Acute blood loss anemia (portal hypertension-related)
Esophageal varices
Gastric varices
Rectal varices
Chronic blood loss anemia
Portal hypertensive gastropathy
Portal hypertensive enteropathy
Portal hypertensive colopathy
Gastric-antral vascular ectasia
Acute hemolytic anemia
Diffuse intravascular coagulation
Drug-induced hemolysis
Chronic hemolytic anemia
Splenic sequestration
Hemolytic anemia associated with copper accumulation
Bone marrow insufficiency
Chronic alcohol abuse
Viral hepatitis-induced aplastic anemia
Drug-induced
Pegylated interferon therapy
Ribavirin therapy
Micronutrient and vitamin deficiencies
Iron deficiency
B12 deficiency
Folate deficiency
Vitamin K deficiency, leading to coagulopathy

Fig. 7.1
Anemia algorithm
Portal Hypertension-Related Anemia
The development of portal hypertension in liver disease predisposes to both acute and chronic gastrointestinal bleeding. In cirrhosis , or end-stage liver disease, portal hypertension may develop as a consequence of increased resistance to blood flow through the liver secondary to architectural distortion from fibrous tissue and regenerative nodules, and is defined by a hepatic venous pressure gradient (HVPG) >5 mmHg [1]. Active intrahepatic vasoconstriction also plays a role, contributing 20–30% of intrahepatic resistance, mostly due to reduced endogenous production of nitric oxide [1]. Portosystemic collaterals, most commonly gastroesophageal varices, are formed in an attempt to decompress the elevated pressures in the portal system. This collateralization is insufficient given a concurrent increase in portal venous inflow from splanchnic arteriolar vasodilation as well as higher resistance noted in the collaterals [2]. In effect, portal hypertension is thought to occur as a result of both an increase in portal resistance as well as portal inflow.
Gastroesophageal varices are the clinically most relevant portosystemic collaterals as their rupture leads to acute anemia through variceal hemorrhage, the most common fatal complication of cirrhosis [1]. These collaterals are present in about 50% of patients with cirrhosis, and correlate with increased severity of liver disease in regards to prevalence and risk of hemorrhage [1]. Variceal hemorrhage occurs at an annual rate of 5–15% with the most important predictor being the size of the varices, with large varices carrying the highest risk at 15% [1]. Acute hemorrhage may cause severe hypovolemia and subsequent secondary iron-deficiency anemia, especially in recurrent bleeding due to depletion of iron stores [3]. Variceal hemorrhage is an emergency and requires prompt volume resuscitation and blood transfusion to maintain a hemoglobin of ~8 g/dL to correct the acute anemia [1]. Medical therapy includes administration of vasoactive drugs such as terlipressin or octreotide [3]. Endoscopic therapy includes band ligation of esophageal varices as well as tissue adhesive obturation of gastric varices [1]. Details regarding the management and treatment outcomes of varices are beyond the scope of this chapter.
Patients with cirrhosis may develop chronic bleeding throughout the gastrointestinal tract from portal hypertensive gastropathy (PHG) , portal hypertensive enteropathy, portal hypertensive colopathy, or gastrointestinal vascular ectasia (GAVE). Chronic gastrointestinal bleeding refers to a reduction in hemoglobin of 2 g/dL within a 6-month period without evidence of acute bleeding or non-steroidal anti-inflammatory drug use [4], though other definitions including the presence of iron-deficiency anemia with a positive fecal occult blood test have also been used [5]. These patients with chronic gastrointestinal blood loss may similarly develop secondary iron-deficiency anemia. Among these etiologies, PHG is the most common, and is recognized endoscopically as a mosaic-like pattern called “snake-skin mucosa ” with or without red spots [6]. Portal hypertensive enteropathy and colopathy describe similar changes in the small bowel and colonic mucosa, respectively. PHG is most commonly found in the proximal stomach (fundus and body) [6]. The prevalence of PHG in patients with cirrhosis ranges from 20 to 98%, with this variation in the literature secondary to multiple factors including lack of uniform diagnostic criteria and classification, as well as different patient populations [4]. Several studies demonstrate a higher prevalence of PHG in patients with more severe liver disease and other complications of portal hypertension, such as esophageal varices [4]. Some studies suggest that the prevalence of PHG may increase once esophageal varices are obliterated, though this is controversial [6].
Many patients with PHG are asymptomatic, though up to 60% exhibit symptoms related to chronic gastrointestinal bleeding [4]. Classification of PHG is done based on severity, with most experts recommending a two-category system [7]. Mild is defined as only noting the snakeskin mosaic pattern; severe is defined as snakeskin mosaic pattern with flat or bulging red or black spots, or related to acute GI bleeding [7]. Acute GI bleeding is fairly uncommon in PHG, ranging from 2 to 12%, and is identified endoscopically [4].
Treatment options are targeted according to the presentation of the patient in terms of the rate of bleeding and related symptoms. It is not uncommon to identify asymptomatic incidental PHG during esophageal varices screening in patients with cirrhosis . At this time, primary prophylaxis of GI bleeding in patients with PHG has not been assessed thoroughly and is not recommended routinely. However, in patients with severe PHG but without varices or active bleeding, prophylaxis with non-selective β-blockers may be considered, though this approach is controversial [4]. For patients where chronic blood loss is of concern, such as those that present with PHG and iron-deficiency anemia, it is important to exclude other causes of iron-deficiency anemia. Iron replacement therapy should be started in all patients with iron-deficiency anemia caused by PHG, either oral preparation or intravenous dependent on the iron deficit [4]. The use of nonselective β-blockers may reduce blood losses in patients with a concern of chronic bleeding secondary to PHG, and therefore should be implemented in this circumstance [5]. For patients with acute GI bleeding secondary to PHG, again, it is essential to exclude other causes of bleeding. Early and aggressive generalized support is essential including blood transfusion to a hemoglobin goal of ~8 g/dL, antibiotics such as IV quinolone or third-generation cephalosporin for spontaneous bacterial peritonitis prophylaxis, and vasoconstrictor therapy with terlipressin, somatostatin, or somatostatin analog such as octreotide [4]. Once the diagnosis of actively bleeding PHG is confirmed endoscopically, argon plasma coagulation (APC) may be applied through an endoscope in situations where a single or a limited number of culprit oozing lesions are apparent on a patient by patient basis [4]. Initiation of non-selective β-blockade should also be considered given evidence of control of acute bleeding within 3 days in 13 (93%) of 14 patients in one small study [8]. Vasoconstriction with octreotide infusion for 48 h has also demonstrated efficacy to stop bleeding [9]. Patients with refractory chronic bleeding due to PHG include those with the disease that remain transfusion dependent despite iron therapy and β-blockade [4]. Patients with refractory acute bleeding due to PHG include those that have recurrent hematemesis despite treatment, such as with vasoactive medications, and/or a 3 g hemoglobin decline without transfusion or an inadequate hemoglobin rise after transfusion [10]. In these cases, rescue therapies such as shunt surgery or TIPS may be considered, as both have been shown to be effective [10]. Although repeated application of APC appears as an attractive option, there are currently insufficient data to recommend it, though this can be considered on a patient by patient basis [4].
One of the major differential diagnoses for acute or chronic GI bleeding that may appear similar to PHG is GAVE, or “watermelon stomach” [4]. GAVE also appears as flat red spots, but in streaks of erythema that appear to be emanating from the pylorus [4]. GAVE is thus located in the distal stomach (antrum), whereas PHG is located in the proximal stomach (fundus and body), which may help distinguish the disorders [4]. GAVE similarly typically presents with chronic GI blood loss and iron deficiency and is a relatively rare cause of acute hemorrhage in patients with cirrhosis [4]. It is also seen in other diseases such as in patients with chronic renal disease, bone marrow transplantation, as well as autoimmune disease such as scleroderma [4]. Interestingly, there does not seem to be a distinct relationship between GAVE and either portal hypertension or cirrhosis , but it is encountered in both clinical situations [4]. The differentiation between GAVE and PHG is important given the different treatment paradigms. GAVE is treated with endoscopic thermoablation with APC as the method of choice, as this has been shown to be effective across multiple studies [11]. Large case series using APC have reported efficacy >90% with no further need for blood transfusions with repeated sessions every 2–6 weeks until adequate eradication [11]. Despite the efficacy of APC in the short term, approximately 30–60% of cases may have recurrent bleeding in the long-term, as thermal treatment effects the superficial epithelium while GAVE commonly involves deeper structures such as in the submucosa that likely predispose to recurrent bleeding [11]. Band ligation has also been used to treat GAVE, as this method may more reliably eradicate the deeper vascular structures, and in small studies has been shown to be at least as effective as APC [11]. More recent studies have also evaluated radiofrequency ablation for the treatment of GAVE through paddle applicators with promising results [11]. Given the nature of the method, uniform tissue penetration is easier to obtain with the RFA paddle catheter and operator-dependent effects are minimized. Cryotherapy with nitrous oxide has also been successfully utilized to treat GAVE; a study by Cho and colleagues evaluated 12 patients, eight of whom had failed prior APC treatment, and achieved complete or partial response in all patients without complications [12]. As a last resort, surgical antrectomy has also been shown to be effective and safe for refractory GAVE for otherwise well-compensated cirrhotic patients that fail repeated endoscopic management [11].
Hypersplenism resulting from portal hypertension represents another mechanism of anemia in liver patients. This portal hypertension may be secondary to cirrhosis , or liver disease complicated by portal vein or splenic vein thrombosis. Extravascular hemolysis occurs in the spleen due to macrophages in the setting of red blood cell stasis and trapping [13]. Interestingly, it is the spleen size and not the degree of portal hypertension that determines the extent of erythrocyte sequestration [13]. The degree of anemia that results from this hemolysis mechanism is variable, and is dependent on the ability to counterbalance red blood cell destruction with an increase in production. The main treatment for anemia secondary to hypersplenism depends on the underlying cause. For hypersplenism secondary to cirrhosis with adequate hepatocellular reserve and splenic vein thrombosis, splenectomy may be considered in severe cases after medical efforts are exhausted, though caution is necessary given significant surgical risk [14]. In addition, partial or complete splenic artery embolization has been utilized to treat hypersplenism causing severe thrombocytopenia , and is generally a safer option [15].
Alterations of Red Blood Cell Membrane
Acquired alterations of the red blood cell membrane due to liver disease are associated with variable degrees of hemolysis. The four major variant red blood cell types that result from chronic liver disease include acanthocytes (spur cells), codocytes (target cells), echinocytes (burr cells), and stomatocytes. These alterations of red blood cell membrane occur due to changes in the concentration and ratio of cholesterol and phospholipids in the cell membrane [16]. Of these, acanthocytes are typically associated with the greatest degree of hemolysis. When occurring in abundance this is referred to as spur cell anemia [17]. This occurs because the lipid composition of the red blood cell membrane of these cells renders them more susceptible to splenic entrapment and destruction, and is typically found in alcoholic cirrhosis , though has been noted in other etiologies of cirrhosis and in neonatal hepatitis [17]. The early splenic consumption of red blood cells can be quite significant; a study by Jandl in 1955 found that red blood cell lifespan was diminished by about 50% in patients with cirrhosis [18]. On the other hand, target cells do not appear to have a diminished lifespan, and may actually be protected from the otherwise splenic conditioning process that leads to consumption [16]. Echinocytes have serrated edges over their entire cell surface and develop this distinct shape due to abnormal high-density lipoprotein binding on membrane receptors; these cells are associated with hemolysis to less of a degree [19]. Stomatocytes, produced by bending or folding of the plasma membrane inwards secondary to membrane compositional changes, appear with mouth-shaped areas of central pallor [20]. This red blood cell variant is also implicated in hemolysis to varying degrees. The resulting hemolytic process associated with many of these acquired alterations of red blood cell membrane in liver disease depend both upon the extent of membrane metabolic changes as well as the degree of hypersplenism and splenic macrophage activity.
Viral Hepatitis-Associated Aplastic Anemia
Aplastic anemia is a rare disorder characterized by pancytopenia from a loss of pluripotent hematopoietic stems cells in the bone marrow in the absence of infiltrative disease [21]. Among causes of aplastic anemia , viral hepatitis is an uncommon cause, encompassing 2–5% of documented aplastic anemia cases in the West and a slightly higher proportion in the Far East [22]. This disease typically afflicts young men and adolescent boys within 2–3 months of acute hepatitis and is usually fatal if left untreated due to rapid and severe marrow failure [22]. Viral hepatitis-associated aplastic anemia cases secondary to hepatitis A, hepatitis B, hepatitis C, hepatitis E, cytomegalovirus, Epstein-Barr virus , parvovirus B19, echovirus, GB virus C, transfusion transmitted virus, and SEN virus have been reported; however, the etiology for most cases remains a mystery [23]. In this patient group without identified virus most also lack toxins, drugs, or blood transfusions exposure [24]. The suggested pathogenesis is due to the induction and activation of T-lymphocytes from viruses and/or antigens within the bone marrow of afflicted patients through the release of interferon-ɣ and potentially other cytokines, ultimately resulting in the apoptotic death of marrow progenitor cells [21]. Furthermore, the development of aplastic anemia after liver transplantation for fulminant non-A, non-B, non-C hepatitis in young patients is not uncommon; the cause of fulminant seronegative hepatitis in such cases is unknown [25].
The clinical presentation of viral hepatitis-associated aplastic anemia is variable, including symptoms and signs related to pancytopenia, such as fatigue and pallor due to anemia, hemorrhagic complications due to thrombocytopenia, and infectious complications due to neutropenia. The diagnosis is suggested by laboratories including the complete blood count revealing pancytopenia and absolute reticulocytopenia. Bone marrow biopsies typically clinch the diagnosis, revealing marked hypocellularity of precursor cells with residual cells appearing normal, as well as absence of malignant infiltrates [26].
Treatment for the condition requires urgent evaluation and hematology consultation. Blood and platelet transfusions should be utilized selectively to limit sensitization, and require irradiation, CMV negativity, and should not be from family members. The two major therapeutic options include hematopoietic stem cell transplantation and immunosuppressive therapy, with hematopoietic stem cell transplantation from an HLA-matched sibling donor being the treatment of choice [23]. A recent meta-analysis found that the mean survival at 5 years for hematopoietic stem cell transplantation was 82% [23]. The same meta-analysis calculated the mean response rate to immunosuppressive therapy at 70% [23]. The details regarding both treatment modalities are beyond the scope of this chapter.
Alcoholic Liver Disease and Anemia
Alcohol consumption is implicated in the development of chronic liver disease and may contribute to anemia through a myriad of effects in the liver patient. Excessive alcohol use may predispose to hemorrhagic gastritis or peptic ulcer disease, and in liver patients that develop portal hypertension from severe alcoholic hepatitis or from alcoholic cirrhosis, may cause portal hypertension related blood loss from bleeding mechanisms described earlier in this chapter. Anemia secondary to vitamin B12 deficiency and/or folate deficiency often found in alcoholic liver disease is described later in the nutrient deficiency portion of this chapter. Of note, the macrocytosis seen in excessive alcohol use is not always related to folate and vitamin B12 deficiency, as this phenomenon is also noted in the absence of these deficiencies [27]. The treatment of alcohol-induced macrocytosis is abstinence. Alcohol is also known to have a direct toxic effect on the bone marrow, resulting in diminished erythropoiesis, which is a further burden of anemia in the liver disease patient [28]. Hemolysis may occur in patients with advanced chronic liver disease due to alcohol through portal-hypertension-related hypersplenism discussed earlier in this chapter. Spur cell anemia has also been noted in patients with alcoholic cirrhosis; this type of hemolytic anemia described previously is caused by a red blood cell membrane defect resulting in spiculated erythrocytes leading to shortened survival [29]. Zieve’s syndrome is an uncommon form of acute Coombs’ negative hemolytic anemia that was first described as a triad of jaundice, hyperlipidemia, and alcoholic steatohepatitis in the alcoholic liver disease patient [30]. This acute syndrome is a relatively rare phenomenon first described by Leslie Zieve in 1957 [30]. Patients are noted to have right upper quadrant pain, low-grade fever, and loose stools in addition [30]. Zieve postulated that hyperlipidemia, particularly lipid lysolecithin, was responsible for the membrane instability that resulted in hemolysis [30]. At this time, the exact underlying mechanism for Zieve’s syndrome is unknown.
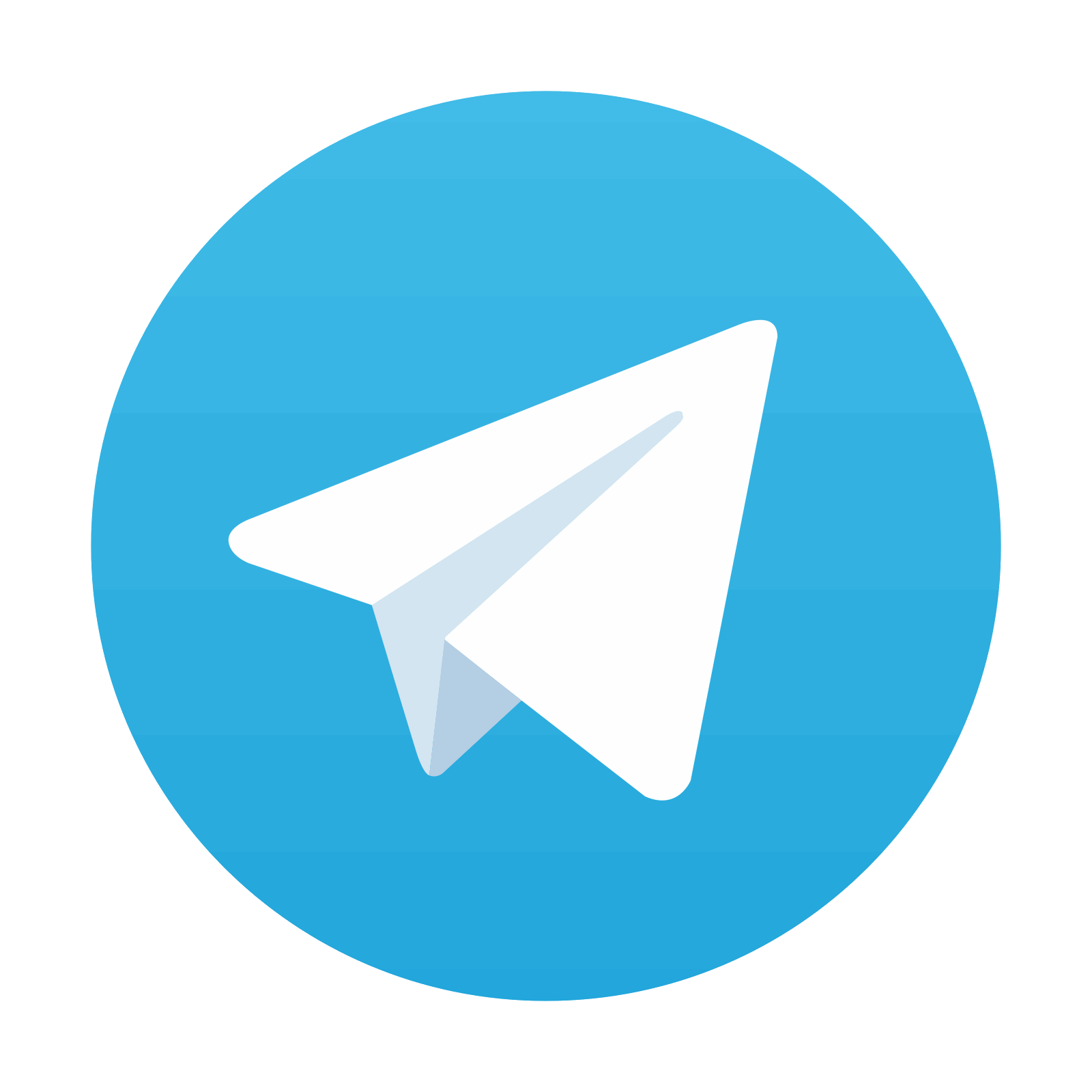
Stay updated, free articles. Join our Telegram channel

Full access? Get Clinical Tree
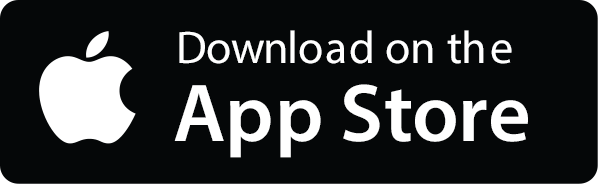
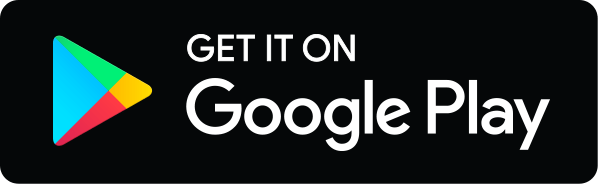