Fig. 15.1
General mechanisms causing diarrhea. Bacterial toxins can affect intestinal epithelial functions through different mechanisms. Chloride secretion and water secretion depend on the altered functionality of CFTR, CaCC, and NHE channels. Alterations in TJ structures alter the electric gradient causing the movement of both ions and water. Pathogen bacteria induce inflammation through the recruitment of immunity cells and the upregulation of pro-inflammatory cytokines. Finally, an altered gut microbiome composition (dysbiosis) supports pathogen infections. CFTR cystic fibrosis transmembrane conductance regulator, CaCC calcium-activated chloride channels, NHE Na+, H+-exchanger, TNFα tumor necrosis factor-α, IL interleukin
Intestinal Ion Transport and Barrier Functions
In normal conditions, fluid transport across the intestinal epithelial cells is a finely balanced process with fluid absorption predominating on fluid secretion. Electrolyte absorption by apical enterocyte depends on the electroneutral and the electrogenic absorption [2], which involves Na+ uptake by the Na+, H+-exchanger (NHE) and Cl− uptake by the Cl−, HCO3 2− exchangers. There are several exchangers: NHE1 is located on the basolateral surface of the enterocyte, whereas NHE2 and NHE3 have an apical localization. The relative contribution of NHE2 and NHE3 depends on their localization within the intestine with NHE3 being the main mediator of electroneutral Na+ uptake [3]. The electrogenic ion absorption is the second major source of apical ion absorption through specific channels. Epithelial sodium channel (ENaC) is the most prominent apical Na+ channel in the colon and allows Na+ influx into the cell along its electrochemical gradient [3].
A basal level of fluid secretion is necessary for accomplishing the nutrient digestive functions. The cyclic AMP-dependent chloride channel defined as the cystic fibrosis transmembrane conductance regulator or CFTR is located on the brush border. This channel is responsible for water secretion in basal conditions and under active stimulation by secretagogues [4]. In the intestine , water secretion is a passive process driven by active ion secretion, predominantly by Cl− secretion [5]. Chloride is taken up across the basolateral membrane via Na+/K+/2Cl− cotransporter type 1 (NKCC1), as an electroneutral process. Chloride accumulation is a passive process driven by Na+ concentration gradient which is maintained by the basolateral Na, K-ATPase. Two distinct potassium channels are located into the basolateral membrane allowing for potassium recycling thus preventing cellular depolarization, ultimately preserving the electrical driving force for chloride exit from the cell. Therefore, chloride accumulates until apical chloride channels are opened. The bulk of chloride output occurs via cyclic adenosine monophosphate (cAMP)-dependent CFTR chloride channel. However, there is an additional class of chloride channels, the calcium-activated chloride channels (CaCC), that are expressed in the enterocyte apical membrane. These channels drive chloride secretion in response to agonists of cytosolic calcium [4]. Three main intracellular signal-transduction pathways regulate water and electrolyte fluxes across the intestinal mucosa: cAMP; cyclic guanosine monophosphate (cGMP); and calcium-dependent pathways. Recently, a fourth pathway involving nitric oxide (NO) has also been described. Physiological cAMP and cGMP concentrations activate CFTR resulting in a mild secretion of chloride, whereas abnormal CFTR activation in crypt cells results in the secretory diarrhea. cGMP generally results in a more potent, though shorter, chloride secretion than that induced by cAMP [6].
The most common mechanism of bacterial diarrhea is the release of bacterial toxins. Bacterial enterotoxins activate signaling molecules such as cyclic AMP, cyclic GMP, or intracellular Ca2+, which, in turn, open cellular Cl– channels leading to an increase in Cl– secretion and consequently of water [5]. The intestinal epithelium forms a selective barrier between the lumen (the external environment) and the body. The electrochemical gradients also depend on TJ that provide a barrier necessary for an efficient transcellular transport. The TJ also support a polarized distribution of membrane proteins on the basal and apical compartments of epithelial cells [7]. The plasma membranes of adjacent intestinal epithelial cells are linked through the TJ, where claudins, zonula occludens 1 (ZO1), occludin, and F-actin interact. E-cadherin, alpha-catenin 1, beta-catenin, catenin delta1, and F-actin interact to form the adherens junction [8]. The loss of barrier function as a consequence of TJ disruption impairs the vectorial absorption and secretion by the intestinal epithelium. During an infection, the redistribution of apical and basal proteins provides new attachment sites for bacteria. Several bacterial toxins act on the TJ protein functions inducing an epithelial damage. At least four toxins produced by Clostridium spp. and a toxin produced by selected E. coli strains have been demonstrated to affect intestinal TJ [9, 10].
In this complex scenario, different bacterial species, each with one or more virulence factors, induce diarrhea in a specific mode. This includes the interaction with specific receptor, the release of virulence factors such as adhesion molecules and enterotoxins and a cascade of events occurring within the enterocyte.
Bacterial Diarrhea
Vibrio Cholerae
This pathogen is easily identified in the stools using Gram stain and is responsible for cholera, a potentially fatal diarrheal disease in humans. Although cholera is now rare in developed countries, it remains a major cause of diarrheal morbidity and mortality in several developing countries. Indeed, with the occurrence of calamities, the spreading of cholera infection in overcrowded refugee camps is a potential significant threat everywhere. The infection is transmitted by the fecal–oral route and is spread through contaminated food and water, the period of incubation ranges from few hours to 5 days. The vast majority of infected subjects remain asymptomatic or experience a mild disease with watery stools, nausea or vomiting, and no significant dehydration. However, cholera may be severe in a number of subjects. Stools are classically described as “rice water” due to the presence of mucus in clear stools. Profuse watery diarrhea and vomiting lead to massive fluid and electrolyte losses that can occur at a rate of 1 l/h, causing a massive dehydration.
Virtually, all cases of non-O1 Vibrio infections in the USA are associated with eating raw shellfish and gastroenteritis ranges from a mild illness to profuse, watery diarrhea comparable to that seen in epidemic cholera. Diarrhea, abdominal cramps, and fever are the most common symptoms with nausea , vomiting, and bloody stools occurring less frequently. As with V. cholerae O1, the mainstay of therapy for diarrheal disease is oral rehydration [11, 12]. In cases of septicemia (which typically occurs in immunocompromised patients), supportive care and correction of shock are essential interventions associated with antibiotics (tetracycline). V. cholerae secretes several toxins, but the most important of these is cholera toxin (CT). CT consists in a single copy of the A subunit and five copies of the B subunit. B subunit binds to plasma membrane, while the A subunit activates adenylate cyclase resulting in elevated cAMP production. The production of cAMP activates the protein kinase A (PKA), which then phosphorylates the regulatory domain of CFTR [5, 13]. In addition to increased Cl− secretion, absorption of Na+ is decreased through a cAMP-dependent mechanism in which the activity of both apical sodium transporters, NHE2 and NHE3, is decreased. This leads to an increase in NaCl levels in the intestinal lumen and drives water by osmotic force [14].
In addition to CT, V. cholerae produces other toxins that modulate ion secretion and alter barrier function to cause massive diarrhea. The toxins that directly affect ion secretion include the accessory CT (ACE), which stimulates Ca2+-dependent Cl− secretion, the non-agglutinable Vibrio cholerae heat-stable enterotoxin (NAG-ST), which activates guanylyl cyclase, thus stimulating cGMP production, leading to protein kinase G (PKG)-mediated activation of CFTR and, finally, the V. cholerae cytolysin (VCC), which creates anion permeable pores into the cell wall [15].
Salmonella
Salmonella typhi and Salmonella paratyphi are Gram-negative, motile bacilli that colonize only humans. Therefore, the infection is acquired through close personal contact or through the ingestion of water or food contaminated with human feces. Typhoid fever continues to represent a global health problem, with more than 12.5 million cases/year [16]. After an incubation period ranging between 5 and 21 days, these bacteria cause a systemic illness characterized by fever, gastrointestinal symptoms, and occasionally neurological symptoms [17]. Chills, headache, cough, weakness, and muscle pain are frequent prodromes and most symptoms resolve within 4 weeks without antimicrobial treatment. However, Salmonella may spread and invade the bloodstream and extraintestinal districts causing a systemic disease defined as enteric fever. In this case, patients relapse with high fever, abdominal pain from inflammation of Peyer’s patches , and intestinal microperforation followed by secondary bacteremia with intestinal agents.
In contrast to S. typhi, infections with non-typhoidal salmonellae are increasing in developed countries. Patients at higher risk for infection include those with immunodeficiencies, age younger than 3 months, alterations in intestinal defenses (e.g., on those antacid treatment), impaired reticuloendothelial function (sickle cell and hemolytic anemia), and ingestion of antibiotics to which the organism is resistant. Reservoirs include a wide range of domestic and wild animals, including poultry, swine, cattle, rodents, and reptiles. Salmonella enteritidis is the leading reported cause of food—borne diarrheal outbreaks in the USA, with eggs and contaminated raw fruits and vegetables identified as major vehicles [18].
The incubation period is 6–45 h, after which fever, headache, vomiting, abdominal pain, and watery stools (which may contain blood, mucus, and leukocytes) appear, lasting from locus to few days. Severe extraintestinal infections can range from life-threatening sepsis to focal infections in the meninges, bones, and lungs. The microorganism is easily isolated from fresh stools or blood culture.
Clostridium Difficile
Clostridium difficile (CD), a Gram-positive anaerobe that forms spores (hence difficult to clear from hospital setting), is now recognized as the most common agent of nosocomial diarrhea [19]. In the past decade, a dramatic increase in the incidence of C. difficile infections (CDI) has been reported worldwide, possibly linked with an excess of antibiotics and other treatment responsible for microflora disruption but also linked with emergence of hypervirulent strains (e.g., NAP1/BI/027) and increased numbers of highly susceptible individuals.
Antibiotic treatment predisposes patients to C. difficile-associated disease in specific conditions [20]. The pathogenesis of C. difficile infection largely depends on the altered balance of the intestinal microbiota, allowing pathogenic strains of C. difficile to infect the intestine [21].
C. difficile causes a broad spectrum of intestinal diseases ranging from mild diarrhea to potentially fatal pseudomembranous colitis (PMC). C. difficile is a major agent of antibiotic-associated colitis [22] and produces three toxins, toxin A (TxA), toxin B (TxB), and a binary toxin (cytolethal distending toxin, CDT) [23]. Both TxA and TxB exert a cytotoxic effect in vitro [24, 25]. In particular, TxA and TxB induce the disaggregation of the actin cytoskeleton, cell rounding, cell death, and loss of intestinal epithelium barrier function leading to increased intestinal permeability and diarrhea [26]. CDT induces a redistribution of microtubules and the formation of long microtubule-based protrusions at the surface of intestinal epithelial cells [9] enhancing adherence and colonization of Clostridia [25, 27].
However, diarrhea is essentially due to a necroinflammatory reaction: C. difficile toxins trigger an extensive inflammatory cascade causing a rapidly progressive damage to host tissues resulting in fluid exudation [28]. In parallel, selected cytokines are also able to induce the activation of enteric nerves and induce Cl− secretion. The onset of symptoms may begin from several days after antibiotic therapy is started until 2 months following cessation of treatment. Diarrhea and abdominal cramps are usually the presenting symptoms, followed by fever and chills in severe cases. Mild colitis, with bloody stools and mucus, particularly if they follow antibiotic treatment, should be considered potentially induced by C. difficile infection. Microbial culture, latex agglutination, tissue culture assay, and enzyme-linked immunosorbent assay (ELISA) are all used for the diagnosis of C. difficile infection.
However, the role of C.difficile in infancy and early childhood is still a matter of debate. This is an emerging agent of diarrhea whose role is limited or questionable in children below 36 months of age [29, 30]. It is also a major agent of antibiotic-induced diarrhea and of severe diarrhea in children with underlying chronic conditions such as inflammatory bowel diseases (IBD) [31].
Asymptomatic C. difficile colonization is common in infants (ranging from 2 to 75 % of healthy children) until 3 years of age and related to delivery mode and feeding [32]. Strains that usually are associated with diarrhea in adults may be detected in asymptomatic infants, and children may be a reservoir of pathogenic strains.
Many healthy children excrete C. difficile in their stools during early infancy, and rates as high as 45 % have been detected in infants attending day care nurseries and as many as 13 % were harboring toxigenic isolates [33].
Independently from the prevalence of carriers and the method to search for C. difficile, the incidence of C. difficile-related diseases in children has progressively increased in the last years, in parallel with data reported in adults, but with a relative more limited clinical impact. A recent 18-year cohort study reported a 12-fold increase of C. difficile infection incidence in children. Overall C. difficile infections increased from 2.6 (1991–1997) to 32.6 per 100,000 persons (2004–2009), with a prevalence of community acquired infection that increased from 2.2 to 23.4 per 100,000 in the same period [34]. However, a role of C. difficile as a severe pathogen in children at risk is supported by a solid data: For this reason, one potential alternative to standard therapy is the use of indigenous intestinal microorganisms from a healthy donor to restore the intestinal microbiota of infected patients [35, 36].
In children with chronic diseases such as IBD , C. difficile may act as severe opportunistic pathogen and trigger burst of the disease with a potentially severe course.
Shigella
Shigella causes 250 million cases of diarrhea and 650,000 deaths worldwide per year. Shigella is a Gram negative, non-lactose fermenting, nonmotile bacillus. Shigella sonnei is the main type in industrialized countries, and S. flexneri and S. dysenteriae predominating in developing countries [37]. The latter is the most dangerous species as it produces Shiga toxin, which can lead to hemolytic uremic syndrome (HUS). Humans are the only natural hosts and transmission occurs by fecal–oral contact. The very low infective load (as few as ten organisms) makes Shigella highly contagious. Shigella causes a dysenteric diarrhea, and the cellular responses to various steps of the invasion process are the primary cause of inflammation. Shigella strains cross the epithelial barrier through the M-cells where they bind to basolateral TLR4 receptor. This causes the production of interleukin (IL)-6, IL-8, and the subsequent release of IL-1β, which attracts PMN cells [38]. Nuclear factor kappa B (NF-κB) and mitogen-activated protein kinase (MAPK)-signaling pathways are activated not only in Shigella-infected epithelial cells but also in uninfected bystander cells [39], and this cell–cell communication amplifies inflammation. However, Shigella extensively disrupts TJs. In polarized cultured T84 epithelial cells, ZO-1, claudin-1, and the phosphorylation status of occludin were all affected by S. flexneri [40]. There is a secondary mechanism that promotes diarrhea, triggered by serine protease autotransporters of Enterobacteriaceae (SPATES), three enterotoxins that alter transepithelial fluid movement and cytoskeletal structure [41]. After 1–4 days of incubation, shigellosis begins with fever, headache, malaise, anorexia, and occasional vomiting and watery diarrhea with progression to dysentery within hours to days. Unusual extraintestinal manifestations may occur, including HUS in children and thrombotic thrombocytopenic purpura in adults. Most episodes of shigellosis in otherwise healthy individuals resolve within 7 days. Shigellae are difficult to grow and are best isolated from fresh stools rapidly inoculated into selective culture plates incubated immediately at 37 °C.
Campylobacter jejuni
These organisms are small, spiral-shaped Gram-negative bacilli that live in the intestines of both wild and domestic animals [42]. Common vehicles for human infection are poultry, unpasteurized milk, and contaminated water [43, 44]. After 3–6 days of incubation, symptoms abruptly begin. Campylobacter diarrhea may present as a typical gastroenteritis with vomiting and profuse diarrhea or as a colitis with abdominal pain and bloody stools, and abdominal pain that may mimic an appendicitis. Diarrhea usually lasts 4–5 days; the microorganism can be identified only from stool. Campylobacter vaccine development has proceeded cautiously, because of concerns about postexposure arthritis or Guillain–Barré syndrome. However, a monovalent, formalin-inactivated, C. jejuni whole-cell vaccine with a mucosal adjuvant has entered human trials.
Yersinia
Yersinia enterocolitica and Y. pseudotuberculosis are two important human enteropathogens widely distributed in the environment , with swine as the major reservoir. The incubation period is 3–7 days, with food-borne transmission. Yersinia’s preference for cool temperatures makes this pathogen more common in Northern Europe, Scandinavia, Canada, the USA, and Japan. Yersinia enterocolitis occurs more often in children younger than 5 years [45] and is characterized by fever, vomiting, exudative pharyngitis, cervical adenitis, abdominal pain, and watery diarrhea, which may contain blood [46, 47]. Diarrhea typically lasts for 14–22 days, but fecal excretion may persist for 7 weeks or longer. Abdominal complications include appendicitis , pseudoappendicitis, diffuse ulcerations of the intestine and colon, intestinal perforation, peritonitis , ileocecal intussusception , toxic megacolon, cholangitis, and mesenteric vein thrombosis. Bacteremic spread may result in abscess formation and granulomatous lesions in the liver, spleen, lungs, kidneys, and bone, as well as meningitis and septic arthritis. Yersinia infection can also be associated with extraintestinal sequelae including reactive arthritis, uveitis, Reiter’s syndrome, and erythema nodosum [48]. Yersinia may be isolated from stools or pharyngeal exudates on commonly used selective media, and appears as Gram-negative colonies after 2–14 days of growth at 25–28 °C.
E. coli
E. coli is the most abundant facultative anaerobe of the human colonic flora and typically colonizes the gastrointestinal tract within few hours after birth. E. coli is a Gram-negative, lactose-fermenting motile bacillus of the family Enterobacteriaceae. Currently, 171 somatic (O) and 56 flagellar (H) antigens are recognized. E. coli includes a heterogeneous group of microorganisms capable to exert various possible interactions with the host, ranging from a role of mere harmless presence to that of a highly pathogenic organism [49]. Six distinct categories of E. coli are currently recognized as pathogens: enterotoxigenic E. coli (ETEC), enteropathogenic E. coli (EPEC), enterohemorrhagic E. coli (EHEC), diffusely adherent E. coli (DAEC), enteroaggregative E. coli (EAEC or EAggEC), and enteroinvasive E. coli (EIEC).
ETEC
ETEC strains elaborate two classes of enterotoxins, namely the heat-labile and the heat-stable enterotoxins (LT and ST, respectively) [50]. LT, the heat-labile enterotoxin, is the analogue of CT and induces chloride secretion by increasing the intracellular cAMP levels. ST, the heat-stable enterotoxin, is a small peptide that causes an increase of intracellular cGMP to induce chloride secretion and diarrhea [51]. The jejunum is a major target of ST-induced anion secretion that is mediated by CFTR [27]. ST binds to its receptor guanylate cyclase C (GCC) on the apical surface of enterocytes, resulting in the generation of cGMP. This in turn activates a cGMP-dependent kinase (cGKII) leading CFTR phosphorylation [50] and the consequent inhibition of Na+ absorption through the apical membrane of jejunal enterocytes [48]. ST does this by acting on both the apical and the basolateral side of intestinal epithelium, providing an example of a molecular mimicry between ST and its endogenous ligand guanylin, an endogenous analogue found both in the intestinal lumen and in the blood that regulates two distinct processes, ion absorption and cell proliferation [52, 53]. Transmission of E. coli occurs by ingestion of contaminated food and water, with peaks during the warm, wet season. Like EPEC, ETEC requires a relatively high inoculum and has a short incubation period (14–30 h). The key symptom is watery diarrhea, sometimes with fever, abdominal cramps, and vomiting. In its most severe form, ETEC can cause cholera-like severe diarrhea and dehydration. The illness is generally self-limited, lasting less than 5 days. Infection with ETEC has also been associated with short- and long-term nutritional consequences in infants and children.
EPEC
This was the first group of E. coli serotype shown to be pathogenic for humans and responsible for devastating outbreaks of nosocomial neonatal diarrhea and infant diarrhea in virtually every corner of the globe. EPEC is characterized by specific serogroups, the most common being E. coli O145, O49, and O157. Some of those strains have additional virulence factors. EPEC strains are peculiar in their ability to induce a characteristic attaching and effacing lesion in the small-intestinal enterocytes and by their inability to produce Shiga toxins.
However, EPEC encode a T3SS and produces a characteristic attaching and effacing (A/E) lesion which is characterized by effacement of microvilli on the epithelial surface at the site of bacterial attachment. This typical lesion is responsible for the loss of absorptive surface and osmotic diarrhea [54]. Intestinal pathogens can disrupt the barrier by directly altering the distribution or phosphorylation status of TJ proteins . EPEC strains alter the phosphorylation status and distribution of TJ proteins, occludin and claudin-1, thereby disrupting the epithelial barrier function [10]. This increases paracellular permeability driving ion and water into the lumen upon electrochemical gradients ultimately causing diarrhea. The link between barrier disruption and diarrhea derives from studies involving the pro-inflammatory cytokine, tumor necrosis factor-α (TNFα) [55, 56]. These observations suggest that EPEC-induced diarrhea is a multifactorial process with alterations of electrolyte, solute, and water transport. EPEC causes a self-limited watery diarrhea with a short incubation period (6–48 h). This may be associated with fever, abdominal cramps, and vomiting, and EPEC is a leading cause of persistent diarrhea (lasting 14 days) in children in developing countries [57].
The microbiological diagnosis of EPEC-induced disease is performed with analytic methodologies different from those used by the standard microbiology laboratory, the most relevant being: (a) serotyping, (b) adherence assay, (c) FAS test, and (d) the specific detection of virulence-involved genes (bfpA and eae genes) using molecular biology techniques [58].
However, the cause–effect relationship between EPEC in the stools and diarrhea is hampered by the high number of healthy carriers.
EHEC
It is another major intestinal pathogen that is a subset of Shiga toxin-producing E. coli (STEC). E. coli O157:H7 is the prototype of EHEC serotype [59].
EHEC adheres to epithelial cells, expresses a T3SS and causes A/E lesions much like EPEC. Unlike EPEC, infection with EHEC may cause severe symptoms including bloody diarrhea and life-threatening HUS. These symptoms are due to the production of Shiga toxin which elicits luminal fluid accumulation in the intestine [60, 61]. The predominant transmission is through the ingestion of contaminated food. Crampy abdominal pain and non-bloody diarrhea are the first symptoms, sometimes associated with vomiting. Diarrhea always becomes bloody and abdominal pain worsens, lasting 1–22 days. Fever is usually absent or low grade. In outbreaks, approximately 25 % of patients are hospitalized, 5–10 % develop HUS, and 1 % may have a poor outcome. The most dangerous complication of EHEC infection is HUS. This is usually diagnosed 2–14 days after the onset of diarrhea. Risk factors include young age, bloody diarrhea, fever, an elevated leukocyte count, and treatment with antimotility agents [62]. The most widely accepted indication for seeking E. coli O157:H7 infection is a patient with bloody diarrhea, in whom an accurate diagnosis may avoid unnecessary surgery.
DAEC
DAEC has an age target ranging from 48 to 60 months and shows a seasonal pattern similar to that of ETEC, occurring more frequently in the warm season. The gastrointestinal symptoms include self-limiting watery diarrhea rarely associated with vomiting and abdominal pain. The diagnosis is mainly based on the DNA probe technique and on the pattern of adherence of the microorganism to HEp-2 cells. Given the technical problems of both assays, their use is limited to epidemiological surveys rather than diagnosis.
EAggEC
Typical clinical features are a watery, mucoid, secretory diarrheal illness with low-grade fever, and little or no vomiting. However, bloody stools have been reported. Infection of EAggEG is detected by the isolation of E. coli from the stools of patients and the demonstration of the aggregative pattern in the HEp-2 assay. Establishing a cause–effect relationship is hampered by the high rate of asymptomatic colonization; if no other organism is implicated in the patients’ illness and EAggEC is isolated, the germ should be considered as a potential cause of diarrhea. A DNA-fragment probe has proven highly specific in the detection of EAggEC strains. Acute diarrhea is apparently self-limiting; however, more persistent cases may benefit from antibiotic and/or nutritional therapy, after susceptibility test [66].
EIEC
Invasive E. coli strains are genetically, biochemically, and clinically nearly identical to Shigella [67]. They also show a similar epidemiological pattern and are endemic in developing countries. The role of EIEC in industrialized countries is limited to rare food-borne outbreaks. EIEC can rarely produce dysentery.
Antimicrobial Therapy
Antimicrobial therapy should not be given to the vast majority of otherwise healthy children with acute gastroenteritis. Acute gastroenteritis in a child without significant underlying disease is usually self-limited regardless of the etiology, which is seldom known at the onset of symptoms. Clinical recovery generally occurs within a few days without specific antimicrobial therapy, and the causative organism is cleared in a relatively short time, usually within a few days or weeks. Complications are uncommon.
Antimicrobial Therapy of Bacterial Gastroenteritis
Antibiotic therapy for acute bacterial gastroenteritis is not needed routinely but could be considered for specific pathogens or in defined clinical settings, including clinical condition and risk factors [68, 69].
Pathogen-Based Approach
Shigella Gastroenteritis
Antibiotic therapy is recommended for culture-proven or suspected Shigella gastroenteritis. The first-line treatment for shigellosis is azithromycin for 5 days. A meta-analysis of 16 studies, which included 1748 children and adults with Shigella dysentery, concluded that appropriate antibiotic therapy shortened the duration of the disease. Several well-designed controlled studies have shown that appropriate antibiotic treatment of Shigella gastroenteritis significantly reduces the duration of fever, diarrhea, and fecal excretion of the pathogen, and thus infectivity, which is very important in children attending day-care centers [70], those admitted in institutions and hospitals. Antibiotic treatment may also reduce complications including the risk ofHUS after S. dysenteriae infection.
WHO [71] recommends that all episodes of Shigella infection be treated with ciprofloxacin or one of the 3-s line antibiotics (pivmecillinam, azithromycin, or ceftriaxone). The major problem, however, is the increasing worldwide resistance of Shigella to antibiotics that is also being observed in Europe [72, 73]. Therefore, Shigella isolates should be tested for susceptibility, and local resistance pattern be closely monitored [74]. A systematic review of data from 1990 to 2009 identified 8 studies in children up to 16 years with shigellosis reporting clinical failure 3 days after treatment. In addition, four studies evaluated bacteriologic failure and five assessed bacteriologic relapse. Clinical failure rate was 0.1 % and bacteriologic relapse was 0 %. Based on these figures, which however derive from low-income countries, antibiotic therapy is consistently effective and strongly recommended in all children with shigellosis. It should be noted, however, that this finding has not been demonstrated in outpatients. Because of the high worldwide resistance, trimethoprim–sulfamethoxazole (TMP–SMX) and ampicillin are recommended only if the strain isolated is susceptible, or if current local microbiologic data suggest susceptibility. In Europe and the USA, resistance to ceftriaxone, azithromycin, and ciprofloxacin has been reported, but is uncommon. The first-line oral empiric treatment recommended for Shigella gastroenteritis is azithromycin for 5 days [72, 73]. Alternatively, nalidixic acid or cefixime can be administered for 5 days. When Shigella isolates are susceptible to (TMP–SMX) and/or ampicillin (i.e., in an outbreak setting), these agents are recommended as first-line treatment. Oral fluoroquinolones can be used in children younger than 17 years when no other alternative is feasible. The recommended first-line parenteral treatment is ceftriaxone for 5 days. Two doses of ceftriaxone can be given to patients without underlying immune deficiency or bacteremia who are fever-free after 2 days of ceftriaxone treatment [75].
Salmonella Gastroenteritis
Antibiotic therapy is not effective and does not prevent complications. Rather, it is associated with a prolonged fecal excretion of Salmonella. Therefore antibiotics should not be used in an otherwise healthy child with Salmonella gastroenteritis. Antibiotics are suggested in high-risk children to prevent the risk of bacteremia and extraintestinal infections. These include neonates and young infants (< 6 months) and children with underlying immune deficiency, anatomical or functional asplenia, corticosteroid or immunosuppressive therapy, IBD , or achlorhydria (weak recommendation, low-quality evidence). A Cochrane systematic review showed that antibiotic therapy of Salmonella gastroenteritis does not significantly affect the duration of fever or diarrhea in otherwise healthy children or adults compared to placebo or no treatment [76]. Moreover, antibiotics were associated with a significant increase of carriage of Salmonella, although other adverse events were not reported. As secondary Salmonella bacteremia—with potential extra-intestinal focal infections—occurs more often in children with certain underlying conditions, and in neonates or young infants; antibiotic therapy with TMP–SMX, ampicillin (to which 10–20 % of isolates in the USA are resistant), cefotaxime, ceftriaxone, or chloramphenicol, is suggested in these children to reduce the risk of bacteremia.
< div class='tao-gold-member'>
Only gold members can continue reading. Log In or Register a > to continue
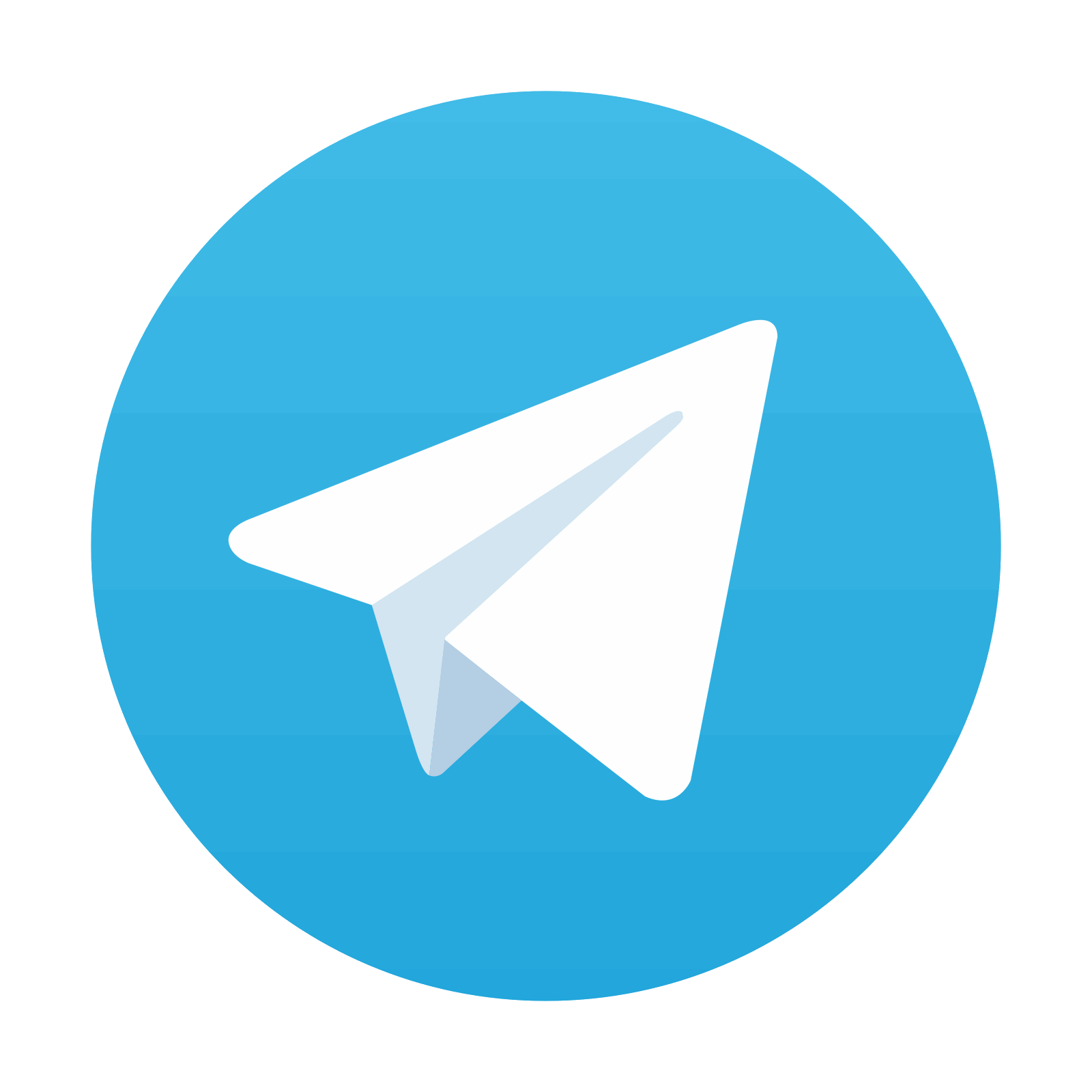
Stay updated, free articles. Join our Telegram channel

Full access? Get Clinical Tree
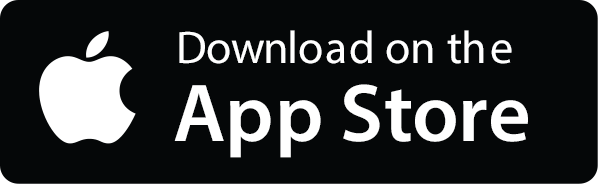
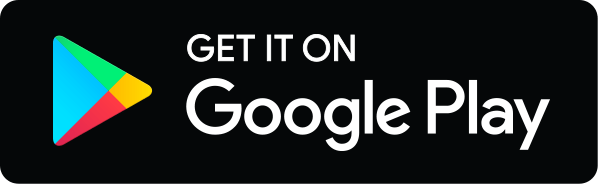