Grade
Symptom severity
Hemoglobin values
0
Within normal limits
12.0–16.0 g/dL for women and 14.0–18.0 g/dL for men
1
Mild
10 g/dL to levels within normal limits
2
Moderate
8.0–10.0 g/dL
3
Serious/severe
6.5–7.9 g/dL
4
Life threatening
<6.5 g/dL
The reported prevalence of anemia in patients with malignancies varies considerably according to different studies [10–14]. These discrepancies are related to the definition of anemia (any hemoglobin less than normal vs. moderate/severe), disease stage, and cancer subtype. Broadly, speaking, approximately 50% of patients with solid tumors have low hemoglobin, and at least 70% of patients with hematologic malignancies are anemic [14]. In some cancer subtypes, these numbers increase to about 90% [3]. The addition of cytotoxic chemotherapy further increases the risk of anemia [10]. Regardless of its precise frequency, anemia is clearly a very real issue that the majority of cancer patients—and their health care providers—will be forced to contend with at some point in the course of this illness.
Risk Factors
Using data from the prospective European Cancer Anaemia Survey (ECAS) , Barrett-Lee et al. analyzed data from 13,628 cancer patients initiating chemotherapy [15]. They identified five pre-treatment factors significantly associated with developing anemia during chemotherapy: initial Hgb ≤12.9 g/dL in females and ≤13.4 g/dL in males; presence of lung or gynecologic malignancy vs. gastrointestinal cancers; cancer at any site other than GI tract and female gender. In addition, platinum-based chemotherapy was associated with a higher incidence of treatment-related anemia [15].
Using the same data set, investigators specifically looked at patients with multiple myeloma and lymphoma (both Hodgkin’s and non-Hodgkin’s) [2]. They found that the following variables were associated with an increased likelihood of anemia: initial Hgb ≤13.3 g/dL in males and Hgb ≤12.7 in females; the presence of persistent or recurrent disease; female gender and use of a platinum agent for chemotherapy. Taken together, these results can aid practitioners in identifying patients at increased risk for anemia, facilitating careful monitoring and enabling the initiation of preventative therapies where appropriate.
Symptoms and Consequences of Anemia in Cancer Patients
Fatigue is the most ubiquitous symptom of anemia. Compared to the general population, patients with malignancies are more likely to be fatigued even in the absence of anemia [7].
The presence of anemia in cancer patients results in more severe fatigue compared to both the general population and their non-anemic counterparts [1]. In addition, cancer-related fatigue produces a greater impairment of everyday functioning than the equivalent degree of non-cancer-related fatigue. This means that anemic cancer patients are expected to have a substantially poorer quality of life [14, 16–18]. In fact, several studies have demonstrated a correlation between Hgb levels and quality of life [10].
As anemia becomes more severe, additional signs and symptoms develop, including headache, palpitations, shortness of breath, and tachycardia. Severe anemia may lead to heart failure, pulmonary edema, and cognitive impairment [10]. In the most extreme circumstances, critically low hemoglobin levels can lead to patient death, as may be the case in end-stage acute leukemia, aplastic anemia, and myelodysplastic syndrome [19]. Thus, persistent anemia results in both increased morbidity and increased mortality for cancer patients.
Physiologically, decreased hemoglobin levels may also promote tumor growth, impair response to treatment, and contribute to the selection of treatment-resistant clones. In a number of cancer subtypes, including cervical, head and neck, prostate, bladder, lung and lymphoma, the presence of anemia has been correlated with a worse outcome [20].
Up to 50–60% of advanced solid tumors contain regions of hypoxic tissue [21]. In large part, this occurs because tumors tend to be relatively poorly vascularized and frequently outgrow their blood supply [14]. However, in about a third of cases, tumor hypoxia is facilitated by decreased blood oxygen-carrying capacity due to anemia. Unlike normal tissue, the anomalous tumor vasculature is unable to accommodate for low hemoglobin levels by increasing local blood flow [21]. Thus, anemia both exacerbates and causes tumor hypoxia.
In an analysis of more than 100 patients with locally advanced cervical cancer, the presence of tumor hypoxia was significantly associated with increased extra-cervical extension and resistance to treatment [22]. Both disease-free and overall survival were reduced in patients with hypoxic tumors. Likewise, in a population of patients with advanced head and neck cancer, disease-free survival was 78% in patients with non-hypoxic tumors, compared to 22% in those with hypoxic tumors (p = 0.009) [23].
Although the mechanism by which a poorly oxygenated tumor environment results in more aggressive disease has not been fully elucidated, several important observations have been made. Firstly, hypoxia leads to upregulation of HIF-1∝, a key transcription factor that regulates the expression of more than 30 genes implicated in tumor progression [24]. Increased levels of HIF-1∝ result in angiogenesis via the upregulation of multiple growth factors including vascular endothelial growth factor (VEGF), platelet-derived growth factor-β (PDGF-β), and transforming growth factor β(TGF-β) among others [24–26]. It also causes downregulation of a number of angiogenesis inhibitors. The resulting increase in tumor vascularization is associated with increased tumor aggressiveness, tumor survival, and metastasis [25, 26].
Extreme hypoxia generates genomic instability, giving rise to genomic changes and clonal selection. These conditions generally result in a highly aggressive, multi-resistant phenotype [25, 26]. In particular, hypoxia appears to select for the highly deleterious p53 mutation [1, 5, 24]. The ensuing tumor expansion results in outgrowth of the local blood supply, leading to additional hypoxia in a self-perpetuating cycle.
Radiotherapy is an integral part of the treatment of many malignancies. Ionizing radiation causes the formation of DNA-damaging free radicals within tumor cells. Oxygen is a necessary component of the chemical reactions that enable free radicals to cause injury to DNA [27]. It has clearly been shown that a hypoxic tumor environment substantially reduces the effectiveness of radiation [27, 28]. In studies of patients with cervical cancer and patients with head and neck cancer, diminished tumor oxygen levels were associated with increased resistance to radiotherapy [28]. Conversely, increasing tumor oxygen levels both in vitro and in vivo is associated with an increase in tumor radiosensitization [27].
In addition to radiotherapy resistance, hypoxic tumor cells have also been shown to be less susceptible to chemotherapy-induced cell death [14, 26]. It is likely that HIF-1 ∝ upregulation plays an important role in chemotherapy resistance. It is believed that cytotoxic agents cause death of neoplastic cells via apoptosis. Induction of HIF-1 ∝ is associated with decreased levels of the pro-apoptotic proteins Bid, Bad, and Bax [24, 26]. HIF-1 inhibitors restore chemosensitivity in renal cell carcinoma, glioma, and pancreatic cancer cell lines [26].
As a means of chemoresistance, tumors frequently employ drug efflux pumps. These are membrane glycoproteins that propel foreign molecules out the cell interior. Overexpression of key efflux pumps such as MDR1 and MRP2 lead to the removal of chemotherapeutic agents from the tumor cells, resulting in subtherapeutic drug concentrations [29]. HIF-1 ∝ has been shown to upregulate both MDR1 and MRP2. Thus, through a host of mechanisms, hypoxic cell conditions lead to chemotherapy resistance [30].
Causes of Anemia in Malignancy
The mechanisms leading to and maintaining normal erythropoiesis are extremely complex [3, 19], and beyond the scope of this review. Broadly speaking, erythropoiesis requires appropriate nutrients, including iron, B12, and folate. Iron needs to be available to cells for hemoglobin synthesis rather than sequestered inside of storage cells such as macrophages. The bone marrow integrity must be intact and functional. Finally, the appropriate signaling mechanisms must be present in order to drive the process of red blood cell production within the bone marrow [19]. In cancer patients, any number of these factors can be impaired [1, 3, 19].
Patients with malignancies frequently have decreased serum iron levels [31]. This may be a direct result of the neoplasm itself, as in gastric or colorectal cancers that cause GI blood loss. Alternatively, the malignancy may interfere with the patient’s nutritional intake, as in esophageal and head and neck cancer that compromise the ability to eat. In other cancer types, anti-neoplastic treatment frequently causes mucositis, nausea, or constipation, making it difficult for patients to consume an adequate diet. In addition to decreased iron, these factors can also result in vitamin deficiencies such as B12 and folate [10]. Myelosuppressive treatment can lead to thrombocytopenia which predisposes the patient to blood loss. In some cases, frequent blood draws for laboratory testing further reduce the patient’s iron stores [1, 32].
While true iron deficiency may be a factor in the anemia of malignancy, functional iron deficiency (also called relative iron deficiency) is thought to play an even greater and more ubiquitous role [32, 33]. Hepcidin is the key hormone involved in iron trafficking [14, 32, 33]. This 25-amino acid polypetide is produced in the liver and, to a lesser extent, in monocytes. Hepcidin blocks the iron export channel, ferroportin, causing sequestration of iron within its target cells. Increased hepcidin levels result in decreased iron absorption from the gut, as well as iron trapping inside of reticuloendothelial cells. As a consequence, serum iron levels decrease and ferritin levels rise. While the patient may have adequate storage iron, the effect of elevated hepcidin is to significantly limit the availability of that iron for erythropoiesis [32]. Interleukin-6 (IL-6) levels are commonly increased in inflammatory and malignant states, and this cytokine has been shown to directly upregulate hepcidin expression [34]. Thus, IL-6 is felt to be a key mediator of functional iron deficiency. Investigators found that in ovarian cancer patients, IL-6 levels were inversely correlated with hemoglobin levels [35].
In cancer patients, the normal molecular events that control erythropoiesis are disrupted. Under routine conditions, red blood cell synthesis is regulated by physiologic stimuli that result in the production of erythropoietin (EPO) , a hormone that is primarily manufactured in the kidney, as well as smaller amounts in the liver [10]. EPO regulates the differentiation and survival of red blood cell precursors inside the bone marrow [32]. A primary downstream mediator of EPO is the GATA1 transcription factor that regulates expression of EPO-specific genes, such as the globin genes [36]. GATA1 effects are inhibited by GATA2 and NFκB [37] (Fig. 9.1).


Fig. 9.1
TNF α inhibits erythropoiesis by direct and indirect effects. In the indirect effect, TNFα activates the transcription factors NF-B and GATA-2, which were also reported as involved in Epo production inhibition by blocking HIF1α in vitro. Low level of Epo decreases the EpoR-mediated signaling pathways resulting among others, in the downregulation of GATA-1, and consequently in a possible deregulation of EpoR expression. TNFα was also reported as activating GATA-2 whose overexpression is known to prohibit erythropoiesis. The combined effects of TNFα result in the decrease in erythro-specific genes expression and hemoglobin production. (Reprinted from Morceau F, Dicato M, Diederich M. Pro-inflammatory cytokine mediated anemia: regarding molecular mechanisms of erythropoiesis. Mediators of Inflammation. 2009; (epub ahead of print). http://dx.doi.org/10.1155/2009/405016. Used with permission under the Creative Commons License: https://creativecommons.org/licenses/by/3.0/)
In the malignant state, the production of various inflammatory cytokines impedes the normal role of EPO on multiple levels. Tumor necrosis factor (TNF) ∝ is a key player in this inflammatory process [38, 39]. By upregulating GATA2, TNF∝ causes inhibition of HIF-1∝, which in turn results in decreased EPO synthesis. GATA2 also blocks the transcription of multiple EPO-specific genes. Among these genes is that of the EPO receptor itself, further limiting the bone marrow’s ability for erythropoiesis. TNF∝ also stimulates NFκB that further enhances the inhibitory effects on the expression of EPO-specific genes, leading to an additional decrease in red blood cell production.
EPO also assumes an integral role in erythropoiesis by enabling the survival of the red blood cell precursors, colony forming unit-E (CFU-E), and proerythroblasts [19]. These cells express large amounts of Fas receptors. When Fas binds to Fas ligand (Fas-L), apoptosis is triggered. EPO downregulates Fas receptors on CFU-E and proerythroblasts thereby promoting their survival. However, in the pro-inflammatory cancer environment, the balance is tipped toward apoptosis due to decreased EPO production [1, 19]. Furthermore, TNF∝, as well as other pro-inflammatory cytokines directly induce apoptosis in CFU-E and proerythroblasts, overcoming the effects of endogenous EPO [19, 38]. Thus, in cancer patients, various cytokines both impair EPO production and counteract the physiologic effects of EPO within the bone marrow. This sequence of events is thought to be especially pronounced in multiple myeloma, wherein the incidence of anemia at diagnosis is approximately 75% [2]. Multiple myeloma cells express Fas-L which is thought to bind to Fas receptors on red cell precursors, leading to apoptosis and a concomitant lack of erythrocyte differentiation [40].
In addition to these numerous impediments to red blood cell production, neoplasia can also be associated with increased erythrocyte destruction [1]. Immune system dysregulation may lead to hemolytic anemia. This can either be related to warm auto-antibody production (e.g., chronic lymphocytic leukemia) [41] or cold agglutinins (e.g., Waldentrom’s macroglobulinemia) [42]. Pure red cell aplasia, linked to thymoma and some lymphoid malignancies, is an autoimmune disorder involving the destruction of erythrocyte precursors in the bone marrow [43]. A rare but life-threatening example of immune dysregulation is hemophagocytic lymphohistiocytosis (HLH) whereby macrophages devour (phagocytose) erythrocytes, leukocytes, and/or platelets. This condition is most commonly associated with lymphoid malignancies but has been described in myeloid neoplasms and solid tumors, as well [44].
In cancer patients, direct bone marrow interference is another potential mechanism of anemia. Most commonly, this occurs via myelosuppressive or myelotoxic antineoplastic treatments such as chemotherapy and radiotherapy [10]. In some cases, the malignancy itself may occupy the bone marrow to a large enough extent that normal hematopoiesis is not feasible. This is often true of malignancies that “reside” in the bone marrow like leukemia and multiple myeloma. However, solid tumor metastasis to the bone marrow results in similar consequences [45].
Patient Evaluation
The primary focus of the anemia workup in a patient with a known malignancy is to determine, first, whether any component of the anemia is related to non-malignancy factors and second if any of these factors are reversible. This latter consideration is particularly important for maximizing patient quality of life and can also be helpful with determining the ability to tolerate intensive anti-neoplastic therapies.
Iron studies, including serum iron, total iron binding capacity (TIBC) , transferrin saturation, and ferritin should be checked. Vitamin B12 and folate levels should be assessed, as well. A thorough history and physical examination is critical to identify the possibility of ongoing blood loss. Examination of the peripheral blood smear, reticulocyte count, lactate dehydrogenase (LDH) levels, Coomb’s testing, and haptoglobin can aid in diagnosing hemolytic anemia. Renal function should be assessed, as renal impairment is a frequent cause of hypoproliferative anemia in cancer patients, particularly the elderly [1].
Nutritional deficiencies should be addressed. Functional iron deficiency is typically manifested by low serum iron, normal or low TIBC, low transferrin saturation, and elevated ferritin [14]. Treatment of functional iron deficiency is discussed in the next section. In some instances, malignancy-associated autoimmune hemolysis may respond to standard treatments such as high-dose glucocorticoids [41]. However, relapses are common and, eventually treatment of the underlying malignancy may be required.
Management of Anemia in Malignancy
For many years, red blood cell transfusion was the only means available to increase hemoglobin in anemic cancer patients [1]. There are a number of risks associated with the administration red blood cell transfusions. Many of these concerns are not specific to patients with malignancy. These include viral and bacterial infections, acute and delayed hemolytic transfusion reactions, febrile nonhemolytic transfusion reactions, transfusion-associated lung injury (TRALI) , volume overload, post-transfusional purpura, iron overload, and anaphylaxis [1]. In general, however, transfusions are considered reasonable and safe when administered with appropriate procedural techniques and close patient monitoring [46].
Interestingly, an association has been suggested between the receipt of multiple red blood cell transfusions and cancer recurrence [47]. Prospective studies have shown a correlation between the number of red blood cell transfusions and colorectal cancer recurrence [1, 47]. This is thought to be on the basis of weakened immune responsiveness as a consequence of depressed cytotoxic T-cell, natural killer (NK) cell, and monocyte function [48]. It would appear that this immunosuppression is related to the presence of leukocytes in the transfused products. The use of leukoreduction techniques significantly lowers the number of donor white blood cells per unit of blood by 99.99%. This substantially reduces the immunological issues associated with leukocytes [49]. In the United States, more than 80% of institutions dispensing blood products have a policy of universal leukoreduction [50].
When transfusing patients with malignancy, the optimal target hemoglobin remains undefined. Studies in the critical care setting have suggested equivalent and possibly improved outcomes with more restrictive transfusion thresholds wherein patients are given blood if Hgb is <7 g/dL, and the target Hgb is 7–9 g/dL [51]. However, it is difficult to generalize these findings to the more chronic anemia associated with malignancy where ongoing, outpatient quality of life becomes an important concern. At the same time, as noted above, more frequent transfusions imply an added risk of complications and possibly an adverse effect on cancer itself. Therefore, national guidelines generally recommend individualizing the decision to transfuse for Hgb between 7 and 10 g/dL according to the particular patient and the specific clinical circumstances [52].
Recombinant human EPO or erythropoietin-stimulating agents (ESAs) were first introduced for the treatment of anemia in patients with end-stage renal disease [10]. ESA use in these patients resulted in a significant reduction in the number of red blood cell transfusions [53]. These impressive data led to the investigation of ESAs in malignancy-associated anemia. A number of large, randomized trials conducted during the 1990s through the early 2000s demonstrated a significant reduction in transfusion requirements for cancer patients undergoing chemotherapy who were treated with ESAs [10]. A meta-analysis of 25 randomized controlled trials (RCTs) comprising 3069 patients confirmed that ESA use reduced the relative risk of red blood cell transfusion in patients with malignancy-associated anemia undergoing chemotherapy (RR 0.67, 95% CI 0.62–0.73) [54]. Of interest, the benefit was more marked for patients with solid tumors compared to hematologic malignancies, possibly owing to the increased likelihood of bone marrow involvement in the latter group [54].
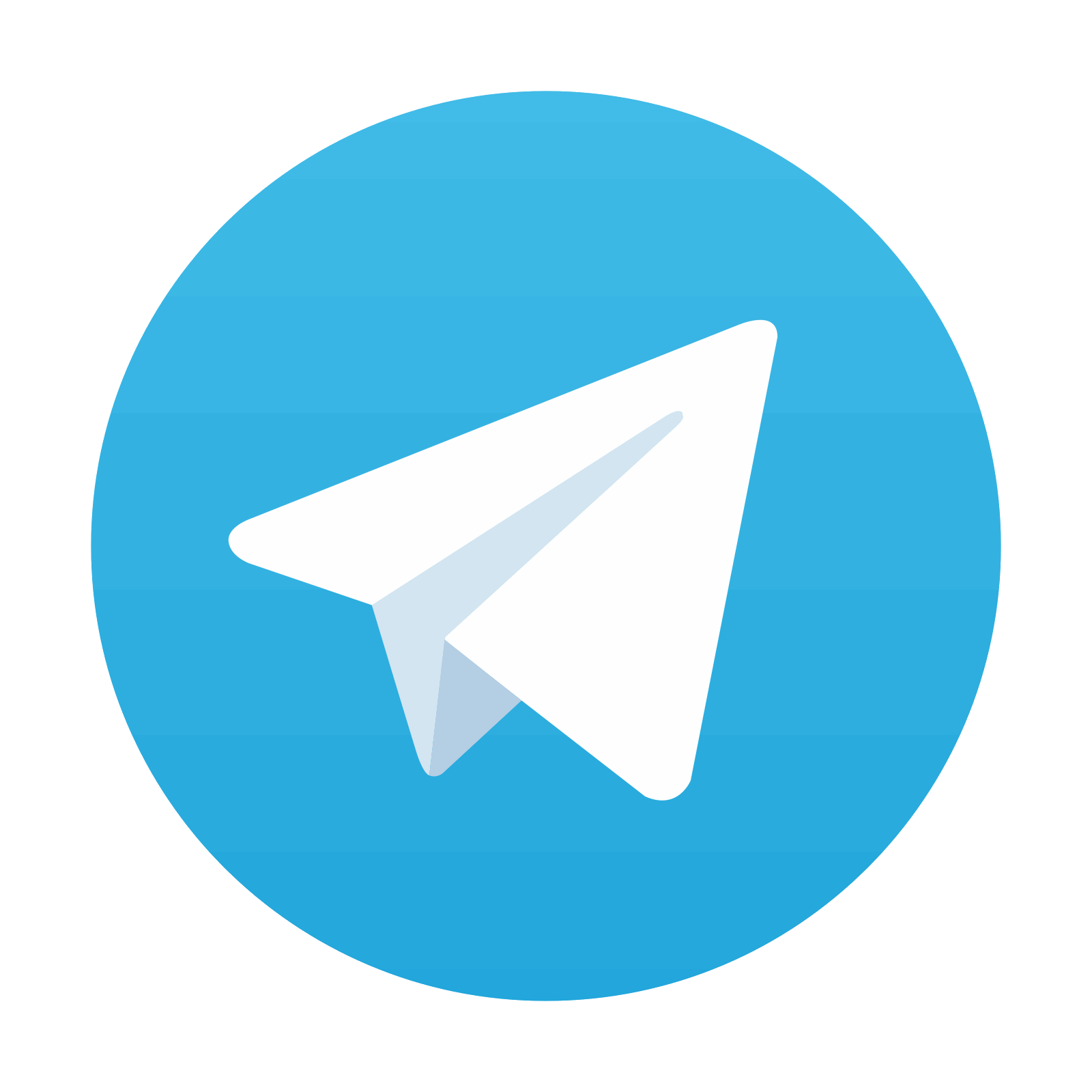
Stay updated, free articles. Join our Telegram channel

Full access? Get Clinical Tree
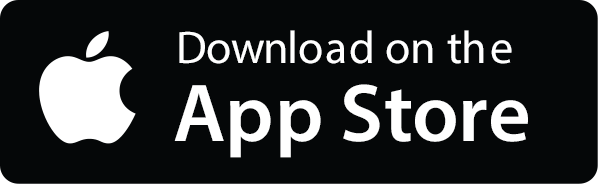
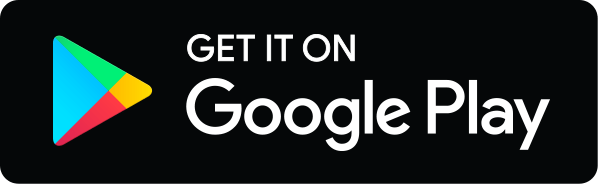