggest that microbiota regulation of BA homeostasis and intestinal FXR activation are involved in controlling hepatic lipid accumulation and glucose metabolism (Fig.15.2). Further studies are needed to clarify the roles of intestinal FXR signaling for improving metabolic diseases.


Fig. 15.2
Conflicting mechanisms of metabolic regulation via intestinal FXR activity. (a) Inhibiting FXR activation decreases hepatic TG levels and improves glucose metabolism. (b) Intestinal FXR activation of FXR agonist leads to FGF15/19 production and improves NAFLD. Synthesized FGF15/19 changes BA metabolism and serum BA composition, which causes TGR5/M-BAR activation, reduced inflammatory cytokine release, and improved insulin resistance. BABR bile acid-binding resin, FGF15/19 fibroblast growth factor 15/19, FXR farnesoid X receptor, NAFLD nonalcoholic fatty liver disease, SREBP1c sterol regulatory element-binding protein 1c, Tβ-MCA tauro-β-muricholic acid
15.3 TGR5/M-BAR Signaling and Metabolic Syndrome
15.3.1 Glucose Metabolic Regulation of TGR5/M-BAR
BA administration improved metabolism including glucose tolerance and insulin resistance. The beneficial effects of BAs, such as decreasing gluconeogenesis and increasing glycogen synthesis, seem to occur not only via FXR signaling but also via other signaling molecules such as TGR5/M-BAR. TGR5/M-BAR signaling induces GLP-1 secretion in murine enteroendocrine STC-1 cells [53]. Moreover, a semisynthetic TGR5/M-BAR agonist 6-ethyl-23(S)-methylcholic acid (6EMCA or INT-777 [54]) stimulates GLP-1 secretion in both murine and human enteroendocrine cells. In this study, knockdown of TGR5/M-BAR by shRNA reduced 6EMCA-induced secretion of GLP-1 in STC-1 cells [12]. A natural TGR5/M-BAR agonist oleanolic acid also improves glucose metabolism [55]. This evidence indicates the importance of TGR5/M-BAR in GLP-1 secretion. An in vivo study with TGR5/M-BAR knockout and TGR5/M-BAR transgenic mice strongly supports the relationship between TGR5/M-BAR and GLP-1 secretion [56]. Considering the current mechanism, TGR5/M-BAR activation increases cAMP levels and the ATP/ADP ratio, which leads to subsequent plasma membrane depolarization and Ca2+ mobilization, resulting in increased GLP-1 release (Fig.15.3) [12]. Hence, these studies suggested that GLP-1 secretion was stimulated by TGR5/M-BAR signaling in vivo and vitro. BAs and TGR5/M-BAR could become therapeutic targets of diabetes.


Fig. 15.3
TGR5/M-BAR-dependent metabolic regulation. TGR5/M-BAR activation leads to increased intracellular cAMP levels, the activation of PKA, and induction of CREB phosphorylation. This series of signaling activity induces the expression of genes bearing CRE and exists in various tissues. TGR5/M-BAR signaling induces energy expenditure in the muscle and BAT, increases GLP-1 secretion in the intestinal L cell, and reduces inflammatory cytokine release in immune cells. CRE cAMP response element, CREB-p cAMP response element-binding protein phosphorylation, Dio2 deiodinase iodothyronine type II, eNO endothelial NO, T3 triiodothyronine
15.3.2 Energy Metabolic Regulation in TGR5/M-BAR
BAs have been reported to stimulate adaptive thermogenesis and energy expenditure via TGR5/M-BAR [11]. TGR5/M-BAR activation leads to increased intracellular cAMP levels, activation of PKA, and induction of CREB phosphorylation. This series of signaling activity induces the expression of genes bearing a cAMP-responsive element (CRE) and exists in various tissues (Fig.15.3) [57, 58].
In the BAT, TGR5/M-BAR stimulation increases the intracellular cAMP level and induces cAMP-dependent iodothyronine deiodinase type 2 (Dio2) expression, which converts inactive thyroxine (T4) to active 3,5,3′-triiodothyronine (T3) to evoke increased energy expenditure [11, 59]. Dio2 increases the nuclear T3 level without various unwanted side effects caused by increased blood T3 levels. Only 20 % of nuclear T3 is produced and secreted from the human thyroid gland, and the remaining nuclear T3 is supplemented from other tissues. Dio2 supplies approximately 50 % of the T3 in the nucleus including the BAT [60]. The BAT is one of the most important targets of BAs to increase energy expenditure. Although BAT had been regarded as a tissue only in newborn infants, recent studies with FDG-PET revealed the existence of BAT around the neck and shoulders in adult humans, especially under brief cold exposure [61–63]. Furthermore, several groups have shown the importance of BAT in adult humans. In healthy patients, the amount of BAT is large and its activity is high but is reduced in obese patients [64–66]. In addition, TGR5/M-BAR and Dio2 are co-expressed in human skeletal muscle, suggesting the existence of the thermogenic mechanism in humans [11]. A human genetic study revealed the association between a single nucleotide polymorphism (SNP), rs3731859, of the TGR5/M-BAR gene and various metabolic indexes including BMI, waist circumference, intramyocellular lipid, and fasting serum GLP-1 levels [67]. Moreover, a recent study found another type of adipocyte called “beige” cells derived from the white adipose tissue. These adipocytes also respond to cyclic AMP stimulation with high uncoupling protein (UCP) 1 expression and respiration rates similar to BAT cells [68, 69]. These accumulating findings suggest a therapeutic approach to improve obesity and metabolic syndrome by increasing energy expenditure through TGR5/M-BAR stimulation.
15.3.3 Anti-atherosclerotic Effect of TGR5/M-BAR
BAs are also associated with atherosclerosis [70, 71]. Treatment with TGR5/M-BAR agonist INT-777 represses the activation of inflammatory cytokines such as NF-κB and inhibits foam cell formation and subsequent atherosclerotic plaques (Fig. 15.3). In addition, INT-777 does not inhibit atherosclerosis in TGR5/M-BAR knockout mice, supporting that the TGR5/M-BAR activation reduces atherosclerosis [70]. Furthermore, TGR5/M-BAR induces the mRNA expression of endothelial NO synthase (eNOS) and activates eNOS by phosphorylation of eNOS at amino acid position 1177 [72]. Recent study have also shown that treatment of TGR5/M-BAR agonist of taurolithocholic acid increases Akt phosphorylation and intracellular Ca2+, which induces NO production and inhibits monocyte adhesion in vascular endothelial cells. This signaling may be associated with anti-atherosclerotic effect of TGR5/M-BAR [73].
15.4 Bile Acids in Clinical Application
15.4.1 Bariatric Surgery
Bariatric surgery may provide another clue to clarify the link between BAs and glucose homeostasis. Bariatric surgery, especially gastric bypass surgery, is an established treatment for obesity and type 2 diabetes mellitus, although the mechanism of its effectiveness is still unclear. Interestingly, the improved glycemic control is observed soon after the operation when the body weight is still unchanged. Therefore, some of the antimetabolic syndrome effects of the surgical intervention are independent of body weight reduction. A recent study suggests that BAs may participate in the immediate effect of bariatric surgery. After gastric bypass, the bile flow is altered, which leads to increased plasma BA levels and incretin secretion [8]. Hormonal factors and the gut microbiota may be involved in the effect of the operation. The gut microbiota is responsible for enteral BA metabolism, and the spectrum of the gut microbiota is affected after gastrointestinal surgery. For example, the predominance of Firmicutes was reportedly mitigated, and other species including methanogens and Prevotellaceae were also suppressed after bariatric surgery [9]. In addition to these studies, recent research has revealed that FXR is associated with the effect of bariatric surgery [10]. In FXR knockout mice, metabolic improvements such as weight loss and improved glucose tolerance were reduced after bariatric surgery. Furthermore, the surgery changed the gut microbial communities differently between wild-type and FXR knockout mice. This study suggested that BAs may affect glucose homeostasis via FXR signaling and alterations of the gut microbiota after bariatric surgery.
15.4.2 Bile Acid-Binding Resins
Bile acid-binding resin (BABR) is an effective drug for the treatment of hypercholesterolemia by lowering LDL cholesterol. BABR absorbs BAs in the intestine, thereby preventing their uptake in the ileum, interrupting their enterohepatic circulation, and facilitating their excretion in the feces. The inhibition of enterohepatic circulation leads to a reduction of the BA pool size, repression of FXR-SHP and FGF15/19 signaling, and induction of CYP7A1 expression and synthesis of BAs from the cholesterol to maintain the BA pool size. A decrease in intrahepatic cholesterol levels activates SREBP-2, which induces the expression of the LDL receptor (LDLR) to enhance cholesterol uptake, reducing serum cholesterol levels. In addition to lowering the serum cholesterol effect, there is interaction between BABR and glucose metabolism [74]. In a diet-induced obesity rat model, BABR decreased serum glucose and improved glucose tolerance [75, 76]. In a clinical trial, a first-generation member of BABR cholestyramine improved glycemia by 13 % in patients with type 2 diabetes [77]. In addition, a second-generation BABR colesevelam also improved glucose clearance and increased serum GIP and GLP-1 levels in patients with type 2 diabetes mellitus [78]. These studies clarified that BABR is not absorbed in the body and there are few unwanted side effects. Furthermore, BABR can decrease blood glucose levels only in high-glucose situations and do not affect normal condition. As a result, in January 2008, the Food and Drug Administration (FDA) in the United States approved this drug as a therapeutic drug for diabetes [77, 79–82].
Although how BABR improves diabetes remains unknown, several possible mechanisms have been proposed. BABR-mediated improvement of hepatic insulin sensitivity depends on downregulating the hepatic cholesterol-LXR-IRS2 pathway [83]. In addition, BABRs induce GLP-1 secretion via the activation of TGR5/M-BAR or GPR40, each being activated by BAs binding with BABR or unabsorbed long-chain fatty acids [53, 84, 85]. Further, BABRs affect the composition of the BA pool and peripheral BAs, resulting in an induction of peripheral energy expenditure and improvement of glucose tolerance [74]. The BABR effects of improving diabetes may be explained by the inhibition of intestinal FXR as well as TGR5/M-BAR signaling [22]. BABR inhibits intestinal FXR activation and improves glucose metabolism by increasing proglucagon gene expression and inducing GLP-1 secretion in ob/ob mice [22]. These findings suggest that inhibiting FXR in the L cell via BABR could be a new target for diabetes.
15.4.3 Drug Targeting for Bile Acids Receptor
Currently, BABR has been approved by the FDA and has been clinically used as a diabetes treatment drug. The association between bariatric surgery and BAs homeostasis was confirmed. In addition to BABR and bariatric surgery, other clinical applications based on the mechanism of metabolic control via BAs signaling are ongoing. For instance, INT-747 (also named 6-ethyl-CDCA), which is a synthetic FXR agonist, exerts a hepatoprotective effect in patients of primary biliary cirrhosis (PBC) [86–88], and a phase III clinical study has already been completed and confirmed the effect of PBC. In addition to medicine, INT-747 has also entered into a study for NAFLD treatment. A phase II clinical trial for NAFLD has been completed, and an improvement was observed in type 2 diabetes mellitus patients with NAFLD. Clinical trials with TGR5 agonists, such as INT-777, are ongoing, and future studies are expected [12, 54, 89]. Altogether, these clinical applications will elucidate the BA signaling mechanisms that will lead to the improvement of metabolic disorders including obesity and diabetes.
15.5 Conclusion
Today, BAs have become important molecules to control metabolic homeostasis. We mainly discussed the relationship between BA metabolism and signal transmission, such as the FXR and TGR5/M-BAR pathways and the possibility that BAs may improve metabolic diseases. Current evidence shows that BAs regulate lipid, glucose, and energy metabolism via FXR or TGR/M-BAR-mediated pathways. Furthermore, the clinical application of FXR and TGR/M-BAR agonists is ongoing.
Recent studies have focused on intestinal FXR signaling; however, conflicting data have been reported regarding the metabolic regulation of intestinal FXR activity. Further studies are necessary to determine intestinal FXR signaling taking into consideration various factors such as microbiota regulation, BA pool size, and BAs composition.
References
1.
Hofmann AF, Borgstroem B. The intraluminal phase of fat digestion in man: the lipid content of the micellar and oil phases of intestinal content obtained during fat digestion and absorption. J Clin Invest. 1964;43:247–57. doi:10.1172/jci104909.CrossRefPubMedPubMedCentral
2.
Urizar NL, Dowhan DH, Moore DD. The farnesoid X-activated receptor mediates bile acid activation of phospholipid transfer protein gene expression. J Biol Chem. 2000;275(50):39313–7. doi:10.1074/jbc.M007998200.CrossRefPubMed
3.
4.
5.
6.
Forman BM, Goode E, Chen J, Oro AE, Bradley DJ, Perlmann T, et al. Identification of a nuclear receptor that is activated by farnesol metabolites. Cell. 1995;81(5):687–93. doi:10.1016/0092-8674(95)90530-8.CrossRefPubMed
7.
Watanabe M, Houten SM, Wang L, Moschetta A, Mangelsdorf DJ, Heyman RA, et al. Bile acids lower triglyceride levels via a pathway involving FXR, SHP, and SREBP-1c. J Clin Invest. 2004;113(10):1408–18. doi:10.1172/JCI21025.CrossRefPubMedPubMedCentral
8.
Nakatani H, Kasama K, Oshiro T, Watanabe M, Hirose H, Itoh H. Serum bile acid along with plasma incretins and serum high-molecular weight adiponectin levels are increased after bariatric surgery. Metab: Clin Exp. 2009;58(10):1400–7. doi:10.1016/j.metabol.2009.05.006.CrossRef
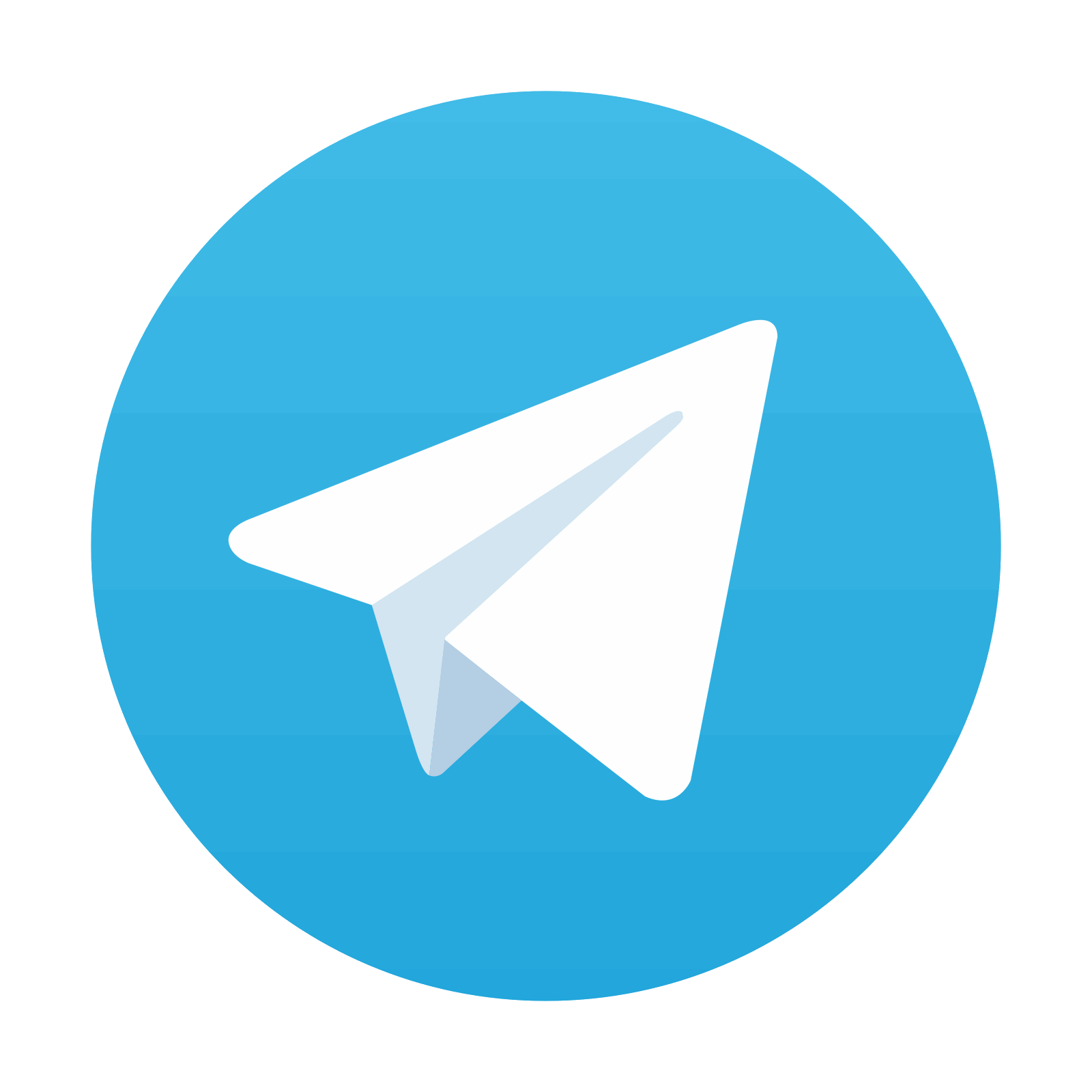
Stay updated, free articles. Join our Telegram channel

Full access? Get Clinical Tree
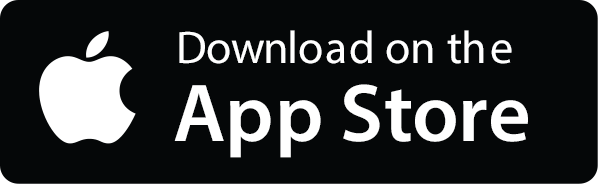
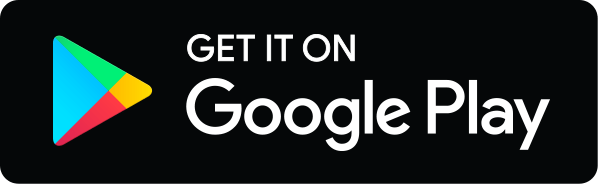