David J. De La Zerda and Frank L. Powell
INTRODUCTION
The most common medical emergency elicited by ascending to high altitude is hypobaric hypoxia from a low partial pressure of inspired oxygen (PIO2). The decrease in PIO2 at high altitude is predicted by the formula: PIO2 = FIO2 × (Pbaro – PH2O). FIO2 is the fraction of inspired oxygen and remains constant at 21% regardless of the altitude. Pbaro is the atmospheric pressure (e.g., 760 mmHg at sea level). PH2O is the partial pressure of water vapor when the air is fully humidified, as it is in the alveoli. Pbaro is the major factor that determines PIO2 because at body temperature (37°C), PH2O is 47 mmHg and FIO2 and PH2O are constant. Table 20-1 shows the PIO2 at various altitudes. The drop in Pbaro at high altitudes causes hypobaric hypoxia. In patients with preexisting hypoxemia from lung disease, either air travel or activities at high altitude (e.g., skiing and mountaineering) can increase the risk of developing severe hypoxemia.
AIR TRAVEL
Although most commercial flights cruise between 22,000 and 44,000 ft (6,700–13,400 m), the Federal Aviation Administration mandates that cabins should be pressurized to simulate an altitude below 8,000 ft (2,438 m). At that altitude, the partial pressure of oxygen in arterial blood (Pao2) in normal individuals is typically between 75 and 53 mmHg, which corresponds to a decrease in oxygen saturation of 3% to 5% from sea level.
According to the Centers for Disease Control and Prevention (CDC), in-flight medical emergencies are rare. One in 10,000 to 40,000 air travelers have a medical incident, 1 in 150,000 require the use of medical equipment or drugs, and 0.3 of 1,000,000 die (and two-thirds of these deaths are due to cardiac disease). The most common medical problems are vasovagal, gastrointestinal, respiratory, cardiac, and neurologic incidents. Prescreening patients appears to be valuable in assuring healthy flying. In a study of 1,115 patients referred for screening, 1,011 were cleared for travel and none had significant in-flight problems. The remaining 104 patients had a variety of unstable and stable conditions that precluded air travel. Preflight evaluations and management requires some understanding of the risks involved for a variety of specific disease states. Even with preflight screening, a few problems can be anticipated.
Barometric Pressure, Altitude and Oxygen |
Most, but not all, patients with chronic obstructive pulmonary disease (COPD) can fly safely with little or no intervention. There is considerable individual variation, since even the moderate altitudes reached in commercial aircraft can lead to changes in ventilatory function. After 60 minutes at 8,000 ft (2,438 m, Pb = 565 mmHg), pulmonary function changes reported in both healthy persons and patients with severe COPD include a fall in forced vital capacity (FVC), increase in residual volume, and variable changes in airway resistance. In a small cohort of 44 COPD patients with FEV1 of 34% (±9%) of predicted who flew for a median time of 3 hours over a 28-day period, 18.2% reported symptoms and signs during their flight. Complaints were primarily dyspnea, edema, wheezing, and cyanosis. Only two patients requested supplemental oxygen, and none required hospital admission. Other studies have shown similar results.
Patients with pulmonary hypertension have an increased risk of hypoxia during air travel because lower inspired oxygen tension induces pulmonary artery vasoconstriction. The subsequent elevation in pulmonary vascular resistance may increase the stress on the already compromised right ventricle. For this reason, patients with functional class III or IV should probably avoid unnecessary flights. Ideally, patients in functional class I and II should receive a formal evaluation with a hypoxia-altitude simulation test before air travel. If, after a thorough evaluation, a patient is deemed fit to fly, the Aerospace Medical Association recommends that exercise be limited during the flight.
Asthma is a common pulmonary condition that poses a risk of exacerbation during air travel. Most flight-associated exacerbations can be managed with inhaled bronchodilators alone. While it is most convenient for the patients themselves to bring their own bronchodilators on board, emergency-use bronchodilators are part of the standard in-flight emergency medical kits on commercial planes. The Aerospace Medical Association recommends that all asthmatic patients bring a course of oral prednisone on board for use in an emergency during the flight.
Pneumothorax poses a special risk during flight. Before air travel, the etiology of a pneumothorax must be established and corrected. A recent pneumothorax may expand during air travel and progress to become a tension pneumothorax. The presence of a pneumothorax, therefore, is an absolute contraindication for air travel. It may be reasonable to travel 3 weeks after successful drainage of a pneumothorax, provided complete resolution is confirmed radiographically prior the flight.
Extrapulmonary air in the chest cavity (e.g., following thoracic surgery) also may pose significant risk and should delay air travel. If the cabin pressure was to abruptly decrease, the entrapped air may rapidly expand and increase the risk of life-threatening tension pneumothorax.
Commercial air travel is associated with an increased risk for the development of deep vein thrombosis (DVT). Extrinsic risk factors include immobility, dehydration, increased venous pressure from cramped seating, endothelial damage from seat edges, and hemoconcentration from fluid shifts to the interstitial space from decreased cabin pressure. Intrinsic risk factors that contribute to the development of DVT from air travel include history of DVT or pulmonary embolism (PE), postthrombotic syndromes, malignancy, pregnancy, and chronic venous insufficiency. In high-risk patients, the American College of Chest Physicians (ACCP) recommends frequent ambulation (when permitted by the aircrew), isometric calf muscle exercise, aisle seating, and graduated compression stockings (below-knee, 15–30 mmHg of pressure at the ankle). ACCP recommends against the use of aspirin or anticoagulants to prevent DVT during air travel, but anticoagulant treatment for other purposes should not be discontinued.
The primary cardiac response to moderate hypobaric hypoxia that occurs during flight is an increase in heart rate with a subsequent reduction in stroke volume. These processes may increase the risk of coronary events in patients with previous histories of coronary artery disease. Stable cardiac disorders, however, do not preclude flight. For example, stable angina requires little, if any, change in management, but air travel within 2 weeks of myocardial infarction would be advisable only if there is no angina or dyspnea at rest, the passenger is not alone or rushed, and nitroglycerin is readily available. Complicated myocardial infarction or limited ambulatory ability requires further cardiology evaluation and perhaps stress testing before air travel.
The Aerospace Medical Association considers the following cardiovascular conditions as contraindications for commercial flights: uncomplicated myocardial infarction within 2 to 3 weeks, complicated myocardial infarction within 6 weeks, unstable angina, decompensated or severe congestive heart failure, uncontrolled hypertension, coronary artery bypass grafts (CABG) within 10 to 14 days, cerebrovascular accident (CVA) within 2 weeks, uncontrolled ventricular or supraventricular tachycardia, Eisenmenger syndrome, and severe symptomatic valvular heart disease. Further recommendations regarding these conditions are beyond the scope of this review, but cardiology evaluation is strongly recommended before air travel.
Supplemental oxygen may circumvent most barriers to flight among patients with limited cardiopulmonary function. The sea level Pao2 is a useful screen to predict which patients might require supplemental oxygen in flight. Oxygen should be considered for patients with a sea level Pao2 of less than 70 mmHg. For those with a Pao2 more than 70 mmHg, a number of preflight evaluations have been used to predict oxygen requirements during flight. For those who already require supplemental oxygen, some authors suggest simply adding 2 L/min of flow to baseline requirements. Others use more complicated prediction equations. Such equations should be used with caution, because they are derived from specific populations. More subjective issues such as cardiopulmonary reserve or muscle weakness are not included in the equations. Prediction equations also tend to overestimate Pao2 values for healthy controls. Below is one such equation that resulted in a high predictive value (r2 = .99, P < .001) at moderate altitude:
where Pao2 alt = Pao2 at expected altitude achieved, Pao2 grnd = Pao2 at baseline elevation, max alt = maximum altitude achieved in meters, and grnd alt = baseline elevation in meters.
In 1984, Gong et al. described the use of the hypoxia-altitude simulation test (HAST) or hypoxia inhalation test, to objectively evaluate at sea level the oxygen demands in patients with pulmonary diseases. Their initial report included 22 patients with COPD who were asked to breathe a low FIO2 (high nitrogen) mixture of gases from a tight-fitting mask for 15 minutes. Three different FIO2 are typically used: 0.209 (baseline), 0.17 (simulating 5,000 ft), and 0.15 (simulating 8,000 ft). These conditions are comparable to the results achieved in hypobaric chambers, but are much simpler to perform.
HAST is a relatively simple test. Initially a baseline arterial blood gas is obtained to measure Pao2 and Paco2. The inhaled gas mixture is then changed to an FIO2 of 0.15. The patient’s vital signs, oximetry, and symptoms (e.g., dyspnea or chest pain) are closely monitored. If worrisome symptoms or physical signs occur, or if pulse oximetry readings fall below 85%, then arterial blood gases are repeated and the test is stopped. If the saturation remains greater than or equal to 88% for 20 minutes, then the test is completed and arterial blood gases are repeated. If Pao2 at the end of the test is greater than 55 mmHg, then supplemental oxygen typically is not required for air travel. If it is less than 50 mmHg, then supplemental oxygen would be warranted. A borderline test result (Pao2 between 50 and 55 mmHg) may be supplemented by an additional arterial blood gas sample taken while the patient is walking and breathing an FIO2 of 0.15.
In summary, several medical problems may occur during air travel. At-risk patients should be carefully evaluated prior to travel and appropriate recommendations should be made based upon their underlying conditions. Hypoxia associated with reduced cabin barometric pressure can be prevented by supplemental oxygen. Patients with preexisting needs for supplemental oxygen may increase their oxygen flow by 2 L/min above their baseline requirements during air travel. For those with milder hypoxemia, HAST or several prediction equations may help guide the decision to supply supplemental oxygen for air travel. Arrangements should be made by the patient with the airline way in advance of the travel date to determine method of administration, stopping points at elevated altitudes, and length of travel time during which supplemental oxygen may be needed. In more challenging situations, discussion with the airline’s flight surgeon may be needed to complete the arrangements.
HIGH-ALTITUDE ILLNESS
Altitudes exceeding those typically simulated by pressurized cabins can produce a unique set of disorders that range from benign to fatal. Fortunately, the more serious problems arise with considerable less frequency. However, increasingly publicized accidents in remote locales reflect a surge of interest in high-altitude adventure and a corresponding risk of high-altitude illness. Most high-altitude illnesses occur among the 12 million skiers traveling to mountainous areas each year. However, increasing numbers of people are adventuring into extreme altitudes where more serious problems can occur.
Altitudes can be defined physiologically as “high altitude” (5,000–11,500 ft, or 1,500–3,500 m) above sea level, “very high altitude” (11,500–18,000 ft, or 3,500–5,500 m) and “extreme altitude” (>18,000 ft or 5,500 m). Altitudes above 20,000 ft have been called the “death zone” as permanent life is not sustainable at such heights. Ascent to high altitudes can produce a continuum of altitude-related illnesses. Acute mountain sickness (AMS) is most common at high altitudes (5,000–11,500 ft), while high-altitude pulmonary edema (HAPE) and high-altitude cerebral edema (HACE) are more serious high-altitude illnesses that may result in death but occur only rarely at very high and extreme altitudes. A number of other altitude disorders can arise, but exceed the scope of this review. These include snow blindness, retinal hemorrhages, hypothermia, and high-altitude flatus expulsion (a serious concern for climbers trapped in the closed confines of a tent during inclement weather), and problems associated with chronic exposure.
Ski resorts constitute the bulk of recreational destinations at high altitude. Many resorts are located at or above 10,000 ft (3,000 m), and they can be accessed very rapidly by motorized transport. There are also commercial and research activities at high altitudes, such as copper mines at 19,520 ft (5,950 m) in Chile and astronomy at 13,779 ft (4,200 m) at the Mauna Kea Observatory in Hawaii. Some 6,000 people climb Mt. Rainier at 14,408 ft (4,392 m) per year, and 800 climbers summit Denali, the highest peak in North America at 20,320 ft (6,193 m). Nearly 50% of all travelers to these altitudes experience some symptoms. Altitude illness is reported by 66% of climbers on Mt. Rainier, 47% on Mt. Everest, and 30% on Denali.
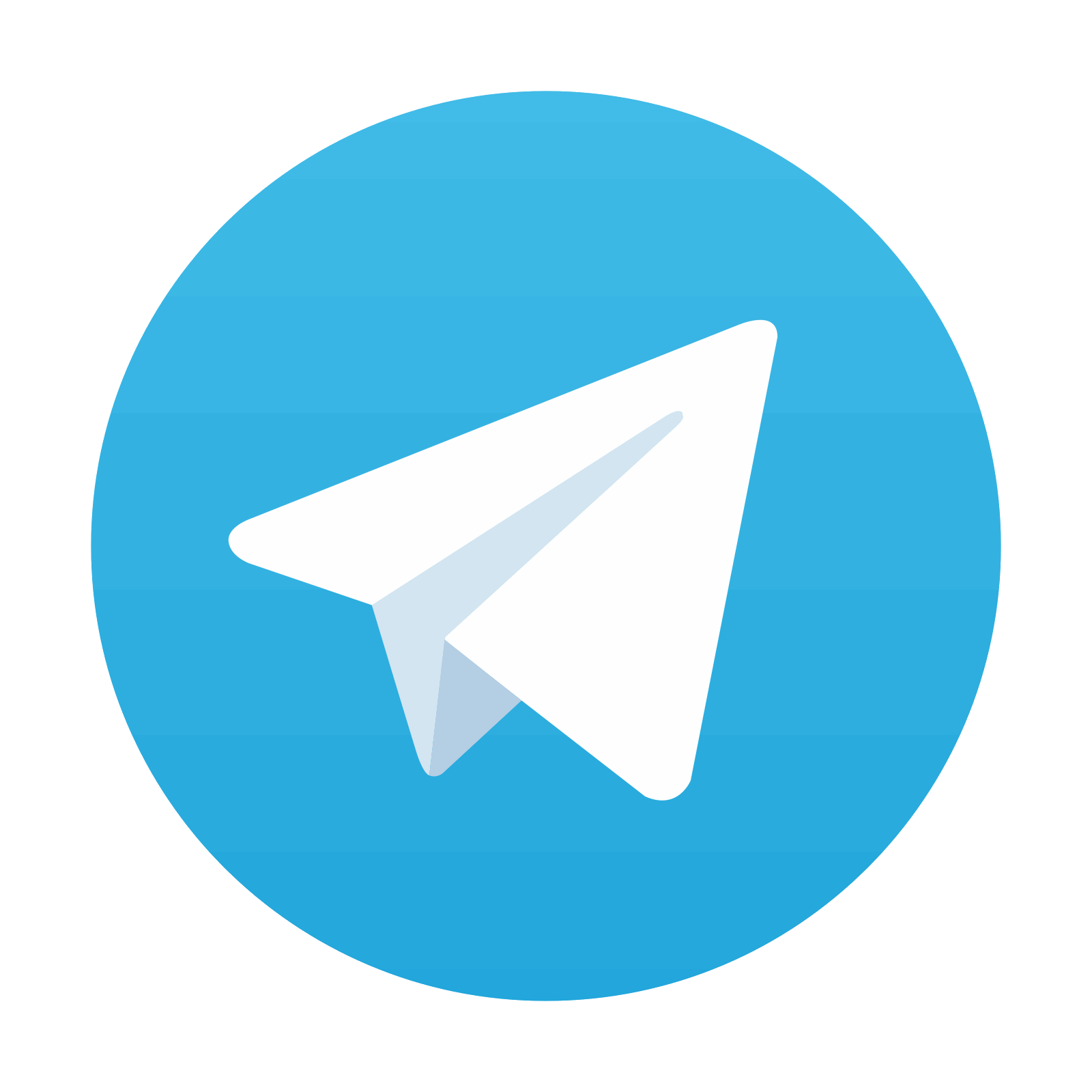
Stay updated, free articles. Join our Telegram channel

Full access? Get Clinical Tree
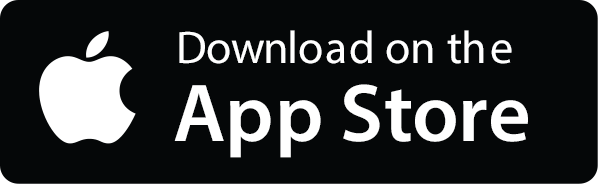
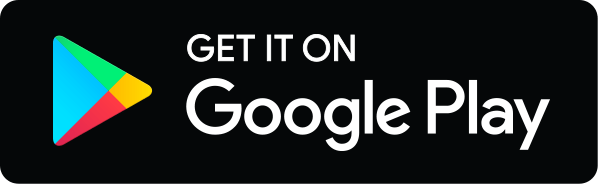