“There is no disease more conducive to clinical humility than aneurysms of the aorta.”
—Sir William Osler
Key Concepts
Epidemiology
The leading cause of aneurysms of the ascending aorta is medial degeneration, which may be idiopathic or accelerated by heritable disorders of connective tissue; conversely, arch aneurysms are due to chronic dissection or long-standing hypertension and atherosclerosis.
Pathophysiology
Histopathologic investigations of aneurysmal tissue have shown that a majority of ascending aortic and arch aneurysms are associated with medial degeneration in the layers of the aortic wall. This is characterized by a triad of (1) loss of noninflammatory smooth muscle cells, (2) fragmentation of elastic fibers, and (3) accumulation of basophilic ground substance in cell-depleted areas of the medial layer of the vessel wall. This combination of factors weakens the aortic wall, thereby increasing wall tension; this can induce aortic dilatation and aneurysm formation, leading to higher wall stress and eventually resulting in intramural hemorrhage, aortic dissection, or rupture. Medial degeneration can be accelerated by inborn errors of metabolism that affect any component of the aortic connective tissue. Known heritable disorders of connective tissue that commonly manifest as ascending and arch aneurysms of the aorta are Marfan syndrome, Ehlers–Danlos syndrome, Loeys–Dietz syndrome, familial forms of thoracic aortic aneurysm and dissection, and bicuspid aortic valve disease; Turner syndrome and Noonan syndrome occur far less frequently.
Clinical features
Most patients with thoracic aortic aneurysms are asymptomatic at the time of presentation, and aneurysms of the ascending aorta usually are detected as incidental findings during testing for other disorders. In contrast, because of their anatomic location and the space-occupying nature of the aneurysm, aneurysms of the aortic arch may impinge on important mediastinal structures and are therefore symptomatic more often than aneurysms of the ascending or descending aorta. Symptoms attributable to the aneurysm are cause for concern and consideration for early surgical intervention. Patients with rupture of an ascending or arch aneurysm often present in extremis.
Diagnostics
The most common finding on chest x-ray for a thoracic aortic aneurysm is widening of the superior mediastinum; other findings include displacement and compression of the mediastinal contents and curvilinear calcification in the aneurysmal wall. Echocardiography is widely available, can be noninvasive [transthoracic echocardiography (TTE)] or minimally invasive [transesophageal echocardiography (TEE)], and is relatively inexpensive. TTE can visualize the aortic root and ascending aorta accurately and is suitable for evaluating the size of moderate aneurysms and assessing cardiac complications such as aortic regurgitation (AR), impaired left ventricular function, and hemopericardium. Intraoperative application of TEE allows continuous monitoring of the surgical procedure without disturbing the sterile field. Because of its speed and wide availability, computed tomography/computed tomography angiography (CT/CTA) is currently the most common diagnostic imaging method for studying the aorta. CTA has the advantage of demonstrating aortic wall thickening, calcification, and luminal thrombus, thus displaying the true axial extent of an aneurysm. Magnetic resonance angiography is emerging as a diagnostic tool but is less readily available and has limited utilization in acute aortic problems because of its limited applicability.
Treatment
Symptomatic patients with ascending or arch aneurysms should be evaluated urgently for surgical repair. Patients presenting with rupture should be operated on urgently, since these conditions are almost uniformly fatal without prompt surgical intervention. Operative intervention in a patient with a non-heritable connective tissue disorder should be considered when the ascending aortic aneurysm diameter ranges from 5 to 5.5 cm. For patients with an aneurysm diameter less than 5 cm who are being monitored with serial imaging, an indication for surgical intervention would be a 10 percent increase in size over a 6- to 12-month time course. For patients with a heritable disorder of connective tissue, surgical intervention for the ascending aorta should be considered at a diameter of 4 cm for Loeys–Dietz syndrome, 4 to 4.5 cm for Marfan syndrome, 4.5 to 5.0 cm for bicuspid aortic valve, and 5.0 cm for Ehlers–Danlos syndrome. Factors that prompt the recommendation for surgery when the aorta is less than 5.0 cm include rapid growth of the aortic diameter (more than 1 cm/year), a family history of premature aortic dissection (dissection at less than 5.0 cm), and the presence of moderate or severe AR. Operative repair varies with the underlying pathology and the quality of the aortic wall, the status of the aortic valve, expected survival, and the general well-being of the patient.
Outcomes
Progress over the past three decades, including improved surgical instrumentation and advances in perfusion strategies with resultant improvements in myocardial and cerebral protection have augmented the surgical armamentarium significantly, facilitating the management of complex aortic problems. Replacement of the ascending aorta is a safe and effective procedure, with operative mortality rates of 2 to 5 percent. The operative mortality varies with the acuity of the operation, patient age, left ventricular function, and extent of operation. Late survival after operation is approximately 65 percent at 5 years and 55 percent at 7 years. For patients with Marfan syndrome, a valve-sparing aortic root replacement is a reasonable alternative to a composite valve graft. Survival is excellent with reimplantation and remodeling techniques and complications are rare, but the long-term durability of these repairs have not been established. For aortic arch replacement, the average operative mortality rate is 13 percent, with long-term survival ranging from 61 to 82 percent at 5 years and 31 to 69 percent at 10 years. Despite these advances, providing adequate cerebral protection and preventing cerebral embolization during reconstruction of the calcified and atherosclerotic aorta are formidable challenges.
Surgical repair of ascending and arch aneurysms of the aorta is one of the most formidable challenges facing the cardiovascular surgeon. The challenges stem from the often-catastrophic consequences of rupture of the thoracic aorta, the variegated clinical presentations associated with aortic disruption, and the risk of incurring devastating neurologic injury during aortic arch repair. This chapter describes the epidemiology, pathophysiology, clinical features, diagnosis, treatment, and outcomes of ascending and arch aneurysms of the aorta.
A true aortic aneurysm is defined arbitrarily as a 50 percent enlargement of the normal aorta for a particular body surface area, age, and gender.1 Clinically, the term usually is reserved for ascending aortic (sinus or tubular) diameters greater than 3.5 to 4.0 cm or aortic arch diameters greater than 4 cm. Some experienced thoracic aortic surgical centers use a ratio of 2× in which the diameter of the enlarged aortic segment is in the numerator and that of the normal contiguous aorta is in the denominator.2 The combination of dilation of the ascending aorta and dilation of the aortic annulus is termed annuloaortic ectasia.3 A false aortic aneurysm is a localized dilation whose wall consists of adventitia, some or all of the media, and compressed periaortic tissue usually associated with trauma, infection, or previous operations on the aorta. The classification of thoracic aortic aneurysms is based on the location, morphologic characteristics, or etiology.4
The location of the aneurysm affects clinical manifestations, natural history, and treatment options as well as providing insight into the etiology of the aneurysm formation. Thoracic aortic aneurysms can involve the ascending aorta, the arch, or the descending aorta. The ascending aorta is the portion of aorta starting at the aortic annulus and ending at the origin of the innominate artery near the pericardial reflection. The ascending aorta is separated further into a proximal sinus portion, also known as the aortic root, and a distal tubular portion, with the demarcation line occurring at the sinotubular junction (STJ), which is at the top of the aortic valve commissures (Fig. 39-1). These distinctions are important in that operations involving the aortic root require repair or replacement of the aortic valve and reimplantation of the coronary arteries. The aortic arch begins at the proximal origin of the innominate artery, traverses in conjunction with the brachiocephalic vessels, and ends at the distal origin of the left subclavian artery, dividing the aortic arch into the proximal, transverse, and distal sections, respectively. Aneurysms of the descending thoracic aorta originate distal to the origin of the left subclavian artery, whereas thoracoabdominal aneurysms originate in the descending thoracic aorta and extend below the diaphragm to involve various extents of the abdominal aorta.
Figure 39-1
The static and basic functional anatomy of the aortic root-valve complex. Note that the sinotubular junction diameter is approximately 15 percent less than that of the base of the aorta (putative aortic annulus). The DaVinci sinus currents are depicted; they enhance leaflet closure at the end of systole and protect leaflets from wall impact during systole. In addition, movement of the annulus changes the tension–length relationships of the leaflets to enhance function. It is difficult to demonstrate in a two-dimensional figure the time-dependent expansion of the aortic root that occurs during the first third of systole and the change in its overall configuration from a truncated cone shape with acorn protuberances of the sinuses to a larger, more cylindric form that would offer less impedance to ejection. FM, free margin; B, base of semilunar leaflet insertion; STJ, sinotubular junction; AA, aortic annulus (defined as the base of the aorta, not the semilunar leaflet attachment scalloped edge). (Reproduced, with permission, from Hopkins RA. Aortic valve leaflet sparing and salvage surgery: Evolution of techniques for aortic root reconstruction. Eur J Cardiothorac Surg 2003;24(6):886–897.)

The gross pathoanatomic description of thoracic aortic aneurysms includes fusiform and saccular aneurysms. Fusiform aneurysms involve a long segment of the aorta with uniform dilation involving the whole circumference of the aortic wall, whereas saccular aneurysms usually involve only a localized segment of the aorta with an eccentric dilation that communicates with the main lumen of the aorta by a variable-sized neck.4
Classification by etiology provides the most meaningful nomenclature for thoracic aortic aneurysms because of the treatment implications but at the same time is the most difficult to do. This stems from the variety of causes of thoracic aortic aneurysms, which histologically look very similar. The leading cause of aneurysms of the ascending aorta is medial degeneration, which may be idiopathic or accelerated by heritable disorders of connective tissue; conversely, arch aneurysms are due to chronic dissection or long-standing hypertension and atherosclerosis. This is discussed in more detail in the pathophysiology section.
The first description of arterial aneurysms is attributed to Galen, who observed false aneurysms in gladiators injured during battle in the second century. During that period, Antyllus made the distinction between traumatic and degenerative aneurysms and was the first to attempt surgical treatment of aneurysms with proximal and distal ligation.5 In 1557, Vesalius6 was credited with the first correct clinical diagnosis, and Morgagni outlined fascinating and detailed case histories, postmortem findings, and commentaries on three cases of ruptured thoracic aneurysms in young adults in 1769. In his great work The Seats [Sites] and Causes of Diseases, he described the graphic case report of a “strumpet [prostitute] of eight-and-twenty years of age” who died suddenly in the act of performing her profession, causing considerable embarrassment to her client.7
The early operative treatment of thoracic aneurysms was entirely palliative, consisting of the introduction of wire within the sac to stimulate clot formation or the wrapping of cellophane around the sac to induce periarterial fibrosis. Before the availability of cardiopulmonary bypass (CPB), surgical treatment of thoracic aortic aneurysms was limited to resection of discrete saccular aneurysms associated with coarctations.7 Even though the first isolated thoracic aneurysm of the arch was resected by Monod and Meyer in Paris in 1950,8 the development of current aortic surgery procedures was contingent on improvements in vascular surgical instruments and the evolution of arterial grafts and bypass procedures in conjunction with CPB and the various perfusion techniques.
Cooley and DeBakey9 are credited with the first resection of an ascending aortic aneurysm and replacement with a graft in 1956; subsequently, a variety of approaches evolved. In 1960, Mueller and colleagues10 combined supracoronary graft replacement with bicuspidization of an incompetent aortic valve. In 1963, Starr and associates11 described supracoronary graft replacement and replacement of the aortic valve. In 1964, Wheat and coworkers12 described a technique of radical resection of the aortic wall that left small buttons of tissue adjacent to the coronary ostia, replacement of the aorta with a graft, and prosthetic replacement of the aortic valve. Bentall and DeBono13 and, independently, Edwards and Kerr14 introduced a method of replacing the aortic valve and ascending aorta simultaneously using a composite valve graft (CVG) and reimplanting the coronary ostia into the graft. After 1968, the CVG procedure evolved to include full-thickness end-to-side coronary anastomoses in the form of Carrel “buttons” of aorta and a full-thickness end-to-end distal aortic anastomosis rather than the original “wrap inclusion” or Bentall technique.2
Resection of aortic arch aneurysms has remained a more formidable surgical challenge. In 1957, DeBakey and colleagues15 reported the first successful replacement of an ascending and arch aneurysm with an aortic homograft, using CPB with separate innominate and left common carotid artery perfusion. Bloodwell and associates16 modified the basic CPB technique in 1968 to perfuse the carotid and vertebral arteries separately and implant the arch vessels together as a single “button.” Griepp and associates17 at Stanford first used the technique of circulatory arrest for complete replacement of the aortic arch in 1975 with implementation of the “open distal.” In 1983, the “elephant trunk” technique was introduced by Borst and associates18 as an alternative for the treatment of patients with extensive aortic aneurysmal disease involving the arch and the descending or thoracoabdominal aorta.
At one stage of development, two aortic arches (right and left) are present with normal development, including persistence of the left arch and involution of the right arch. Originating from the aortic arch are branching vessels, with many variations in both the number and the position of those vessels. Permutations may be as few as one or as many as six branches, with these anomalies intimately associated with the development of the fourth arterial arches (Fig. 39-2A). In about 80 percent of people, the branching order is innominate, left common carotid, and left subclavian; however, in 11 percent of these patients, a bovine arch exists where the innominate and the left common carotid have a common ostia and the left subclavian arises independently from the arch (Fig. 39-2B).19,20 Aberrant right subclavian artery (ARSCA) affects approximately 0.1 percent of the population and is often an incidental finding on imaging studies. It occurs when the right fourth aortic arch and the right dorsal aorta involute cranial to the seventh intersegmental artery.21 The ARSCA arises from the descending aorta or a diverticulum and crosses to the right behind the esophagus (Fig. 39-2C). Surgeons must be aware of the possible variations in the great vessels arising from the aortic arch because misidentification during surgery could be catastrophic.
Figure 39-2
Arch development and anomalies. A. Diagrams showing transformation of the early aortic arch pattern (6–8 weeks) into the adult pattern. (Reproduced with permission from Moore KL. The Developing Human: Clinically Oriented Embryology. Philadelphia: WB Saunders Co., 1982.) B. Aortogram demonstrating a bovine arch with a pseudoaneurysm near the origin of the innominate artery. (Reproduced with permission from Jweied E, Fogelson B, Fishman D, Merlotti G. Blunt injury of the innominate artery associated with a bovine arch. J Trauma 2002;52(5):1002–1004.) C. Diagram showing the embryologic basis of an aberrant right subclavian artery. (Reproduced with permission from Moore KL. The Developing Human: Clinically Oriented Embryology. Philadelphia: WB Saunders Co., 1982.)

The aortic wall is organized into three distinct layers: the intima, media, and adventitia (Fig. 39-3). The media of the aorta is composed of smooth muscle cells within a matrix of elastin, collagen, and other structural proteins, including fibrillin, laminin, glycosaminoglycans, proteoglycans, and fibronectin. The overall strength of the aortic wall is determined by the relative amounts of elastin and collagen; elastin conveys the arterial recoil capabilities, and collagen provides the necessary structural strength. Defects in either protein can cause aortic pathology.22 Table 39-1 lists the etiologies of thoracic aortic aneurysms; these entities and their clinical implications are described in the following section.
Figure 39-3
Artery wall. Diagrams of a muscular artery prepared by hematoxylin and eosin (H&E) staining (left) and an elastic artery stained by Weigert’s method (right). The tunica media of a muscular artery contains predominantly smooth muscle, whereas the tunica media of an elastic artery is formed by layers of smooth muscle intercalated by elastic laminae. The adventitia and the outer part of the media have small blood vessels (vasa vasorum) and elastic and collagenous fibers. (Reproduced with permission from Junquiera LC. Basic Histology Text and Atlas, 11th ed. New York: McGraw-Hill, 2005.)

|
Histopathologic investigations of aneurysmal tissue have shown that the majority of ascending aortic and arch aneurysms are associated with medial degeneration in the layers of the aortic wall.23,24 These degenerative changes within the aortic media, which historically and incorrectly were called medial necrosis or cystic medial necrosis,25 are characterized by a triad of (1) loss of non-inflammatory smooth muscle cells, (2) fragmentation of elastic fibers, and (3) accumulation of basophilic ground substance in cell-depleted areas of the medial layer of the vessel wall. This combination of factors first weakens the aortic wall, thereby increasing the wall tension, which can induce aortic dilatation and aneurysm formation. In turn, this leads to higher wall stress, eventually resulting in intramural hemorrhage, aortic dissection, or rupture. Medial degeneration occurs normally to some extent with aging, but the process is accelerated by hypertension.26 In addition, turbulent flow within the aneurysm cavity frequently leads to the formation of laminated thrombus and further atherosclerotic depositions in the aortic wall. The thrombus lining and aortic calcifications, although often substantial, convey no added strength for the prevention of rupture.22
Medial degeneration is accelerated by inborn errors of metabolism that affect any component of the aortic connective tissue (i.e., ground substance, collagen, elastin, or smooth muscle cells). Known heritable disorders of connective tissue that commonly manifest as ascending and arch aneurysms of the aorta are Marfan syndrome (MFS), Ehlers–Danlos syndrome (EDS), Loeys–Dietz syndrome (LDS), familial forms of thoracic aortic aneurysm and dissection, and bicuspid aortic valve (BAV) disease; Turner syndrome and Noonan syndrome occur far less frequently.
Among hereditary diseases, MFS is the most prevalent inherited connective tissue disorder, with a prevalence of approximately 1 in 3000 to 5000 individuals. The diagnosis is clinical, relying on the revised Ghent consensus criteria.27 The condition is inherited in an autosomal dominant manner with complete penetrance but demonstrates variable expression with significant intra- and interfamilial variation (Fig. 39-4).2 More than 100 mutations have been identified in the fibrillin-1 gene on chromosome 15, as well as more recently identified missense mutations in the transforming growth factor β (TGF-β) gene, which encodes for the TGF-receptor. As a result, defective coding of fibrillin, the major structural component of elastin, occurs as well as excessive TGF-β activity. The consequence is both a decrease in the amount of elastin in the aortic wall and a loss of the normally highly organized structure of elastin (Fig. 39-5). In addition, Marfan aortic tissue has enhanced expression of metalloproteinases, which may promote both fragmentation of medial elastic layers and elastolysis.28 The end result is an aorta that exhibits markedly abnormal elastic properties that lead to progressive increases in stiffness and dilation. Additional cardiovascular complications include mitral valve prolapse and regurgitation and left ventricular dilation, but aortic root dilation is the most common cause of morbidity and mortality.
Figure 39-5
Histology. Normal aorta stained with hematoxylin and eosin (H&E) × 120 (upper left) showing uniform distribution of elastin layers and fibromyocytes between them and Alcian blue/fuchsin/picric acid stain × 120 (lower left) with elastin stained red, fibromyocytes pale khaki-colored, and connective tissue pale gray–blue. Erdheim disease of the aorta stained with H&E × 120 (upper right) shows irregular distribution of fibromyocytes with irregularity and loss of elastic lamellae. In the lower right, Alcian blue/fuchsin/picric acid stain × 120 shows disruption and loss of elastic lamellae (red) and a hyperabundance of acidic mucin (green–blue).

EDS is a rare autosomal dominant inherited disorder of connective tissue that results from mutations in the COL3A1 gene, which encodes for type III collagen on chromosome 2. The classification of EDS has been simplified into six major types, including vascular EDS (formerly known as type IV EDS), which carries a substantial risk of rupture of the aorta and its major branches.29 Arteries in the thorax and abdomen account for 50 percent of the vascular complications,30 but any anatomic location can be involved, with a predilection for medium-size arteries.
The LDS is a recently described autosomal dominant aortic aneurysm syndrome that has widespread systemic involvement.31 A spectrum of mutations in transforming growth factor β receptors 1 and 2 (TGFBR1 or TGFBR2, respectively) cause the LDS, which histologically results in a loss of elastin content and disarrayed elastic fibers in the aortic media.32 The phenotype of LDS overlaps with that of MFS (aortic aneurysm, arachnodactyly, dural ectasia), but it also includes distinctive features such as widely spaced eyes (hypertelorism), bifid uvula and/or cleft palate, and generalized arterial tortuosity. The natural history of LDS differs considerably from other connective tissue disorders with the average age at a first cardiovascular event much lower than that for patients with untreated MFS or vascular EDS, suggesting that mutations in TGFBR1 or TGFBR2 predispose to more aggressive and widespread vascular disease.
It is coming to be known that patients without genetic syndromes such as MFS or EDS also manifest familial clustering of thoracic aortic aneurysms and dissections (TAAD). In the Yale database of over 1600 patients, detailed family trees of 300 families revealed that 21 percent of aneurysm probands have a first-order relative with a known or likely aortic aneurysm.33 Most pedigrees suggest an autosomal dominant mode of inheritance, but there is marked variability in the expression and penetrance of the disorder in which some inherit and pass on the gene but show no manifestation of the disease. These nonsyndromic patients have been studied, and to date three genomic loci for nonsyndromic familial TAAD have been elucidated: the TAAD1 locus mapped to the long arm of chromosome 5, with approximately half of the identified families mapping to this locus; the FAA1 locus on the long arm of chromosome 11, which is responsible for aneurysmal disease in one large family; and the MFS2 locus on chromosome 3, which is a rare cause of familial TAAD.4 Therefore, TAAD is now a recognized heritable disorder of connective tissue.
Turner syndrome is a sex aneuploidy syndrome in which the most common chromosome constitution is 45-X0; it has an incidence of 1 in 5000 live female births. The cardiovascular problems include BAV (present in one-third of subjects), coarctation of the aorta, hypertension, and TAAD. Aortic root dilation is present in approximately 40 percent of Turner syndrome patients. Another rare genetic disorder that occasionally predisposes to aortic aneurysms is Noonan syndrome, an autosomal dominant condition.
BAV is the most common congenital heart malformation, with a population prevalence of 1 to 2 percent. It is not clear whether BAVs are heritable, but groups have shown that there is a high incidence of familial clustering that is compatible with an autosomal dominant inheritance pattern with reduced penetrance.34–36 Familial aggregation studies have indicated that 9.1 percent of persons with BAV have a first-degree relative with BAV, with males and females equally affected.36 The clinical consequences of BAV disease focus on either valvular (stenosis, insufficiency, or infection) or vascular (dilation or dissection) complications. Sabet and coworkers37 performed a large pathologic review and revealed that BAV disease results in a stenotic lesion in three-quarters of patients, insufficiency in 15 percent, and a mixed lesion in 10 percent. The vascular complications of BAV disease are less well understood, but the presence of BAV is an independent risk factor for progressive aortic dilation, aneurysm formation, and dissection.38 In fact, BAV disease carries a 9- to 18-fold higher incidence of ascending aortic dilation.39,40 The cause of this association initially was attributed to “poststenotic dilation,” with high-velocity turbulent flow distal to a stenosis leading to downstream dilation. However, advances in molecular biology have offered new insights that implicate intrinsic abnormalities of the aortic wall as being responsible for the pathologic development of aortic dilation. Embryologically, the aortic valvular cusps and ascending arterial media both arise from common neural crest cells during development. Apoptosis of those neural crest derivatives, specifically premature vascular smooth muscle cell apoptosis within the aortic media, is present in ascending aortic aneurysms of BAV carriers.41,42 In addition, fibrillin-1 deficiency in the aortic media of BAV patients has been implicated in triggering matrix metalloproteinase (MMP) production, leading to matrix disruption and thus aortic dilation (Fig. 39-6).43 The cause of BAV is unknown, but it is associated with accelerated degeneration of the aortic media, indicating that BAV disease is an ongoing pathologic process, not a discrete developmental defect.44
Figure 39-6
The elastic laminae of the aortic media provide structural support and elasticity to the aorta. In normal tricuspid valve patients (A), fibrillin-1 microfibrils tether smooth muscle cells to adjacent elastin and collagen matrix components. In patients with BAV (B), deficient microfibrillar elements result in smooth muscle cell detachment, matrix metalloproteinase release, matrix disruption, cell death, and a loss of structural support and elasticity. (Reproduced, with permission, from Fedak PW, Verma S, David TE, et al. Clinical and pathophysiological implications of a bicuspid aortic valve. Circulation 2002;106(8):900–904.)

Both Stanford type A and type B chronic aortic dissections tend to dilate over time. The natural history starts with a vulnerable aortic wall at baseline, which is stressed with the tearing of the intimal flap, leaving the structural integrity of the aorta destabilized to only the weakened outer wall of the false lumen. Consequently, those with chronic aortic dissection are at high risk for aneurysm formation. Debakey and colleagues found that aneurysms developed in 46 percent of patients with uncontrolled hypertension but in only 17 percent with controlled hypertension.45 Recently, Griepp and coworkers found that after repair of acute type A dissections, the growth of the distal aorta averaged 0.85 mm a year for the aortic arch and 1.24 mm a year for the descending thoracic aorta.46
Osler47 adopted the term mycotic to denote aneurysms originating on the basis of infection.48 Mycotic aneurysms of the thoracic aorta are rare but can be fatal if they are not diagnosed early. Bacterial seeding of the aortic wall can occur by hematogenous spread to the intima or the vasa vasorum, lymphatic spread, or direct extension from an adjacent infected focus. The thin endothelial intimal lining of the aorta is generally highly resistant to infection, but disruption of this barrier by atherosclerosis reduces resistance to infection.49 Epidemiologically, this disease, which traditionally has been due to endocarditis, now has an increased tendency to afflict the elderly, possibly as a result of an atherosclerotic load facilitating infection. Aneurysms are usually saccular and well localized, with Staphylococcus aureus and Salmonella species being the predominant organisms. Surgery with complete excision and debridement of infected tissue with in-line aortic reconstruction is the definitive treatment. Lifelong prophylactic antibiotic therapy may be advisable to prevent recurrences unless there is a clearly identifiable and treatable source of infection.
Syphilis was once perhaps the most common cause of ascending aortic aneurysms, but in an era of aggressive antibiotic treatment, such luetic aneurysms are seen rarely in medical centers.50 Syphilitic aortitis is a chronic mesarteritis and periarteritis of the aorta, with patchy destruction and scarring of musculoelastic medial tissue and replacement by vascularized connective tissue.48 The complications of aortitis develop 10 to 20 years after onset of disease in most cases, with the most common manifestation being a localized saccular aneurysm in the ascending aorta.51
Walker and colleagues52 were the first to define the term inflammatory aneurysm. Typical histologic examination shows signs of chronic inflammation in the adventitia with a marked lymphoplasmacytic cell infiltrate and granulation or proliferation of fibrous tissue. Three inflammatory arteritides affect the ascending and arch of the aorta with aneurysmal disease: Takayasu arteritis, Behçet disease, and giant cell arteritis (GCA).
Takayasu arteritis is a nonspecific inflammatory disease of unknown etiology that mainly involves the medial layer in the elastic arteries such as the pulmonary artery, the subclavian artery, and abdominal branches of the aorta.53 The inflammatory process results in a predominantly chronic arterial occlusive lesion of the main branches of the aortic arch but occasionally results in arterial aneurysmal formation, following a cyclic course.54 Histologically, thickening of the intima is the normal occurrence, resulting in stenosis and occlusion; however, degeneration of the elastic tissue with proliferation of the connective tissue mainly in the medial layer of the arteries may result in dilatation of the artery and aneurysm formation.54
Behçet disease is a multisystemic disorder characterized by recurrent urogenital ulcers, ocular manifestations, and skin lesions, with cardiovascular involvement appearing in only 7 to 29 percent of patients.55 However, the leading cause of death in patients with Behçet disease is rupture of a large aortic or arterial aneurysm.56 Venous lesions are the norm, with arterial manifestations accounting for only 12 percent of vascular complications in patients with Behçet disease. The arterial lesion, which develops in the aorta and the pulmonary artery as well as in their major branches, is an aneurysm in 65 percent of patients and an occlusion in 35 percent.55 At histologic analysis, aortitis is seen in both the active and scar stages. Active aortitis leads to the destruction of the media and fibrosis, predisposing the patient to saccular aneurysms.57 Perforation of the arterial wall caused by obliterative endarteritis of the vasa vasorum may result in aneurysm formation or rupture.58
GCA, also known as temporal arteritis, cranial arteritis, granulomatous arteritis, and Horton disease, is a chronic systemic vasculitis of unknown etiology that affects medium-size and large arteries.59–61 It has been reported that as many as 15 percent of patients with temporal arteritis may have angiographic evidence of aortic involvement62 and that patients with GCA are 17.3 times more likely to develop a thoracic aortic aneurysm than is the general population.63 Typical histopathologic findings are disruption of the internal elastic lamina and an inflammatory cellular infiltrate with giant cells.61
Dilation of the ascending aorta is currently the most common cause of isolated aortic valvular regurgitation.64 A normal valve becomes incompetent as a result of the passive stretching of its leaflets and commissures caused by dilation of the sinotubular ridge, the ascending aorta, or the sinuses, although the aortic annulus often remains normal in size in the non-Marfan population.
The decision to recommend surgical therapy is based on the clinical assessment that the potential for complications during observation and the associated consequences are more significant than the morbidity and mortality of surgical repair. This clinical decision must rely on current knowledge of the natural history of ascending and arch aneurysms.
Unlike the natural history of untreated abdominal aortic aneurysms, the natural history of thoracic aortic aneurysms is ill defined, mainly because of the asymptomatic course of the disease until dissection, rupture, or the appearance of symptoms. The majority of the published information details the natural history of descending thoracic aneurysms, with substantially less information available for ascending or arch aneurysms. With that caveat, there is comprehensive information available for ascending and arch aneurysms related to MFS, but such data are lacking for other pathologies. It is appreciated that the size and etiology of the aneurysm contribute to the natural history of thoracic aortic aneurysms, which often concludes with patient death because of rupture or dissection.
According to Laplace law,
T = (P × R)/M
where T is the tension in the walls, P is the pressure difference across the wall, R is the radius of the cylinder, and M is the thickness of the wall; the combination of an enlarging radius and a thinning wall leads to increased wall tension. This represents the onset of a positive feedback cycle that results in progressive aortic dilation and culminates in rupture or dissection. In all reliable contemporary natural history studies, an ascending aortic diameter of 6 cm emerges as the mean or the median diameter quite consistently.65 Coady and associates found that a diameter of 6 cm is the “hinge point” beyond which there is a 30 percent increase in the probability of rupture.66 The 5-year survival rate for patients with ascending aortic aneurysms ranged from 36 to 61 percent in the earlier days67 and reached 59 to 77 percent in the modern era68 for aneurysms equal to or greater than 6 cm and less than 6 cm, respectively. Regarding aneurysm growth rates, Hirose and coauthors noted that the growth rate of aneurysms was greater in the arch (0.56 cm/year) than in the descending thoracic (0.42 cm/year) or the abdominal aorta (0.28 cm/year).69 A more recent review of the Yale database70 reported an annual growth rate of 0.07 cm for aneurysms in the ascending aorta or aortic arch and found that increasing size is associated more strongly with an increased risk of rupture than with an increased risk of dissection. In addition, the risk of rupture with time as a function of initial aneurysm size is 11 times worse with an aortic size of 5.0 to 5.9 cm and nearly 27 times worse with a size of 6.0 cm or greater when each is compared with aneurysms less than 4.0 cm.
In addition to aneurysm size, the etiology of the aneurysm has a profound impact on rupture, dissection, and death. The most common cardiovascular complication in patients with MFS is progressive aortic root enlargement that initially occurs at the sinuses of Valsalva. An ascending aortic aneurysm can precipitate acute type A dissection, aortic rupture, aortic regurgitation (AR), or all three, and these complications were the primary cause of death before the advent of successful preventive therapies.2 Currently, aortic dissection is the major cause of premature morbidity and mortality in patients with MFS. In a study by Murdoch and associates,71 that looked at causes of death in MFS patients, cardiovascular manifestations accounted for 93 percent of the deaths (52 of 56), with aortic root complications (dilation, dissection, and regurgitation) accounting for 80 percent of those deaths with an average age at death of 32 years. This was similar to the findings of Marsalese and coworkers, in which 87 percent of the known causes of death were attributable to the cardiovascular system and 61 percent were the result of aortic dissection, rupture, or sudden cardiac death with a mean age at death of 35 years.72
BAV is also an independent risk factor for progressive aortic dilation, aneurysm formation, and dissection.73 Dore and associates74 evaluated 50 adults with BAV with transthoracic echocardiography (TTE) and found that progressive dilation occurred at all levels (from the basal attachment of the leaflets to 1 cm beyond the STJ), ranging from 0.3 mm/year at the basal attachment within the left ventricular outflow tract to 1.0 mm/year 1 cm beyond the STJ. These rates of dilation are greater than the reported rate of 0.8 mm/decade in the normal population. In fact, BAV disease carries a 9-to-18-fold higher incidence of ascending aortic dilation.75,76 Furthermore, valve replacement of BAV for either aortic insufficiency or stenosis does not eliminate the risk of aortic complications. After aortic valve replacement, BAV patients, when compared with tricuspid aortic valve patients, have progressive dilation of the proximal ascending aorta77 and have a greater incidence of sudden death and aortic events.78 Thus, the influence of BAV disease on the ascending aorta is a reality; surgeons should consider valve pathology when making decisions about ascending aortic replacement.
The natural history of LDS, resulting from a spectrum of mutations in TGFBR1 or TGFBR2, differs considerably from other connective tissue disorders with the average age at the first cardiovascular event much lower than that for patients with untreated MFS or vascular EDS. In a cohort of 90 patients,32 the median survival was 37.0 years, with 27 deaths during the study period with the mean age of death being 26.0 years (range: 0.5–47.0), and the mean age at vascular surgery—most often for ascending aortic aneurysm or dissection—was 19.8 years (range: 1.2–46.0). Twenty-nine patients (32 percent of the cohort) died before the age of 19 as a result of a vascular dissection or rupture, suggesting that LDS is an aggressive connective tissue disorder with a worse cardiovascular risk profile than classic MFS, including aortic dissection at a young age and at small dimensions.
Most patients with thoracic aortic aneurysms are asymptomatic at the time of presentation, and aneurysms of the ascending aorta usually are detected as incidental findings during testing for other disorders. Examples include widening of the mediastinum or prominence of the aortic knob on routine chest radiography and aortic enlargement on echocardiography, thoracic computed tomography (CT), or magnetic resonance imaging (MRI). In contrast, aneurysms of the aortic arch, because of their anatomic location and the space-occupying nature of the aneurysm, may impinge on important mediastinal structures and are therefore symptomatic more often than aneurysms of the ascending or descending thoracic aorta.24 Hoarseness results from stretching of the left recurrent laryngeal nerve, stridor from compression of the trachea, dysphagia from impingement on the lumen of the esophagus, dyspnea from compression of the lung, and plethora and edema from compression of the superior vena cava (SVC).79 Aneurysms of the ascending aorta associated with dilation of the aortic valve annulus may present with signs and symptoms of AR. In addition, chest or back pain may indicate acute expansion or leakage of the aneurysm in which the location of discomfort roughly correlates with the involved aortic segment. Precordial pain is associated with involvement of the ascending aorta; radiation to the neck and jaw often accompanies aortic arch involvement, whereas descending thoracic and thoracoabdominal aortic aneurysms tend to produce interscapular and low back pain, respectively. In contrast, the clinical features of aortic dissection are the acute onset of chest and/or back pain of a blunt, severe, and sometimes radiating and migrating nature.80 Symptoms attributable to the aneurysm are cause for concern and consideration for early surgical intervention. Patients with rupture of an ascending or arch aneurysm often present in extremis (see Figs. 39-7 and 39-8).
The physical examination is often normal in a patient without rupture of an ascending or arch aneurysm, with the rare exception of a large aneurysm that is palpated in the suprasternal notch. Other physical findings may include venous distention secondary to superior vena caval or innominate vein obstruction. Aneurysms of the ascending aorta associated with dilation of the aortic valve annulus may present with signs of AR (i.e., widened pulse pressure, decrescendo diastolic murmur, bisferiens pulse, “pistol-shot sound,” Duroziez sign, and Quincke pulse). A thorough vascular examination should be carried out to identify concomitant peripheral vascular disease (abdominal aortic aneurysms are present in 10–20 percent of patients with atherosclerotic involvement of an ascending aortic aneurysm).81,82 The physical examination also should focus on identifying marfanoid features (Fig. 39-4) as well as phenotypic features of LDS (i.e., blue sclerae, hypotelorism, bifid uvula, malar flattening, retrognathia, camptodactyly, arachnodactyly, and translucency of the skin with visible veins and distended scars32).
There are no primary laboratory abnormalities associated with aortic aneurysmal disease.
The diagnosis of aortic aneurysms has been revolutionized by developments in cross-sectional imaging. Traditionally, these aneurysms were investigated by contrast angiography, but the last two decades have seen considerable developments in the diagnosis of aortic disease by echocardiography, CT, and MRI. These developments have led to a complete change in the way in which aortic disease is evaluated and have contributed to an improvement in treatment outcomes.83
When a thoracic aortic aneurysm is detected, it is typically not possible to determine the rate of growth; therefore, if the aneurysm does not meet the criteria for surgical intervention, it is appropriate to obtain a repeat imaging study 6 months after the initial study. If the aneurysm is unchanged in size, it is reasonable to obtain an imaging study on an annual basis in most cases. However, if there is a significant increase in aortic size from one study to the next but surgical intervention is still not indicated, the interval between studies should be decreased to 3 or 6 months. For surveillance to be useful, serial imaging studies should be performed in the same center with the same technique so that direct comparisons can be made between comparable images.50
The abnormality of a thoracic aneurysm may be detected on chest radiographs, with the most common finding being widening of the superior mediastinum. Other findings include displacement and compression of the mediastinal contents, such as the trachea and esophagus, and curvilinear calcification in the aneurysmal wall (Fig. 39-9).84 However, aneurysms that involve the ascending aorta and the aortic arch sometimes cannot be differentiated from tumors or other masses in the mediastinum.85
Both TTE and transesophageal echocardiography (TEE) play an important role in aortic imaging. Echocardiography is widely available, noninvasive (TTE) or minimally invasive (TEE), and relatively inexpensive. TTE can visualize the aortic root and ascending aorta accurately and is very suitable for evaluating the size of moderate aneurysms and assessing cardiac complications such as AR, impaired left ventricular function, and hemopericardium (Fig. 39-10). It is less than ideal for showing the full extent of the aortic arch and evaluating the origins of head and neck vessels. In contrast, TEE with the current multiplanar transducers has few blind areas (i.e., posterior to the trachea) that limit evaluation. Most important, because of its bedside applicability, TEE is the imaging modality of choice for the diagnosis and exclusion of ascending aortic dissection in an unstable patient, with a European multicenter study reporting a diagnostic sensitivity of 99 percent and a specificity of 98 percent.86 Combined with color flow imaging, TEE allows not only the identification of the intimal flap with the true and the false lumen and identification of the entry and reentry tears but also the detection of associated complications such as AR, pericardial and pleural effusions, coronary involvement, and thrombosis in the false lumen. Today, in most centers, patients with proximal aortic dissection diagnosed by TEE who are surgical candidates are taken directly to the operating room, saving valuable time. In addition, intraoperative application of TEE allows continuous monitoring of the surgical procedure without disturbing the sterile field, in contrast to epicardial echocardiography.
Figure 39-10
Echocardiography. A. Long-axis view of a patient with a bicuspid aortic valve and an ascending aortic aneurysm. Note that the ascending aneurysm starts at the level of the sinotubular junction without dilation of the sinuses. B. In contrast, note the long-axis view of a Marfan disease patient with dilation of both the sinuses and the ascending aorta.

Because of its speed and wide availability, CT and computed tomographic angiography (CTA) are currently the most common diagnostic imaging methods for studying the aorta. With the advent of multidetector-row scanners, CT can provide the excellent spatial resolution necessary to image the aorta accurately with reasonable doses of iodinated contrast medium with a short acquisition time.87 Limitations on contrast use because of renal insufficiency or a history of allergy usually can be dealt with, particularly when there is an urgent need to make a definitive diagnosis. CTA combines a rapid bolus intravenous injection using a pressure injector with a timed breath hold and spiral CT acquisition during peak arterial opacification. Curved planar reformations and three-dimensional (3-D) reformations using maximum-intensity projection (MIP) and shaded surface display (SSD) after segmentation and editing of bony and other unwanted structures provide excellent visualization of the aorta and its branch vessels (Figs. 39-11 and 39-12).88 CTA (axial images) has the advantage of demonstrating aortic wall thickening, calcification, and luminal thrombus, thus displaying the true axial extent of the aneurysm, as the aortography or 3-D MIP and SSD images display only the enhancing lumen of the vessel. In addition, these wall parameters are important in establishing the etiology of the aneurysm and in decision making for further management. However, it should be noted that measurement based purely on the basis of axial images, which may not be orthogonal, can be misleading. Tortuosity of the aorta can lead to a false estimation of aneurysm size; therefore, 3-D SSD reconstruction has facilitated assessment of aneurysm size and extent significantly.
Figure 39-11
Computed tomography angiography of the thoracic aorta in a 59-year-old man. A 2.5-cm axial slice (A) showing a uniformly dilated ascending aortic aneurysm measuring 7.0 cm in diameter. Curved planar reformation through the aortic arch (B) shows extension of the aneurysm into the aortic arch and aneurysmal dilatation of the innominate artery. A volume-rendered image (C) displays rotation of the right sinus of Valsalva anteriorly. Both right and left coronary arteries are patent but ectatic.

Figure 39-12
Computed tomography angiography of the thorax with retrospective ECG gating in a 53-year-old man with a bicuspid aortic valve. A thick-slab volume-rendered image (A) shows aneurysmal dilatation of the ascending aorta with extension into the aortic arch. A volume-rendered image with transparent rendering of the flow lumen (B and C) allows visualization of the inner surface of the thoracic aortic aneurysm (B) and provides a good three-dimensional view of the bicuspid valve (viewed cranially).

CTA is the imaging study of choice in a suspected leaking aneurysm, in which rapid evaluation is imperative. In elective ascending and arch aneurysm repair, CTA is able to display the origins of aortic branches and their relation to the aneurysm, the proximal and distal extent of the aneurysm, and the adjacent thoracic structures, all of which are critical to operative planning. Currently, 64-bit scanners also are capable of imaging coronary arteries, eliminating the necessity for selective catheter injection for coronary assessment.
MRI is emerging as the premier imaging method for the diagnosis of diseases of the thoracic aorta in stable patients. MRI provides visualization in the sagittal and coronal planes and eliminates the need for noniodinated contrast and radiation exposure. Magnetic resonance angiography (MRA) of the thoracic aorta usually requires a combination of several available MRI methods, each of which has certain advantages and contributes to the diagnostic versatility of the technique. Contrast-enhanced MRA (CE-MRA) is the most widely used MRA method because it is rapid and robust, providing projection images of the aorta similar to those provided by conventional invasive angiography. Black-blood MRI permits assessment of the vessel wall by saturating the signal from the lumen. Phase-contrast imaging provides functional information about the flow. Gradient-echo cine images can demonstrate AR in the presence of disease of the ascending aorta. The field continues to develop, and many new MRA methods promise further improvements in acquisition time and resolution.89 However, MRI/MRA is less readily available and has limited utilization in acute aortic problems because of limited applicability to ventilated and invasively monitored patients. Indwelling pacemaker wires also frequently prevent its use.
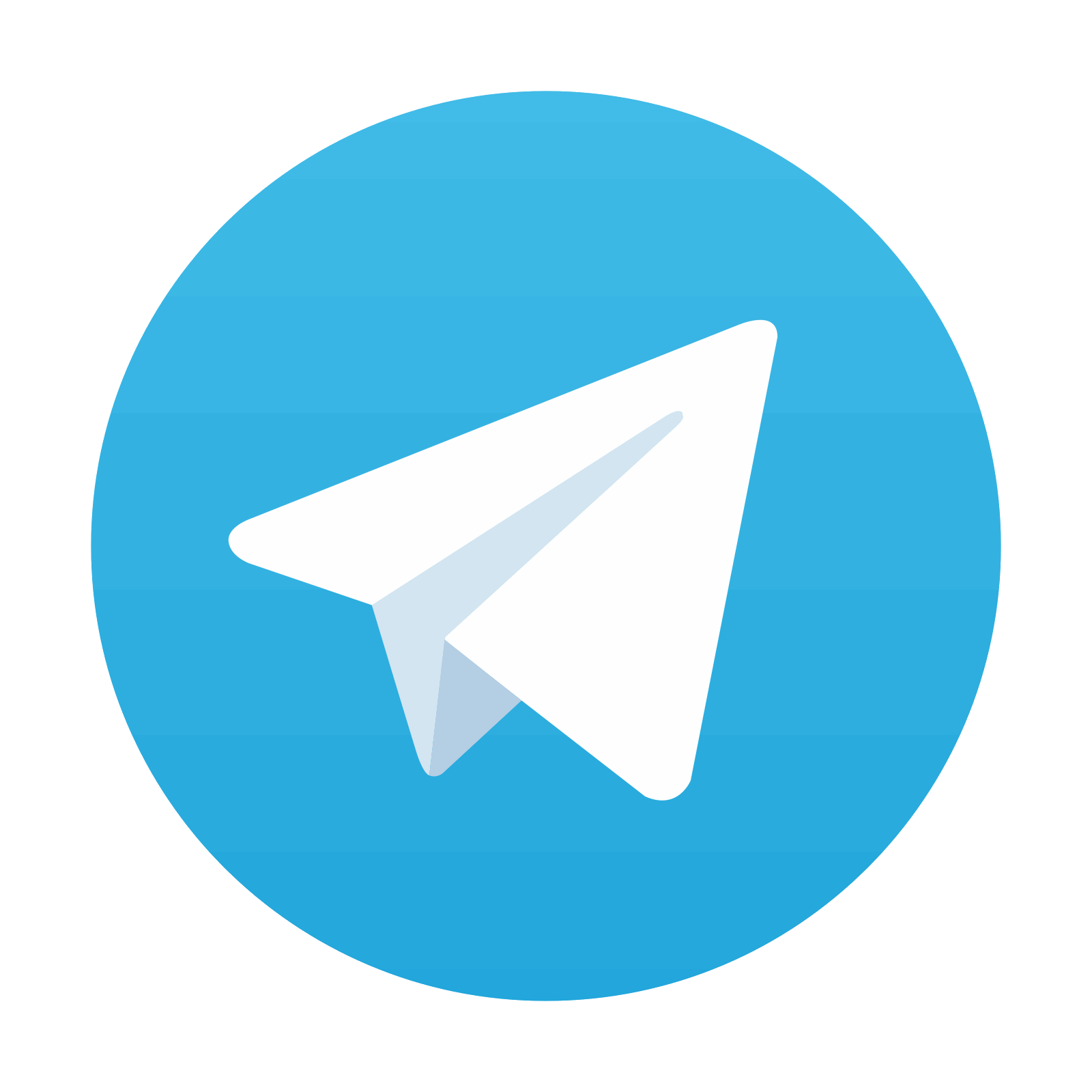
Stay updated, free articles. Join our Telegram channel

Full access? Get Clinical Tree
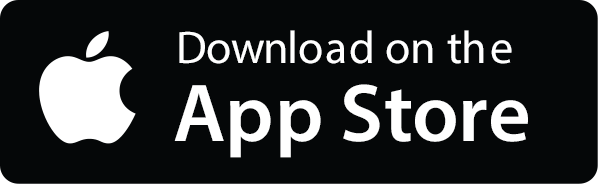
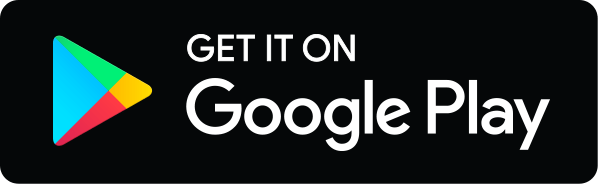