Hepatic
Renal electrolyte glucose
Cardiovascular
Pulmonary
Neurologic
Haematologic
Decreased metabolic function
Acute kidney injury
Vasodilated state
Aspiration risk
Hepatic encephalopathy
Bleeding and thrombosis
Decreased gluconeogenesis
Hepatorenal syndrome
Hyperdynamic cardiac function
Hepatopulmonary syndrome
Cerebral oedema
Unreliable INR
Decreased lactate clearance
Hyponatraemia
Cirrhotic cardiomyopathy
Compression atelectasis
Intracranial hypertension
Decreased factor synthesis
Hyperbilirubinaemia
Hypoglycaemia
Drug-induced hypertension
Pleural effusions/hepatic hydrothorax
Thrombocytopaenia and platelet dysfunction
Varices
Insulin resistance
Portopulmonary hypertension
Acute respiratory distress syndrome
Dysfibrinogenaemia
Portal hypertension
Ascites
9.1.8 Preoperative Assessment
Routine tests of interest for the anaesthesiologist include: complete blood count; urea, creatinine, and electrolytes; liver function studies, including albumin and transaminases; coagulation studies, including PTT and INR; virology studies, including hepatitis A, B, and C, cytomegalovirus, Epstein-Barr virus, herpes simplex virus, HIV, varicella zoster virus; blood group and antibody screening; arterial blood gas, if oxygen saturations are low; electrocardiogram; chest X-ray, posteroanterior and lateral; transthoracic echocardiogram, including estimation of pulmonary artery pressures; and pulmonary function studies in smokers or those with a history of pulmonary disease. Additional investigations are typically undertaken at the discretion of the preoperative assessment physician, although many centres use local protocols or guidelines to guide practice [28, 53–57].
In our institution the pre-anaesthetic evaluation is performed in two stages. In the first stage, all LT candidates are examined by the anaesthesiologist; then they are evaluated by a multidisciplinary team (including haematologists, surgeons, anaesthesiologists, cardiologists, specialty nurses, psychologists, transplant coordinators); the patients are then placed in the waiting list.
The assessment of any neurological deterioration occurring after the initial first-stage evaluation is imperative; also signs of progressive metabolic acidosis, infection or sepsis, cardiovascular instability, pulmonary infection, and severe coagulopathy need to be corrected and treated.
The second-stage evaluation is performed immediately before surgery, for the anaesthetic planning. Patients older than 50, with clinical or family history of heart disease or diabetes, undergo evaluation for CAD with emission computed tomography using technetium-99. In case of CAD the patients undergo arteriography of the coronaries, or, if the examination is considered dangerous, a computed tomography angiography may be preferred. When needed, the patients undergo percutaneous treatment with angioplasty or stenting of the coronary lesions to prepare them for transplant. In case of elevated pulmonary pressures, the patients undergo right-heart catheterization.
9.2 Intraoperative Monitoring and Management of Liver Transplant Recipients
9.2.1 Monitoring
Routine monitoring includes ECG, oxygen saturation, and invasive blood pressure before induction of anaesthesia. Invasive arterial blood pressure monitoring is essential for providing continuous monitoring and evaluate frequent haemodynamic changes. Pulmonary artery catheters, oesophageal Doppler, pulse contour analysis (PiCCO), lithium dilution technique (LIDCO) and transoesophageal echocardiography (TEE) are all used in different centres. TEE has been used only sporadically as a cardiovascular monitor during orthotopic liver transplant. This is most likely because of concerns regarding the risk of provoking haemorrhage of the gastric or oesophageal mucosa in patients with portal hypertension and impaired coagulation [58]. Despite these concerns, in some centres, the use of this technique is routinely used to improve the monitoring of volume status and myocardial function and to assess the response to cardioactive drugs [59]. Intraoperative TEE may provide additional critical information, such as identification of intracardiac thrombi [60] or complications related to TIPSS [61]. Recently, a report presented a case of postreperfusion graft congestion; TEE revealed a haemodynamically significant thrombotic stenosis of the IVC [62]. The PiCCO technology to assess cardiac output is another less invasive monitoring technique compared to pulmonary arterial catheterization. This device enables the assessment of intravascular blood volume and therefore guides a correct intraoperative fluid management [63]. Coagulation monitoring is best provided by thromboelastography (TEG) although platelet count, activated prothrombin time, thromboplastin time, fibrinogen, and fibrinogen decay products can also supply information and guide reintegration therapy [64, 65].
9.2.2 Anaesthesia Management: Induction and Maintenance
Anaesthesia typically is performed in a rapid sequence, and ventilation is started immediately. A steep drop in SpO2 can occur rapidly after anaesthesia induction in patients with pre-existing hypoxemia and ascites [66].
Generally the patients are ventilated with anaesthesia-integrated machine, with minimal flow technique; a balanced technique is used for maintenance of anaesthesia, with a volatile agent, narcotic and non-depolarizing muscle relaxant [13]. The lungs are ventilated using a protective strategy with a combination of low tidal volumes (6–8 mL/kg), a positive end expiratory pressure of 6–8 cm H2O and regular recruitment manoeuvres [67, 68]. The effects of the anaesthetic technique on patient outcome are unknown. It has been suggested that isoflurane offers advantages over sevoflurane and desflurane in terms of its impact on splanchnic blood flow; however, the evidence supporting this assertion is weak. Investigations addressing the effects of desflurane had conflicting results. In an animal study, desflurane has been shown to decrease hepatic blood flow in a dose-dependent manner at concentrations up to 1 MAC. However, a human study, although excluding patients with hepatic diseases and achieving non-statistically significant results, has shown increased hepatic blood flow using desflurane compared to isoflurane [69]. Another study comparing the effects of desflurane and sevoflurane in terms of hepatic blood flow and hepatocellular integrity showed that both agents preserved well the hepatic functions, but decreased splanchnic perfusion and oxygen delivery to the liver. The increased metabolism of sevoflurane which is a hundred times that of desflurane is not known to have detrimental effects on the liver [70]. Paralysis is titrated to achieve suppression of the neuromuscular function, assessed using a peripheral nerve stimulator. Cisatracurium may be the preferred neuromuscular blocking agent in patients undergoing liver transplantation because of its organ-independent elimination and diminished histamine release [71, 72].
9.2.3 Intraoperative Management: Preanhepatic Stage
The preanhepatic phase begins with the surgical incision and ends with the cross-clamping of the portal vein, the suprahepatic inferior vena cava, the infrahepatic inferior vena cava, and the hepatic artery. This phase involves dissection and mobilization of the liver and identification of the porta hepatis.
With abdominal incision and drainage of ascites, hypovolaemia typically occurs. During this phase of surgery, ascites is drained, adhesions are taken down, the vascular and biliary structures are identified, and the diseased liver is mobilized. Blood loss during this phase of surgery may be significant. Previous abdominal surgery (including hepatic resection or liver transplant) or previous intra-abdominal sepsis, including spontaneous bacterial peritonitis, may make this phase of surgery more difficult and the bleeding more significant. Coagulation status should always be monitored by inspection of the surgical field. Patients with active sites of bleeding should be transfused with fresh-frozen plasma, packed red blood cells, prothrombin complex concentrates, antifibrinolytic, recombinant factor VIIa (rFVIIa) or platelets [73, 74]. In addition, desmopressin can be considered for patients with concomitant renal dysfunction and uraemic bleeding. Measurements from thromboelastography (TEG) or rotational thromboelastometry (ROTEM) assess the viscoelastic properties of a whole blood sample as a function of time to reflect the function and interaction of coagulation factors, blood cells and platelets. These measurements detect a hypercoagulable state and distinguish hyperfibrinolysis from other causes of coagulopathy, such as factor depletion or thrombocytopaenia, to offer a composite picture of the clotting cascade [74–93]. The maintenance of a low positive CVP during parenchymal transection is desirable to reduce hepatic venous bleeding and to allow an easier control of venous injury, because a low CVP can be translated into a low pressure in the hepatic veins and sinusoids. However, the risks of a low CVP include cardiovascular instability, air embolism [91, 94, 95] and adverse outcomes including acute renal failure. Thus, maintenance of low venous pressures must be balanced against adequate perfusion of the organs. The use of terlipressin to achieve these goals has been investigated, demonstrating a reduced portal venous pressure whilst maintaining the renal perfusion [96]. However, further studies are required to evaluate its safety in terms of splanchnic perfusion and post-transplantation portal venous blood flow [97].
Hyponatraemia should not be corrected rapidly. A perioperative rise of 21–32 mEq/L in the serum sodium level was associated with central pontine myelinolysis in one report, whereas an increase of 16 mEq/L was not [98]. Citrate intoxication, ionized hypocalcaemia resulting from the infusion of citrate-rich blood products in the absence of hepatic function, is avoided by the administration of calcium chloride. Ionized hypomagnesaemia also results from citrate infusion, but values of ionized magnesium gradually return to normal after graft reperfusion [99].
9.2.4 Intraoperative Management: Anhepatic Stage
The anhepatic stage begins with the occlusion of vascular inflow to the liver and ends with graft reperfusion. There are two major approaches to the removal of the diseased liver: piggyback technique and venovenous bypass [100].
Piggyback technique uses only partial or side clamping of the IVC, with preservation of some caval flow. This technique causes less haemodynamic compromise and leads to shortened operative and warm ischaemia times, reduced red blood cell and blood product use, and similar graft function and survival outcomes [101]. Venovenous bypass (an extracorporeal circuit to bypass the IVC cross-clamp and return venous blood from the portal and lower body districts) [102] attenuates the decrease in preload, improves renal perfusion pressure, lessens splanchnic congestion and delays the development of metabolic acidosis [103]. The use of VVB is not without risk; in fact, air embolism, thromboembolism and incidental decannulation may be fatal or result in significant morbidity. VVB is not uniformly used at all centres [104–106]; a reasonable approach is to consider the use of venovenous bypass only if trial clamping of the IVC causes profound hypotension associated with a dramatically reduced cardiac index, which does not respond to inotropes and volume loading, or if anatomy or surgical expertise precludes the piggyback approach. A flow rate of 2–3 L/min is targeted, and depending on pre-existing coagulopathy, a small dose of heparin may be given to reduce the risk of in-circuit clot formation. With the exclusion of the native liver from the patient’s circulation, there are profound effects on the patient’s metabolic state. The most significant change during the anhepatic phase is the loss of the lactate-metabolizing capacity of the liver and a rise in plasma lactate and decrease in plasma pH [107]. This lactic acidosis is exacerbated when the graft liver is reperfused, and thus, many practitioners choose to treat the acidosis during the anhepatic phase to reduce the risk of severe acidosis with reperfusion [107]. In patients with profound preoperative liver failure or if the anhepatic phase is prolonged, it is appropriate to monitor and treat plasma glucose. If large volumes of blood products are transfused during the anhepatic phase, the reduced capacity of the body to metabolize citrate in the absence of the liver can lead to citrate-associated hypocalcaemia. Particular care should therefore be paid to plasma ionized calcium levels during the anhepatic phase to avoid the risk of reduced vascular tone and compromised myocardial contractility [47].
9.2.5 Intraoperative Management: Neohepatic Stage
This phase of the procedure commences with the reperfusion of the liver graft (usually after completion of the vena cava and portal vein anastomoses) and ends with the closure of the skin and transfer of the patient to the recovery area or ICU. During this phase of surgery, the liver graft is reperfused with the recipient’s blood via the portal vein, the hepatic artery and the hepatic veins. During this phase, the biliary anastomosis is made. If the recipient’s biliary system has unfavourable anatomy or is diseased, the graft biliary system may require anastomosis to the recipient small bowel, significantly prolonging the operating time. During the reperfusion phase, it is vital that the anaesthesiologist responds actively to the physiological changes this phase entails, in order to optimize the conditions for the survival of the graft. Maintenance of appropriate perfusion pressures to the graft and avoidance of high central venous pressures which may contribute to venous congestion are key factors to this purpose. The surgeon is often able to advise the anaesthesiologist about the venous congestion of the graft and its colour and may request lowering the central venous pressure to optimize this situation. In addition, the reperfusion of the graft, which has been previously kept in ice for preservation, represents an important thermal load for the recipient to absorb. It is expected that the recipient’s core temperature (measured, e.g., by a temperature probe on the pulmonary artery catheter) will drop approximately 0.5–1 °C in the minutes following reperfusion. A relatively rapid increase in the core temperature of the recipient following this initial dip can be regarded as an important sign of exothermic cellular metabolism and function of the graft. Additionally, improvement in acid-base status and stable glucose levels are reassuring signs of graft function. Postreperfusion syndrome is the most significant anaesthetic concern during the reperfusion phase. This syndrome consists of severe cardiovascular dysfunction with decreased cardiac output, severe systemic hypotension, bradyarrhythmia, asystole, raised pulmonary artery pressure, and raised pulmonary capillary wedge pressure and central venous pressure [108]. Reperfusion syndrome is usually observed in the first minutes following the reperfusion of the liver graft and, if not managed actively, can cause cardiac arrest. The formal definition of the syndrome has been refined to include a mean arterial pressure drop of at least 30 % for a period of at least 1 min, within 5 min of reperfusion. Postreperfusion syndrome has proven difficult to predict, with only increased age of the liver graft donor being a strong predictive factor. Its occurrence has been associated with poor outcomes in terms of survival and postoperative renal function [109]. It is not clear if these associations are causative. Various methods are used counteract the negative haemodynamic effects of this syndrome with variable effects. These include flushing the graft with cold saline or autologous blood, sequential or partial unclamping of hepatic graft outflow and anticipatory use of various antihistamine agents, vasopressors, calcium, bicarbonate and methylene blue in cases resistant to other treatments [110]. Haemodynamic perturbations are treated with vasopressor agents, including cautious bolus doses of phenylephrine and epinephrine [111]. Calcium chloride and sodium bicarbonate are also often administered, guided by arterial blood gas results. Life-threatening hyperkalaemia requires prompt treatment; calcium chloride and sodium bicarbonate are the drugs of choice. Dialysis should be considered early in the procedure for oliguric patients with elevated potassium levels [109].
9.2.6 Management of Intraoperative Coagulopathy
Patients with active sites of bleeding should be transfused with fresh-frozen plasma (FFP), packed red blood cells, prothrombin complex concentrates, antifibrinolytic agents, recombinant factor VIIa (rFVIIa) or platelets.
Prothrombin complex concentrates (PCCs) contain the vitamin K-dependent clotting factors II, VII, IX and X and the coagulation inhibitors protein C and S, which allow the correction of coagulation alterations using small fluid volumes. Current evidence suggests that even in high-risk patients, PCCs are safe and that thromboembolic events are rare [82, 83].
FFP is often transfused in order to correct a deranged INR; however, the exponential relationship between coagulation factors and the measured value of PT/INR is not always appreciated. It has been shown that FFP is unable to contribute a sufficient amount of coagulation factors to correct PT/INR by 50 % in most cases, even for mildly prolonged PT/INR [84, 85].
Antifibrinolytic therapy reduces blood loss and transfusion requirements [86]; these drugs are recommended for the treatment of fibrinolysis evidenced by microvascular oozing or TEG/ROTEM clot lysis measurement (CLI < 15). Meta-analyses have shown that both tranexamic acid and aprotinin can reduce RBC transfusion requirements during OLT [87]. Various dosing regimens have been suggested, and it is unknown which is the lowest effective dose. Currently, tranexamic acid is usually given in 1–2 g increments. In the early years of OLT, the routine use of prophylactic antifibrinolytic agents was common, since the mortality associated with massive blood loss was high, and the risk associated with antifibrinolytic drugs was small in comparison. Now that massive haemorrhage is less frequent, antifibrinolytics are no longer recommended for routine prophylaxis [88]. The response to antifibrinolytic agents should be monitored using TEG/ROTEM to guide the administration of further doses.
Hypofibrinogenaemia has also been shown to influence blood product requirements. A baseline MA (maximum amplitude of the clot measured with TEG) of <35 mm at the beginning of the transplant and measured fibrin degradation products >48 mg/L has been demonstrated to lead to hyperfibrinolysis in 100 % of patients [89]. When haemodilution and massive bleeding occur, fibrinogen is the first factor to reach critical levels [90]. A concentration of <1.5–2 g/L increases haemorrhagic tendency, so this value or signs of functional fibrinogen deficit on TEG or ROTEM should trigger immediate fibrinogen repletion [74].
Recombinant factor VII improves haemostasis by directly activating factor X, precipitating the conversion of prothrombin to thrombin to form a haemostatic clot. Factor VII binds to the surface of activated platelets at sites of vascular injury, increasing localized thrombin generation. Several meta-analysis and systematic reviews of the use of factor VII in hepatic surgery (including transplantation) failed to show a reduction in transfusion requirements, but they showed a significant increase in the incidence of arterial thrombotic events [91, 92]. The ESA guidelines for massive bleeding in visceral and transplant surgery echo this with recommendation against the prophylactic use of rFVIIa, reserving its use only as rescue therapy for uncontrolled bleeding.
Intraoperative platelet transfusions have been identified as a strong independent risk factor for survival after OLT, with a greater hazard ratio than RBCs transfused, particularly for TRALI [112]. A low platelet count combined with a low fibrinogen always leads to a reduced MA/MCF and is strongly associated with an increased tendency towards bleeding [93].
A marked heparin-like effect on the TEG at the time of reperfusion is common and is due to both exogenous heparins administered to the organ donor and the release of endogenous heparinoids from the vascular endothelium and activated macrophages, triggered by the ischaemia-reperfusion injury. This does not appear to contribute significantly to the risk of bleeding and is usually a temporary phenomenon, unless graft function is poor [113]. Reversal with protamine is rarely indicated. Native TEG is extremely sensitive to heparin, and endogenous heparin can be detected in some patients even prior to reperfusion [114, 115].
Table 9.2 synthesizes the main intraoperative tips for anaesthesiologic management for every phase of the intervention.
Table 9.2
Main intraoperative tips for anaesthesiologic management for every phase of the intervention
Anaesthetic induction and maintenance | Preanhepatic stage | Anhepatic stage | Neohepatic stage |
---|---|---|---|
Routine monitoring electrocardiography pulse oximetry, capnography, temperature, invasive arterial blood pressure, baseline arterial blood gas and thromboelastogram 8.0 F or larger cannulas into peripheral vein | Maintain Hgb >10 g/dl Lower CVP (5 cmH2O), restriction of fluid administration Infusion of albumin 20 % if severe hypoalbuminaemia or drainage of ascites Epinephrine or dopamine to preserve cardiac output CO >5 L/min | Maintain Hgb >8 g/dl IV fluids to keep CVP around 5 cmH2O If venovenous bypass maintain flow rate of 1.5/2 L/min | Maintain Hgb >8 g/dl Euvolaemia (CVP 5–10 mmHg) IV adrenaline 10/20 mcg bolus to keep MAP >60 mmHg |
Induction of anaesthesia in a rapid sequence, if risk of aspiration Sellick’s manoeuvre Fentanyl 1–2 μg/kg, propofol 0.5–2 mg/kg, cisatracurium 0.5–1.0 mg/kg Maintain anaesthesia balanced with minimal flow, low tidal volume (6–8 ml/Kg), positive end expiratory pressure 5–8 cm H2O if necessary recruitment manoeuvres Venous access 8.0 French bilumen into left internal jugular vein ultrasound-guided or right if use venovenous bypass | Maintain coagulation status by thromboelastography if prolonged R time Prothrombin complex concentrates (if necessary restriction of FFP administration) or FFP If decreased alpha angle reduced MA platelets infusion or fibrinogen concentrates (maintain fibrinogen >2 g/L) | Maintain coagulation status by thromboelastography if increased lysis (CLI <15) Antifibrinolytic therapy | Maintain coagulation status by thromboelastography delayed graft function and heparin-like effect Maintain MA >45 mm with platelet infusion |
Invasive monitoring 8.0 French pulmonary catheter into right internal jugular vein transoesophageal echocardiography if portopulmonary hypertension or bypass | Norepinephrine to keep mean blood pressure >60 mmHg | Adrenaline/norepinephrine to preserve blood pressure >60 mmHg and CO >5 L/min | Adrenaline/norepinephrine to preserve blood pressure >60 mmHg and CO >5 L/min |
Intravenous antibiotics | Transoesophageal echocardiography if necessary recommend in postreperfusion graft congestion | ||
Warmers | Correct metabolic acidosis, hypocalcaemia, hypomagnesaemia and glycaemia | Correct metabolic acidosis, hypocalcaemia, hypomagnesaemia and glycaemia | Correct metabolic acidosis, hypocalcaemia, hypomagnesaemia, glycaemia and hyperkalaemia |
Connect to rapid infusion system (flow rates 500–1,500 mL/min) | After skin closure echo Doppler for intrahepatic flow and transfer to intensive care | ||
Incision |
9.3 Postoperative Management of Liver Transplantation
Intensive care management of liver-transplanted patients mainly focuses on rapid haemodynamic stabilization, early weaning from mechanical ventilation, proper fluid administration, kidney function preservation, identification and prompt treatment of poor graft function and appropriate monitoring and correction of coagulopathy.
9.3.1 Postoperative Ventilatory Support and Weaning from Mechanical Ventilation
In some patients respiratory weaning is feasible immediately at the end of the surgical procedure. Other patients are stabilized in the ICU before discontinuing mechanical ventilation, in order to ensure that liver function is satisfactory. A number of studies suggest that early or very early tracheal extubation (immediately in the operating room or within 3 h postoperatively) has been associated with a persistent maintenance of satisfactory gas exchange. The incidence of reintubation was not increased when compared to patients extubated later [116, 117]. Mandell et al. [118] demonstrated that a protocol for early extubation and rapid transfer of liver recipients from the ICU to the surgical ward did not negatively impact on long-term outcome. In the early extubation protocol, 1- and 3-year graft and patient survival were above the national average at the time. More recently, Biancofiore et al. [117] retrospectively studied 168 patients who underwent orthotopic liver transplantation and identified a number of risk factors for delayed extubation (Table 9.3). The best predictor of successful extubation according to their results was a model for end-stage liver disease (MELD) score less than 11. Finally, it is clear that an optimal patient selection strategy is key for successful early extubation, though definite criteria and timing are not yet well defined by the current literature. Furthermore, it is not possible to generalize results from different centres who recommend this strategy, due to differences in the preoperative clinical conditions of the patient populations, the surgical skills and the postoperative resources available.
Table 9.3
Risk factors for delayed extubation in LT patients
Severity of liver disease before surgery (Child-Pugh and MELD score) |
UNOS status |
Age |
Duration of graft ischaemia |
Duration of surgery |
Primary graft dysfunction |
Intraoperative blood requirements |
Body temperature on ICU admission |
Renal dysfunction |
Hepatic encephalopathy |
Need to inotropes or vasopressor |
Inadequate oxygenation |
Mechanical ventilation has a number of potentially detrimental side effects in the liver transplant patient. It can worsen venous congestion of the liver graft by increasing the intrathoracic pressure and reducing venous return from the IVC and hepatic veins [119]; moreover, prolonged mechanical ventilation increases the risk of ventilator-associated pneumonia [120]. Failure of an early extubation strategy may be associated with impaired oxygen delivery to the newly grafted liver.
A difficult weaning from mechanical ventilation is very often a consequence of postoperative respiratory complications. These can be attributed to massive transfusions, pleural effusion, inadequate clearance of bronchial secretions, pneumonia and adverse effects of the immunosuppressive therapy. Acute respiratory distress syndrome (ARDS) is one of the major complications following OLT. Its main causes include a severe reperfusion syndrome, substantial blood loss, prolonged surgical times and early postoperative infections, mainly caused by translocation of gram negative bacteria form the intestinal mucosa. The pathophysiological mechanism of transfusion-related acute lung injury (TRALI), a causative factor of ARDS, seems to be related to donor alloantibodies that react against granulocytes or leucocyte antigens (anti-HLA) [121]. The management and treatment of respiratory complications, including ARDS, are primarily supportive, with obligatory mechanical assistance in cases of ventilatory failure. It is known that ventilation at high intrathoracic pressure may cause venous congestion of the graft increasing the risk of ischaemic damage. The available literature regarding the ventilatory strategy in transplanted liver patients with ARDS provides little evidence, but we have to underline that the preservation of the graft function is mandatory. This can be achieved by privileging the maintenance of a very good oxygenation, even if elevated intrathoracic pressures have to be applied. Sometimes liver transplant patients prove difficult to wean form mechanical ventilation, due to unsatisfactory gas exchange during various T-piece trials. In these circumstances a rapid extubation followed by an immediate application of a noninvasive ventilator support should be considered. Noninvasive ventilation (NIV) by adding a pressure support (PS) with a continuous positive end expiratory pressure (PEEP) could prevent the loss of vital capacity and impede severe lung derecruitment following extubation. As opposed to the first applications in solid organ transplantation, when NIV was mainly delivered by full facial mask [122], nowadays it is predominantly delivered by the helmet system. Daily experience shows that the helmet is more suitable for longer application of NIV. The first application of NIV in solid organ transplant recipients was described by Antonelli et al. [123]. If postoperative respiratory failure is severe enough to require a prolonging of mechanical ventilation, ventilator strategies that minimize insults to both the lung and the allograft should be used. Airway pressures and PEEP should be set in order to improve oxygenation without simultaneously impairing liver outflow. In liver recipients affected by severe ARDS, low tidal volume (6 mLKg−1 of ideal body weight), relatively high respiratory rates and PEEP confer a survival advantage by keeping the lung open and avoiding atelectasis and shear stresses on lung units [124]. Mechanical ventilation with high PEEP, as previously stated, has been reported to impair liver outflow. Besides determining an increase retrograde blood accumulation and liver oedema, an excessive PEEP (>10 cmH2O) may also depress the splanchnic perfusion and hepatic performance, by increasing venous stasis in the portocaval system and depressing cardiac output. When critical hypoxaemia occurs in the setting of a severe respiratory failure, inhaled nitric oxide may be administered.
9.3.2 Postoperative Haemodynamic Monitoring and Circulatory Stabilization
Because of potential cardiocirculatory instability and the need to optimize cardiac output and organ perfusion, haemodynamic monitoring must be strict in the immediate postoperative period. Maintenance of postoperative graft function depends primarily on liver cell recovery, which can be enhanced by optimizing the liver haemodynamics and preventing venous stasis. Knowledge of the preload and afterload indexes of both right (RV) and left ventricle (LV), mean and transpulmonary pressure and pulmonary vascular resistance (PVR) is useful in managing pharmacologic interventions, volume therapy and vasoactive drug administration. It is useful to insert also a pulmonary artery catheter (PAC) equipped with a fast response thermistor capable of assessing RV ejection fraction (RVEF%) and ventricular filling through RV end-diastolic volume calculation (RVEDV).
Subclinical hypovolaemia or excessive cardiac filling resulting in pulmonary oedema and deterioration of gas exchange may lead to inadequate graft perfusion and increase postoperative morbidity. Patients with cirrhosis tend to have impaired ventricular contractility in response to physiologic stress or pharmacologic stimulation. Additionally, metabolic disturbances, in the form of acidosis, hypothermia and electrolyte disturbances, can further reduce the cardiac performance and lead to circulatory instability. Haemodynamic depression may also be a long-term result of the reperfusion syndrome and/or a consequence of graft nonfunction. Other causes of postoperative hypotension are a pre-existing dilated cardiomyopathy, the potential for coronary artery disease and unrecognized hypovolaemia from various factors, including third space losses, haemorrhage and ongoing ascites formation. The possibility of perioperative myocardial infarction causing left ventricular dysfunction must be kept in mind in cases of refractory circulatory dysfunction. Postoperative ‘subclinical’ pulmonary oedema is not infrequent, with at least 50 % of these episodes developing within the first 24 h. The rapid improvement of systemic vasodilatation with the return of liver graft function, which can result in a sudden increase in the afterload, is another potential cause of excessive strain on the heart. It is known that the evolution of some cardiocirculatory parameter and ossiforetics (progressive increasing in arteriovenous oxygen content) plays an important role as prognostic indexes.
Haemodynamic optimization following orthotopic liver transplant aims at preventing inadequate cardiac filling, which results in suboptimal tissue perfusion and possible organ failure. Continuous monitoring of dynamic parameters of fluid responsiveness and/or assessment of RV (right ventricular) end-diastolic filling and RV ejection fraction % is helpful in maintaining an adequate central blood volume. Optimizing cardiac output will avoid excessive fluid administration, thus preventing both pulmonary congestion and an unrecognized increase in the sinusoidal and hepatic vein pressures. In liver-transplanted patients, a vasodilated and hyperdynamic state may take days or weeks to regress to near-normal levels. Moderate filling followed by vasoconstriction should effectively treat this evolving clinical condition. Infusion of norepinephrine is usually started in the operating room to ameliorate the hyperkinetic status of the patients and continued in ICU to achieve a good mean arterial pressure and thus a good perfusion of the new liver.
A correct fluid and electrolyte balance in the immediate recovery period in the ICU is also mandatory. Many studies demonstrate that one of the significant predictors of readmission to the ICU of the transplanted patients was the amount of blood product administered intraoperatively [125]. Generous fluid replacement may result in volume overload, water-sodium retention and capillary leak syndrome in the third space and may further worsen graft congestion and oedema caused by ischaemia-reperfusion syndrome. Once the postoperative haemodynamics have been stabilized, it is necessary to promote the return of the sequestered fluid from the peripheral circulation and third space, back to the central circulation. An appropriate negative fluid balance in the first days after operation apparently decreases the incidence of early pulmonary complications and may be associated with improved oxygen delivery to the graft. Lowering right ventricular volume and pressure would create a venous pressure gradient between the portal and the central venous circulation that draws blood through the donor graft. A rationale approach to maintaining circulating volume is by providing two-thirds of required fluids with crystalloids and replacing half of drain loss with 5 % albumin solution. The real advantage of albumin solutions on the final outcome is still under debate as the evidence for a specific benefit as been substantial only in the settings of decompensated cirrhosis. A few reports have addressed the use of albumin after orthotopic liver transplant [126]. Although the postoperative transfusion policies may differ among centres, the replacement of blood components to achieve haemoglobin between 8 and 10 g L−1, as commonly adopted during transplant surgery, could be a valid approach [127]. Maintaining a postoperative haematocrit between 25 and 30 % would be helpful to guarantee an adequate oxygen delivery to the new graft. Disturbances of the metabolic function of different organs and alterations of the hormonal balance are very common and mainly caused by ischaemia-reperfusion injury, surgical stress and pre-existing pathologies and the various drugs administered to the patients. Common disturbances include alteration of metabolism of glucose, of lactate levels and of electrolytes. Hyperglycaemia is the most frequent alteration, and it is due to the preoperative stress, liberation of endogenous catecolamines, administration of high-dose steroids to induce immunosuppression, chronic immunosuppressive therapy (calcineurin inhibitors) and glucose given for enteral or parenteral nutrition. A tight control of blood glucose levels is mandatory and best achieved by continuous infusion of insulin. Hyperglycaemia is very common and usually lasts until a good peripheral utilization of glucose and normalization of the endogenous hormonal secretion returns. This is greatly ameliorated by an early enteral feeding. The persistence of hypoglycaemia on the other hand can be a picket sign of a compromised liver recovery.
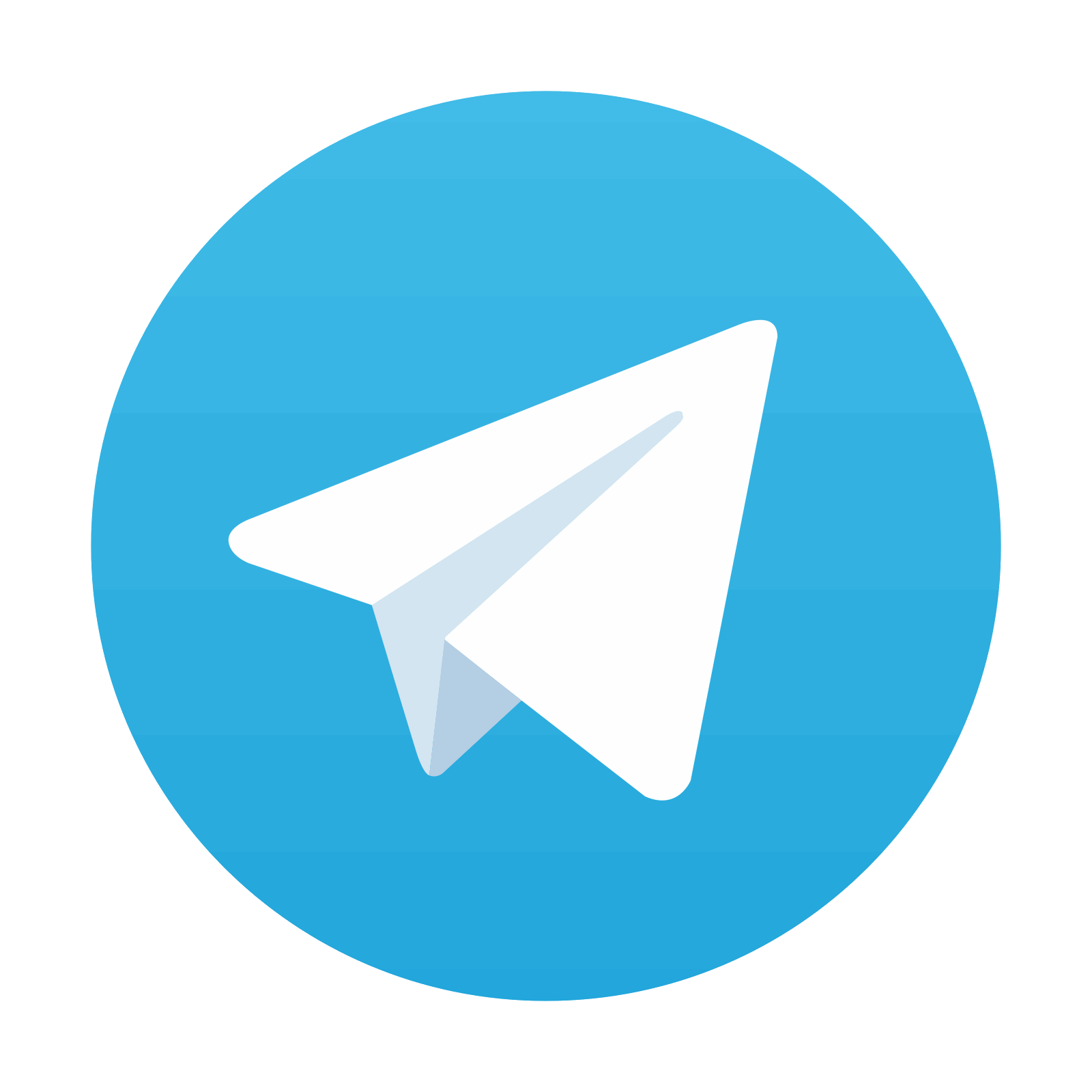
Stay updated, free articles. Join our Telegram channel

Full access? Get Clinical Tree
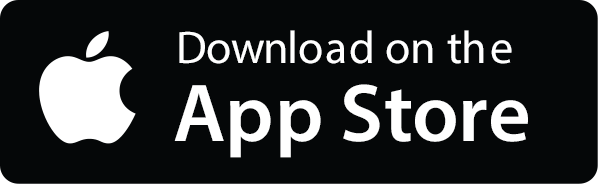
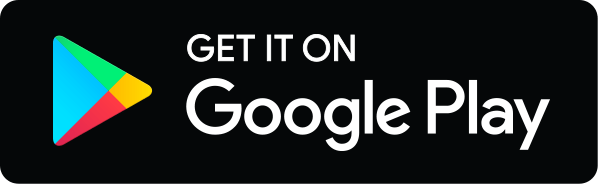