Fig. 12.1
Azathioprine (AZA) metabolism. XO xanthine oxidase, 6-TU 6-thiouric acid, and 6-TIMP 6-thioinosine monophosphate
An apparent genetic polymorphism has been observed in TPMT activity in both the Caucasian and African-American population. Negligible activity is noted in 0.3 % of individuals and low levels (<5 U/mL of blood) in 11 % of individuals. TPMT enzyme deficiency is inherited as an autosomal recessive trait, and to date, 10 mutant alleles and several silent and intronic mutations have been described. In patients with the heterozygous TPMT genotype, 6-MP metabolism is shunted preferentially into the production of 6-TG nucleotides. Although 6-TG nucleotides are thought to be lymphocytotoxic and beneficial in the treatment of patients with leukemia and lymphoma, patients with low (<5) TPMT activity are at risk for bone marrow suppression by achieving potentially toxic erythrocyte 6-TGN levels on standard doses of 6-MP [16]. Despite low TPMT enzyme activity levels, presumed therapeutic erythrocyte 6-TGN metabolite levels can still be achieved without untoward cytotoxicity by lowering the dose of 6-MP 10- to 15-fold [17].
6-TGNs are active ribonucleotides that collectively function as purine antagonists, incorporating into DNA, thereby interfering with the ribonucleotide replication. Recent studies have also shown that one of these 6-TGN ribonucleotides, 6-TGTP, induces the apoptosis of both peripheral blood and intestinal lamina propria T-cell lymphocytes through the inhibition of Rac1, a GTPase that inhibits apoptosis. The specific blockade of CD28-dependent Rac1 activation by 6-TGTP is the proposed molecular target of 6-MP and its prodrug AZA (Fig. 12.1) [18].
The intracellular buildup of this specific 6-TGN metabolite may also be dependent on others, as yet undefined inherent genetic polymorphisms. Our recent studies have also proposed that there may also exist pharmacogenetic differences in the intracellular transport of 6-MP in peripheral blood lymphocytes that could potentially affect responsiveness to antimetabolite therapy. Our studies have shown an inherent variability in the transport of 6-MP in immortalized lymphocytes derived from patients with IBD. In these studies, seven inward and eight outward transporters were tested. One patient demonstrated the least amount of intracellular transport of 6-MP that correlated with the lowest susceptibility to 6-MP cytotoxicity. In this particular patient, multiple inward transporters, including the concentrative nucleoside transporters CNT-1, CNT-3, and the equilibrative nucleoside transporters ENT-3 and ENT-4 were notably low in expression. In comparison, a second patient exhibited robust 6-MP transport, an increased susceptibility to 6-MP cytotoxicity, and an increased expression of all influx transporters (except CNT-1), and equilibrative transporter ENT-4. Although no single transporter was either under- or overexpressed to explain these patterns of 6-MP transport, a correlation was shown between intracellular drug levels and the in vitro susceptibility to 6-MP-induced cytotoxicity. Interestingly, these differences were independent of 6-MP dose or erythrocyte 6-MP metabolite levels that were monitored clinically. Ongoing studies will also attempt to correlate these differences in drug transport with clinical responsiveness to antimetabolite therapy and drug metabolite levels. Identification of such transporters prior to initiating therapy may allow physicians to tailor therapy more effectively in patients with steroid-dependent IBD [11].
Clinical Application of Metabolite Testing
In patients with UC, the aim is to optimize antimetabolite therapy early in the course of the disease in order to minimize the overall risk for disease progression. The factor with the most significant direct correlation with disease progression is severity of colitis early in the course of the illness [5]. In a large population-based cohort study, patients with severe active UC were 14.8 times more likely to have disease progression compared to patients without severe colitis. Patients with left-sided colitis at diagnosis are 2.5 times more likely to progress to extensive colitis than patients with isolated proctitis progressing to either extensive colitis or left-sided disease [19]. Although disease progression can occur in patients of all age groups, most children will present clinically with extensive colitis at diagnosis, while those children presenting with either proctosigmoiditis or left-sided disease will rapidly progress to pancolitis within 6 years of the diagnosis [20]. In general, pediatricians regard ulcerative colitis as a rapidly progressive disease in children, with an associated increased likelihood of requiring proctocolectomy. The rapid induction and maintenance of disease remission remain the primary goal therapy in patients with UC. Using a Markov model, there is an 80–90 % probability that a patient with clinically inactive disease would remain in remission for a year, with a 20 % chance of relapse in the following year. By contrast, data from patients with clinically active disease demonstrate a 70 % probability of having a relapse during the year following diagnosis [21]. The same results were shown within the post hoc analysis of the combined ACT I and ACT II data among the infliximab-treated patients. Interestingly, mucosal healing was the primary end point of long-term remission in those studies [22]. The importance of tissue healing was also underscored by Froslie and coworkers. In that study, patients with UC that achieved tissue healing at 1 year were less likely to require colectomy in the subsequent 5-year follow-up period [23].
Although 6-MP and AZA have clinical efficacy in maintaining disease remission in patients with UC, the wide therapeutic dosing range used in clinical practice today would suggest that pharmacokinetic differences in drug metabolism may also influence responsiveness to therapy. Moreover, a true separation between immunosuppression and cytotoxicity has yet to be defined since the dosing of 6-MP and azathioprine has been based largely on clinical outcome. Indeed, the wide range in azathioprine dose used in clinical practice would suggest that a safe and established therapeutic dose has yet to be determined. The situation is further complicated with recent evidence that would suggest that mucosal healing of the affected bowel decreases the risk of disease relapse and progression. Conventional dosing strategies must now be redefined based on these new end points of clinical remission that includes mucosal healing. Nevertheless, immunosuppression is not without its risk. The clinician must always remain aware of potential adverse effects, including allergic reactions, hepatitis, pancreatitis, bone marrow suppression, and lymphoma while attempting to achieve an optimal therapeutic response irrespective on how the physician chooses to define it clinically [24, 25].
The measurement of erythrocyte 6-TG and 6-MMP metabolite levels by means of high-pressure liquid chromatography (HPLC) has now become a useful clinical tool for documenting patients’ compliance to therapy. In our preliminary study, erythrocyte 6-TG metabolite levels showed a strong inverse correlation with disease activity, where the lack of clinical response was clearly associated with low (<50) erythrocyte 6-TGN metabolite levels. To date, a number of studies in both the pediatric and adult literature have supported the notion of therapeutic drug monitoring in patients with IBD. However, a uniform consensus has not yet been reached on account of the absence of well-controlled clinical trials (Table 12.1) [7–9, 26–29]. Although a meta-analysis by Osterman and colleagues has shown that higher metabolite levels correlated with a more favorable clinical response, no clearly defined therapeutic window of efficacy and toxicity has been established based on 6-MP metabolite levels [30]. Since mucosal healing has now become the salient end point for clinical remission, metabolite testing should now be considered just as a guide to therapy. The notion of using the existing threshold 6-TGN metabolite levels would seem antiquated. At present, the existing technology should only be used in identifying pharmacogenomic differences in drug metabolism, monitoring patient compliance with antimetabolite therapy, and avoiding excessive immunosuppression in patient with recalcitrant disease, high (>400) 6-TGN levels, and normal white blood cell counts.
Table 12.1
Clinical responsiveness to 6-MP and AZA therapy based on threshold (235–250a) erythrocyte 6-TGN metabolite levels
Study | Patients (response) | 6-TGN response threshold | Odds ratio | |
---|---|---|---|---|
Above | Below | |||
Dubinsky [26] | 92 (30) | 0.78 | 0.40 | 5.0 |
Gupta [27] | 101 (47) | 0.56 | 0.43 | 1.7 |
Belaiche [28] | 28 (19) | 0.75 | 0.65 | 1.6 |
Cuffari [7] | 82 (47) | 0.86 | 0.35 | 11.6 |
Achkar [8] | 60 (24) | 0.51 | 0.22 | 3.8 |
Lowry [9] | 170 (114) | 0.64 | 0.68 | 0.9 |
Goldenberg [29] | 74 (14) | 0.24 | 0.18 | 1.5 |
TPMT Testing
Low and Intermediate (<5 U/mL Blood) TPMT
Eleven percent of the population is considered heterozygous carriers of the TPMT-deficient allele and potentially at risk for drug-induced leukopenia. In the patient who is homozygous recessive with absent TPMT enzyme activity, there is the added risk of severe, irreversible bone marrow suppression. Since then, there have been a number of similar cases of irreversible bone marrow suppression both in patients with IBD on maintenance azathioprine therapy and in patients with leukemia on standard doses of 6-MP. It remains the author’s opinion that these patients should not be considered candidates for antimetabolite therapy.
A number of secondary malignancies, including acute myelogenous leukemia and brain tumors, have been insinuated to be related to the use of maintenance 6-MP therapy in patients with leukemia and the heterozygous TPMT genotype. Although 6-TG and 6-MMP metabolites were not measured in these patients, it may be assumed that these patients were potentially exposed to high-maintenance 6-TG metabolite levels despite presumed therapeutic 6-MP dosing and were thus overly immunosuppressed.
In IBD, Black and coworkers showed that patients with Crohn’s disease and a “mutant” TPMT allele also incurred significant drug-induced leukopenia on standard doses of azathioprine therapy and were compelled to discontinue treatment. In contrast, patients with the wild-type allele achieved a good clinical response while on azathioprine therapy without untoward cytotoxicity [31]. This study and others would suggest that all patients with the heterozygous allele are at an increased risk for drug toxicity and should not be prescribed azathioprine or 6-MP therapy. However, this would exclude 11 % of the population who could potentially benefit from 6-MP therapy. It has been shown in prospective open-label clinical trials that by identifying these patients prior to initiating AZA therapy and adopting a moderate dosing strategy (6-MP, 0.5–1 mg/kg/day; AZA, 1–1.5 mg/kg/day), most patients may achieve a favorable clinical response while avoiding potential bone marrow suppression. It remains the author’s opinion that these patients be monitored carefully with serial CBCs.
High (>16 U/mL Blood) TPMT
The genetic polymorphism in TPMT activity observed in the general population may also have far-reaching implications regarding patient responsiveness to therapy and clinical response time. Twenty percent of the population is considered to be rapid (>16) metabolizers of 6-MP and AZA and in theory would require larger than the standard doses of drug in order to achieve any therapeutic drug benefit [21]. In these patients, 6-MP metabolism is shunted away from 6-TGN production and into the formation of 6-MMP (Fig. 12.1). In patients with leukemia, high TPMT activity is associated with an increased risk for disease recurrence [17].
In a prospective open-label study in adults, just 20 % of patients with either UC or Crohn’s disease and erythrocyte TPMT levels >16 U/mL of blood responded to AZA therapy despite therapeutic drug dosing (2 mg/kg/day). In comparison, 30 % of patients with TPMT levels between 12 and 16 U/mL blood responded to therapy. These were also more likely to require higher dosages (2 mg/kg/day) of AZA from the outset in order to optimize their erythrocyte 6-TGN metabolite levels [21].
In comparison, patients with TPMT activity levels ≤12 U/mL blood achieved high (>250) mean erythrocyte 6-TG levels after 16 weeks of induction AZA. This occurred even though both groups received a similar dosage of AZA. In this patient population, 69 % of patients achieved a favorable clinical response with presumed therapeutic erythrocyte 6-TGN metabolite levels after 4 months of continuous AZA therapy [21].
High hepatic TPMT activity may draw most of the 6-MP from the plasma, thereby limiting the amount of substrate available for the bone marrow and peripheral leukocytes. This concept of rapid AZA metabolism interfering with therapeutic response could explain the low response rate in a controlled clinical trial in Crohn’s disease that compared high-dose oral (2 mg/kg/day) azathioprine therapy with and without initiating a short course of high-dose intravenous (1.6 g/36 h infusion) AZA therapy. That study was confined to individuals with upper normal or high levels of TPMT enzyme activity so that the intravenous azathioprine treatment group could be studied safely. Even at 2 mg/kg/day of oral azathioprine therapy, only 20 % of these rapid metabolizers in both groups achieved clinical remission, a clinical response that is lower than that reported in most consecutive patient publications [32].
Furthermore, high (>15) erythrocyte TPMT levels may also explain the rather low clinical response noted in the AZA treatment arm of the SONIC trials. In that study, despite optimized induction dosages (2.5 mg/kg/day) of AZA, just 30 % of patients responded to therapy [33], a clinical response that is lower than what has been generally concluded from the Cochrane meta-analyses of AZA therapy in treating patients with IBD [34].
Clinical Application of TPMT Testing
Most physicians will monitor CBC and serum aminotransferases monthly during the first 3 months of initiating therapy. Although TPMT measurement has been shown to predict leukopenia in up to 20 % of patients, TPMT monitoring may be used clinically to increase the level of physician comfort in prescribing antimetabolite therapy, in general, and in minimizing the perceived need for monitoring CBC, and for dose titration, all of which may increase clinical response time.
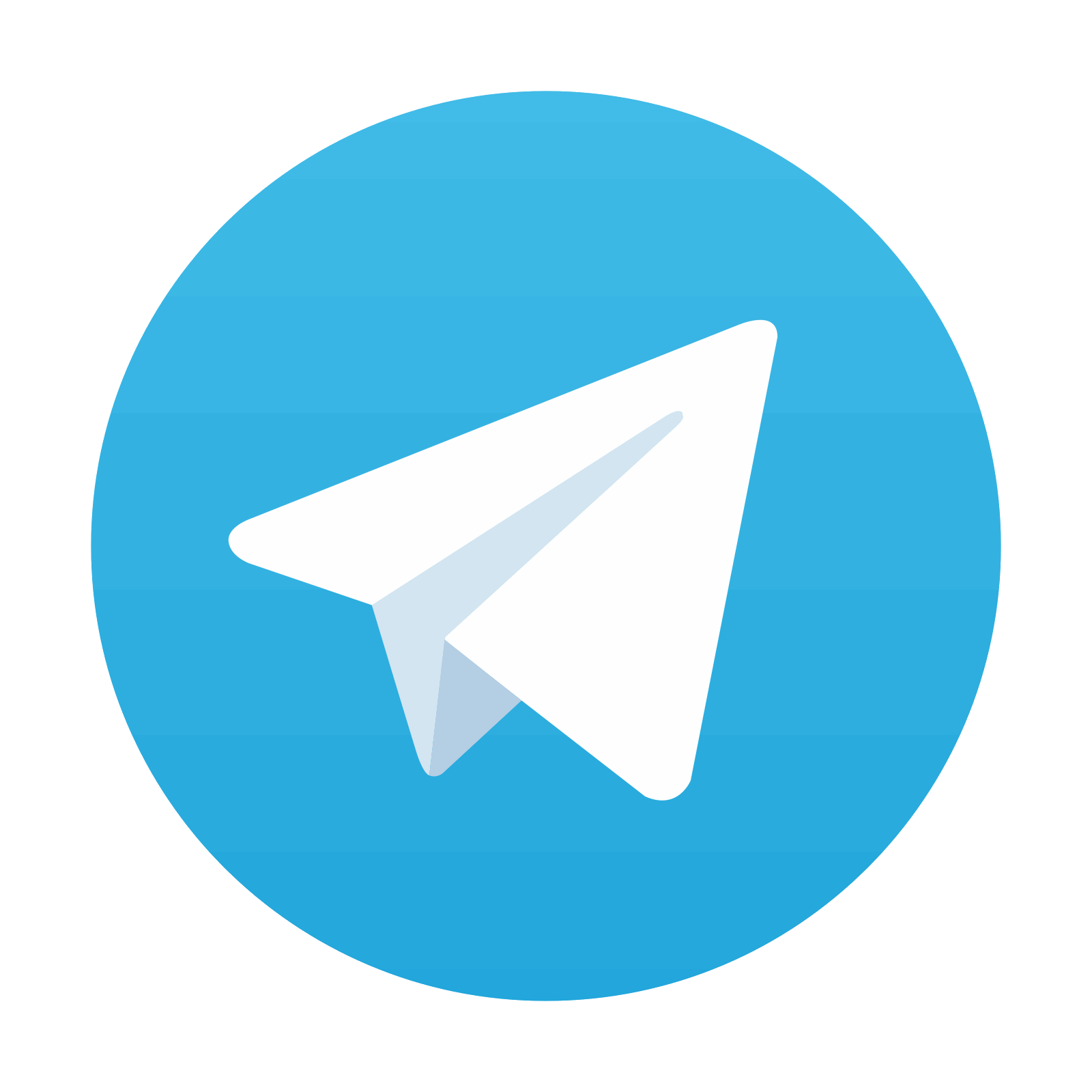
Stay updated, free articles. Join our Telegram channel

Full access? Get Clinical Tree
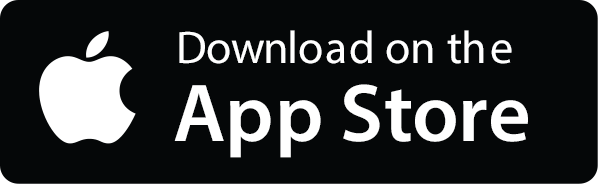
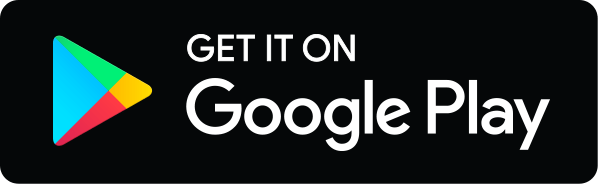