Fig. 10.1
Bile acid receptors (FXR, TGR5) and metabolic regulation in the liver. FXR is expressed in hepatocytes in the liver. TGR5 is expressed in LSECs and Kupffer cells in the liver, but not hepatocytes. The ability of different BAs to activate FXR occurs in the following order CDCA>LCA=DCA>CA. TGR5 can be activated by a variety of BAs in the order LCA>DCA>CDCA>CA. FXR farnesoid X receptor, TGR5 G-protein-coupled receptor 1, LSECs liver sinusoidal endothelial cells
10.2.1 Role of FXR in NAFLD and NASH
Both conjugated and unconjugated BAs can activate FXR, and thus BAs may serve as endocrine hormones to regulate metabolism via FXR [14–16]. The ability of different bile acids to activate FXR-α occurs in the following order CDCA>LCA=DCA>CA [17]. FXR is an important regulator of lipid and glucose metabolism as well as inflammatory response. Activated FXR improves lipid and glucose homeostasis and inhibits inflammation.
FXR-null mice developed severe fatty liver, and elevated circulating free fatty acids, which was associated with elevated serum glucose and impaired glucose and insulin tolerance [18].
Inflammation plays an important role in the pathogenesis of NAFLD. Fxr −/− mice display elevated levels of inflammation and develop spontaneous HCC at age of 9–12 months [19]. Diabetes/insulin resistance facilitates the progression HCC when FXR is deficient [20]. The increased levels of inflammation observed in Fxr −/− mice may partly be explained by elevated BAs levels in these mice.
The findings that FXR plays an important role in regulating both lipid homeostasis and inflammation suggest that FXR may modulate the progression of NAFLD. Indeed, Fxr −/− mice have increased hepatic TG accumulation. FXR deficiency causes pathologic manifestations of NASH in low-density lipoprotein receptor knockout mice (Ldlr −/− mice) after a high-fat feeding; the liver of these mice displayed massive steatosis and inflammatory infiltration [21].
FXR also regulates hepatic fibrosis. FXR is expressed at very low levels on hepatic Kupffer cells, stellate cells, and sinusoidal endothelial cells. FXR activation with 6-ethyl chenodeoxycholic acid (6-ECDCA), an FXR ligand, prevents liver fibrosis in porcine serum-treated rats or rats that underwent bile duct ligation and decreases expression of matrix proteins, including α1-collagen, transforming growth factor β-1 (TGF-β-1), alpha smooth muscle actin (αSMA), and tissue inhibitors of matrix metalloproteinase (MMP) 1 and 2 [22]. Recently, FXR activation has been shown to increase the expression of the microRNA mir29a, which regulates the expression of several extracellular matrix proteins in hepatic stellate cells [23].
It has been reported that in the NAFLD patients, the hepatic expression of FXR is significantly decreased. In this study, the expression of two other nuclear receptors, liver X receptor (LXR) and sterol regulatory element-binding protein 1C (SREBP-1C) that are closely related to fat metabolism and its regulation, was induced [24]. Thus, the reduced FXR expression may play a role in the pathogenesis of human NAFLD.
Interestingly, in two animal models of NASH, serum levels of tauro-β-muricholic and taurocholic acid were increased [25]. Hepatic expression of transporters of bile acids from the circulation to the liver (Slc10a1/Slco1a1) was decreased, whereas those transporters that transfer bile acids to the blood (Abcc1/4) were increased. It has been therefore discussed that if bile acid content is decreased in the liver, vicious circle may aggravate NASH [26], because decreased ligand activation of FXR could lead to triglyceride accumulation and inflammation in the liver.
Another study on 113 NAFLD patients reveals a close association between BA synthesis and plasma BA concentration and the severity of NAFLD [15]. In these patients, both the CYP7A1 and the bile acid transporter Na+/taurocholate cotransporting polypeptide (NTCP) expression are increased, likely as a result of increased FFA levels [27].
10.2.2 Role of TGR5 (GPBAR1) in NAFLD and NASH
TGR5 is a member of the rhodopsin-like superfamily of G-protein-coupled plasma membrane receptor for BAs, which mediates many of the rapid and non-genomic actions of BAs. TGR5 has been implicated in the control of glucose homeostasis, inflammation, and liver functions. Like FXR, it is originally regarded as an orphan receptor without known ligands [14, 28]. Recently, it has been known that TGR5 can be activated by a variety of bile acids in the order LCA>DCA>CDCA>CA [29].
Activation of TGR5 by bile acids stimulated adenylate cyclase, rapid intracellular cAMP production, and protein kinase A activation. TGR5 plays an important role in BA homeostasis as well as in glucose/lipid homeostasis and energy expenditure. It regulates the expression of genes involved in inflammation, modulates plasma glucose and lipid levels, and increases energy expenditure in skeletal muscle and brown adipose tissue [28, 30–32].
TGR5 is highly expressed along the intestinal tract such as the ileum and colon, which are exposed to high levels of bile acids [29]. It has been shown that bile acid-activated TGR5 stimulated the production of glucagon-like peptide-1 (GLP-1), which promotes insulin secretion and regulates glucose homeostasis, in an enteroendocrine cell line [33]. Despite that the liver is a major bile acid target organ, TGR5 expression in the liver is low. In the liver, TGR5 is expressed in sinusoidal endothelial cells and Kupffer cells, but not in hepatocytes [28, 34].
TGR5 is also expressed in monocytes and macrophages, and in human spleen, suggesting that it plays an anti-inflammatory role in the immune system. Indeed, TGR5 activation has shown protective effects in various inflammation-related diseases in experimental models [29, 35]. Kupffer cells are capable of secreting proinflammatory cytokines, which can contribute to the progression of NAFLD [36]. When Kupffer cells were treated with the synthetic TGR5 agonist INT-777, there was a reduction in the lipopolysaccharide (LPS)-induced production of inflammatory cytokines through the TGR5-cAMP-dependent pathway. INT-777 treatment attenuates the expression of inflammatory mediators by antagonizing NF-κB activity in wild-type mice but not in Tgr5 −/− mice [37]. The anti-inflammatory and anti-steatotic properties of TGR5 suggest that TGR5 may protect against the development and progression of NAFLD [38, 39].
10.2.3 Role of Sphingosine-1-Phosphate Receptor 2 in NAFLD and NASH
Sphingosine-1-phosphate (S1P) is a potent bioactive sphingolipid that is involved in a variety of cellular processes, including cell proliferation, differentiation, motility, angiogenesis, inflammation, and malignant transformation [40]. Intracellular S1P is synthesized from sphingosine by sphingosine kinase 1 (SphK1).
Intracellular S1P can directly activate various cellular signaling pathways or be exported out of cells by specific transporters (spinster homologue 2; Spns2) in the cell membrane [41]. Extracellular S1P exerts its function via activating five different G-protein-coupled receptors (S1PR1–5) on the cell membrane to induce various cellular responses [42]. S1PR1 is ubiquitously expressed and plays a key role in angiogenesis, vascular maturation, and immune cell trafficking. Deletion of S1PR1 affects maturation and is embryonically lethal.
Unlike S1PR1, mice deficient in S1PR2 exhibit no phenotypic defects, but develop spontaneous and sporadic seizures [43]. Studies in S1pr2 −/− mouse have also shown S1PR2 to be responsible for proper development of the auditory and vestibular systems [44].
S1PR2 is highly expressed in the liver and plays a unique and critical role in the pathophysiology of the liver. The role of S1PR2 in bile acid-mediated hepatic lipid metabolism was identified in recent studies [45]. In primary rodent hepatocytes, conjugated bile acids activate S1PR2, which further activates the downstream ERK1/ERK2 and AKT signaling pathways [45]. Bile acid-mediated activation of ERK1/ERK2 and AKT signaling pathway plays an important role in the regulation of hepatic glucose and lipid metabolism [46, 47]. In primary rat hepatocytes, insulin and bile acids both activated glycogen synthase activity to a similar extent.
Infusion of taurocholate (TCA) into the chronic bile fistula rat rapidly activated the AKT and ERK1/ERK2 signaling pathway and glycogen synthase activity [48]. In addition, TCA induced a rapid downregulation of the gluconeogenic genes, PEP carboxykinase (Pepck) and glucose-6-phophatase (G-6Pase), and a marked upregulation of small heterodimeric partner (SHP) mRNA in the livers [47]. These results suggest that TCA has insulin-like activity to regulate hepatic glucose metabolism both in vitro and in vivo. A recent study reported that S1pr2 null mice rapidly develop overt fatty livers on a high-fat diet compared to wild-type mice, suggesting that S1PR2 is an important regulator of hepatic lipid metabolism [49].
10.3 Bile Acids and Gut Microbiota in NAFLD and NASH
BAs and gut microbiota are closely linked through the enterohepatic circulation, which plays a role in communication between the liver and intestine. BAs are produced from cholesterol in the liver, conjugated to amino acid glycine or taurine, and secreted into the small intestine. Conjugated BAs are absorbed in the terminal ileum to return to the liver.
BAs secreted from the liver inhibit gut microbial growth through their detergent property. Gut bacteria also regulate bile acid biotransformation in the intestine, which alters bile acid composition generating secondary bile acids by deconjugation and dehydroxylation (Fig. 10.2). Recent evidence indicates that there is a regulatory relationship between the development of obesity and altered gut microbiota, suggesting that the microbiota can induce NAFLD or its progression toward overt nonalcoholic steatohepatitis [50].


Fig. 10.2
Bile acid and gut microbiota. BAs regulate gut bacteria overgrowth and protect against inflammation. BAs secreted from liver inhibit gut microbial growth through their detergent property. Gut bacteria also regulate bile acid biotransformation in the intestine, which alters bile acid composition generating secondary bile acids by deconjugation and dehydroxylation. High-fat diets and drugs alter bile acid biotransformation and gut microbiota and contribute to pathogenesis of liver-related metabolic diseases
A number of studies showed that germ-free mice were protected against diet-induced obesity compared with the conventionally raised counterparts [51, 52]. Interestingly, germ-free mice receiving cecal microbiota from ob/ob mice had higher energy absorption from food and more weight gain than germ-free mice harboring cecal microbiota from lean mice [52].
Dysbiosis is the major environmental factor, which affects bile acid metabolism, and contributes to diseases not only in the gastrointestinal system but also in chronic diseases including NAFLD, diabetes, and obesity [53]. Treating dysbiosis by modulating bile acid metabolism by gut microbiota may be a therapeutic approach for improving and preventing NAFLD.
10.4 Therapeutic Potential of BAs and Derivatives in NAFLD and NASH
Many reports have described that activation of FXR inhibits inflammation in the liver and intestine, and FXR agonists are potential therapeutic drugs for metabolic and inflammatory diseases. Obeticholic acid (OCA, 6-ethyl-CDCA or INT-747) is a synthetic derivative of chenodeoxycholic acid and a highly potent and selective FXR agonist that has anticholestatic effects [54].
In experimental studies, OCA increases insulin sensitivity, inhibits gluconeogenesis, inhibits lipogenesis, and has anti-inflammatory and antifibrotic properties [55]. OCA ameliorates high-fat diet-induced obesity and insulin resistance in mice, as well as insulin resistance and fatty liver in Zucker rats (fa/fa) [56]. OCA antagonizes NF-kB-stimulated inflammation in the liver [57], modulates innate immunity in animal models of colitis [58], and inhibits and preserves the intestinal barrier in inflammatory bowel disease [59]. The phase II clinical trial of OCA for NAFLD and T2DM patients showed improved insulin sensitivity, reduced γ-glutamyl-transpeptidase levels (a marker of NASH), and weight loss [60]. Recently, the multicenter, randomized, placebo-controlled trial (FLINT), which was conducted by the NIDDK NASH Clinical Research Network, revealed that OCA improved the histological features of NASH [61].
Since TGR5 signaling inhibits the production of proinflammatory cytokines, drugs targeting to TGR5 also have the potential to treat NAFLD or NASH. A bile acid derivative INT-777 is a selective and potent TGR5 agonist [62, 63]. In animal studies, INT-777 improves glucose tolerance, stimulates GLP-1 secretion from enteroendocrine L cells, and improves insulin sensitivity [31]. TGR5 agonists also reduce and prevent inflammation in the liver [28]. Recently, the FXR and TGR5 dual agonist INT-767 (6α-ethyl-3α,7α,23-trihydroxy-24-nor-5β-cholan-23-sulfate) has been shown to improve NAFLD by modulating hepatic monocyte activity [38].
10.5 Future Perspectives in NAFLD and NASH
Recent research advances in bile acid metabolism and signaling enabled us to understand an important role for bile acids in integration of hepatic lipid, glucose, and energy metabolism. Among them, findings of bile acid receptors have largely contributed to progress in translation of basic research in bile acid metabolism to clinical applications for drug therapies of NAFLD and NASH.
Results of FLINT trial, showing OCA being effective in histological improvement in NASH, are the great first steps of therapeutic strategies, but appear to require further analysis in terms of histological reversibility and long-term follow-up for hepatocellular carcinoma. It is anticipated that new treatment strategies, including nonbile acid-based agonists specific for FXR and TGR5, will be developed for treating NAFLD and NASH.
References
1.
Postic C, Girard J. Contribution of de novo fatty acid synthesis to hepatic steatosis and insulin resistance: lessons from genetically engineered mice. J Clin Invest. 2008;118:829–38.CrossRefPubMedPubMedCentral
2.
Cohen JC, Horton JD, Hobbs HH. Human fatty liver disease: old questions and new insights. Science. 2011;332:1519–23.CrossRefPubMedPubMedCentral
3.
4.
Larter CZ, Farrell GC. Insulin resistance, adiponectin, cytokines in NASH: which is the best target to treat? J Hepatol. 2006;44:253–61.CrossRefPubMed
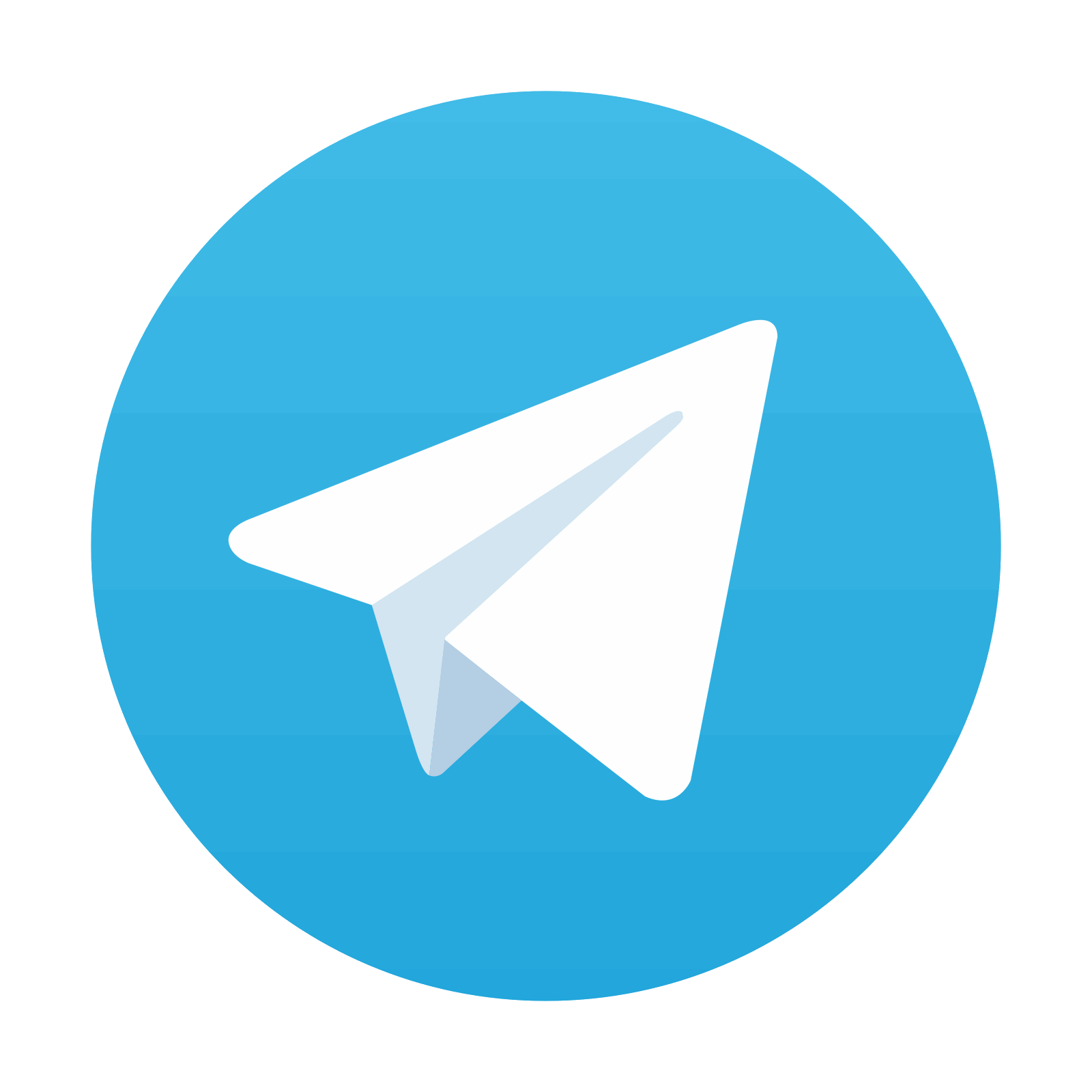
Stay updated, free articles. Join our Telegram channel

Full access? Get Clinical Tree
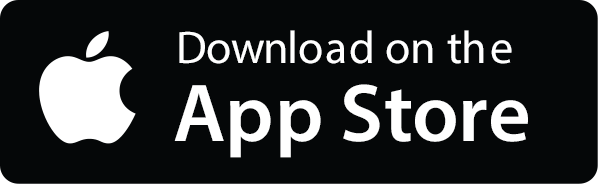
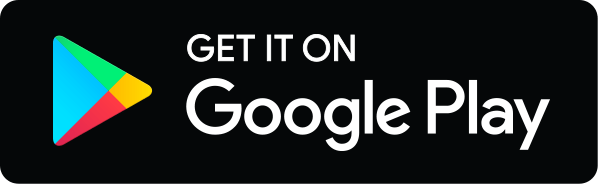