6.2 Effects of Glucocorticoids on Adipose Tissue
Glucocorticoids have a number of very important functions. Their involvement to contrast stressor agents or situations is known for many years, although not completely elucidated.
Recent studies showed that obesity cannot be explained just as a mechanism of cause–effect, but many factors and conditions can contribute to its pathophysiology. In this regard, physiological and pathophysiological stresses have a significant long-term impact. In fact, in a stressful situation, GCs are secreted through the CRH pathway, and these elevated levels of CGs seem to stimulate appetite, increasing feeding and consequently producing obesity [7]. GCs play an important role in lipid uptake. It has been demonstrated that GC receptor is expressed in adipose tissue and its density in visceral adipose tissue is higher than in other adipose deposits [8]. Visceral adipose tissue has been associated to cardiovascular disease [9]. Veilleux et al. showed an increased accumulation of visceral adipose tissue in humans with a higher 11-β-HSD1 activity, increased lipolysis, and increased levels of lipoprotein lipase (LPL) activity [10]. Moreover, Morton et al. demonstrated that 11-β-HSD1-deficient mice were resistant to diet-induced visceral obesity [11]. Taken together, all these data can suggest that there is an irrefutable correlation between GCs and visceral adipose tissue; anyway, the underlying mechanisms are not completely elucidated.
The action of GCs on fatty acid metabolism is very complicated and can be explicated through the beta-adrenergic pathway, where cAMP is usually the first target of investigation. The action of GCs can be shared in the short term and long term. At short term, GC infusion enhances the release of non-esterified fatty acids from the adipocytes through the activation of a particular type of LPL, hormone-sensitive lipase [12]. Long-term effects, instead, were mediated by the regulation of the transcription of specific gene target including HSL, ATGL, and perilipin (cf. Table 6.1).
Table 6.1
Summary of previous studies investigating the effects of GCs on lipolysis
Article | Adipose model | Dose/type of GC | Duration | Lipolysis | Suggested mechanisms |
---|---|---|---|---|---|
Fain et al. | Isolated parametrial adipocytes; high-fat–fed rats | Dexamethasone 0.016 μg/mL (0.04 μmol/L) | 4 h | ↑ FFA release | Altered transcription |
– | – | – | – | ↔ glycerol release | – |
Fain et al. | Isolated parametrial adipocytes; rat | Dexamethasone 0.1 μg/mL (2.5 μmol/L) | 4 h | ↔ glycerol | ↔ cAMP |
Lamberts et al. | Isolated epididymal adipocytes; rat | Dexamethasone 0.1 μg/mL (2.5 μmol/L) | 4 h | ↔ glycerol | ↔ cAMP |
Samra et al. | In vivo human | Hydrocortisone 1.5 μmol/L | Acute IV infusion | ↑ overall NEFA release | ↑ LPL activity |
– | – | – | – | ↓ NEFA venoarterial difference in abdominal | ↑ peripheral lipase activity |
– | – | – | – | – | ↓ visceral lipase activity |
Ottosson et al. | Isolated subcutaneous human | Hydrocortisone 1 μmol/L | 3 days | ↓ basal lipolysis | ↓ Production or |
– | – | – | – | – | ↑ elimination of cAMP |
– | – | – | – | – | ↓ β-adrenergic–stimulated lipolysis |
Campbell et al. | 3T3-L1 adipocytes | Corticosterone 1–100 μmol/L | 48 h | ↑ glycerol release | ↑ HSL and ATGL transcription |
– | – | – | 48 h | ↓ glycerol release | ↓ cAMP activity |
Bjorntorp has demonstrated that GCs determine the activation of LPL with consequent increase of visceral adipose deposit [3].
However, in literature there are many different evidences about the pathway of GCs on lipolysis, but often they are in contrast with each other; a summary of the previous studies is reported in Table 6.1.
6.3 Effects of Glucocorticoids on the Differentiation of Adipose Tissue
Since several years, it is known that glucocorticoids stimulate lipolysis; people with glucocorticoid excess usually present increased fat deposits. The increased appetite sense and the lipogenic effect of the associated hyperinsulinemia caused by the high levels of GCs may explain this contradiction. Anyway, the relationship between adipogenesis and glucocorticoid hormones is very complex.
In the last 15 years, many new knowledge about the adipose tissue have been discovered. In fact, adipose tissue is discerned in visceral and subcutaneous fat. The relationship between human fat topography and metabolic syndrome was investigated at molecular level, and an important role of glucocorticoid hormones was found.
In vitro studies were performed to explain the pathway of differentiation from stem cell to adipose tissue. Significant differences of adipocyte differentiation were found between human and rodent culture cells [13].
In rodent preadipocyte cell lines, the addition of glucocorticoid in the first 48 h (expansion clonal phase) to the calf serum showed a stimulatory effect. This effect has been attributed to a direct stimulation of C/EBPδ transcription, repression of the pref-1, an antiadipogenic preadipocite factor and to the depletion of a specific subcellular pool of histone deacetylase 1 (HDAC1) [13, 14].
In human primary preadipocyte differentiation, the action of GCs is limited to the first 48 h and determines an increased level of transcription factors and adipogenic markers such as C/EBPα that is not present in rodent cells [15] (Fig. 6.2).
This early elevation does not reflect an increase in mRNA levels; in a second moment, elevated levels of C/EBPα and PPARγ are observed, and at this time, they are correlated to the mRNA levels. At day 2–4, mRNA levels of both molecules remain elevated, but protein expression of the only C/EBPα starts to decline despite the high mRNA levels. These observations could explain a different role in adipocyte maturation of PPARγ vs. C/EBPα, with a predominant role for PPARγ in mature adipocyte [13].
In vivo studies were also performed. Part of literature indicates that cortisol in humans and corticosterone in mice stimulate the adipogenesis; in fact, in obese mice, adrenalectomy decreases the rate of body weight gain, but this effect is completely reversed when the animals are treated with corticosterone [16].
Many theories have been hypothesized to explain the mechanisms at the basis of visceral obesity.
Of course, increased secretion of glucocorticoid is associated to obesity; anyway, cortisol levels are not elevated in idiopathic obesity suggesting an increased metabolism of glucocorticoid hormones.
Some authors suppose that in obesity there is an increased local active glucocorticoid concentration and that this determines a peripheral amplified activation of its receptors.
Other authors presume a pivotal role of 11-β-HSD type 1.
Disorders of this enzyme were found in obese models. In fact, 11β-HSD is impaired in obese rats and an increased local activity in visceral fat was found. The consequence of this hyperactivity is a higher concentration of active glucocorticoid responsible for visceral fat increase [17]. In the same manner, it could be speculated that in human an increased activity of 11-β-HSD plays a role in visceral obesity and metabolic syndrome.
GCs may contribute to obesity in many ways. It has been demonstrated that GCs regulate the appetite center through direct and indirect actions. Although an acute stress condition seems to suppress appetite sense, the GCs released in the hours and days after the event play a feeding role. However, recent studies have also demonstrated that, in humans, high levels of cortisol are related to the amount of ingested food 1 h after the injection of CRH and the amount of food is directly correlated to the cortisol peak.
Several studies have examined the possible interactions of GC target molecules and their mechanism on the appetite center. Various molecules have been invoked:
1.
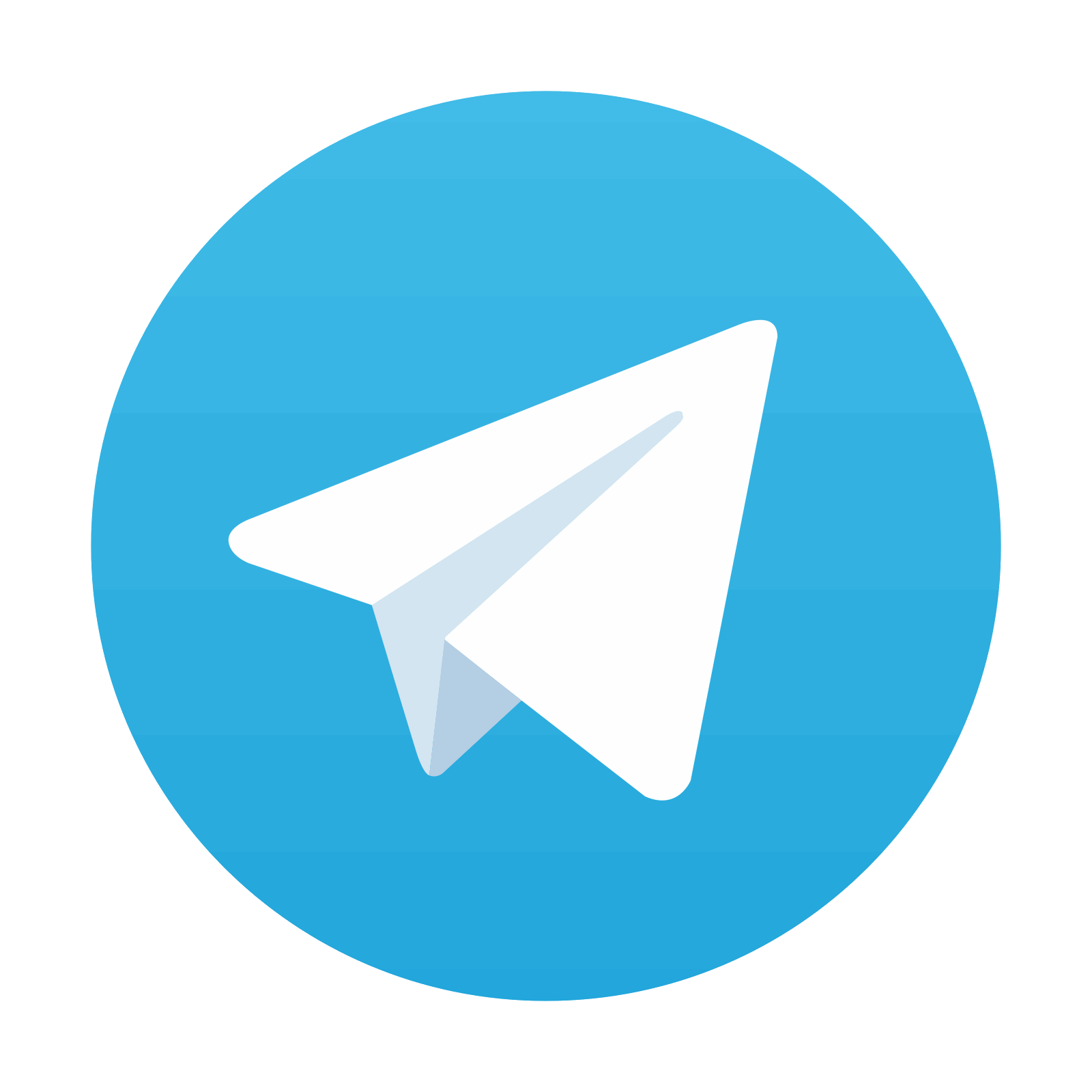
Neuropeptide Y (NPY) and agouti-related peptide (AgRP) [the gene expression of these molecules is upregulated in the arcuate nucleus by an increase of AMP-activated protein kinase signaling through GC stimulus] [18].
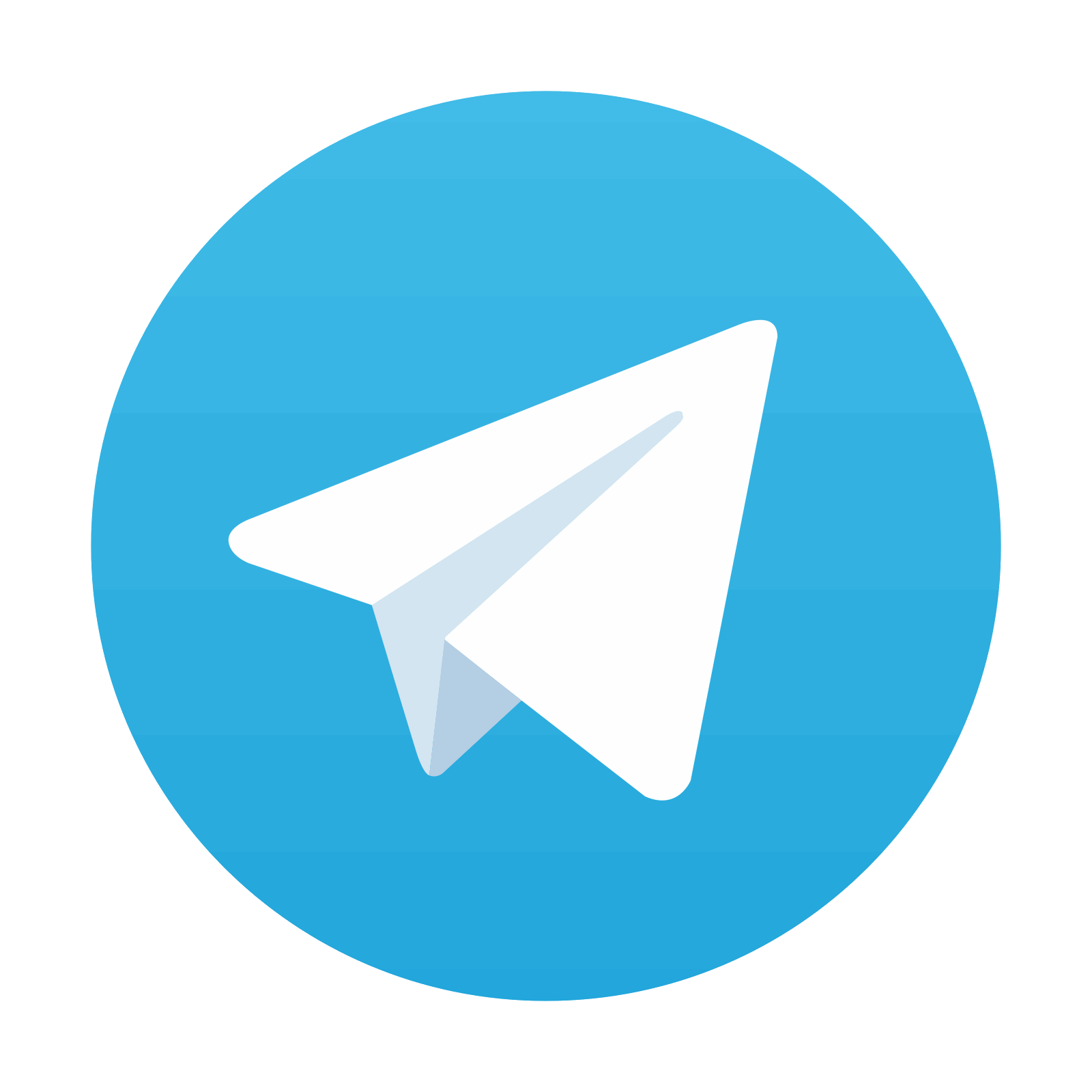
Stay updated, free articles. Join our Telegram channel

Full access? Get Clinical Tree
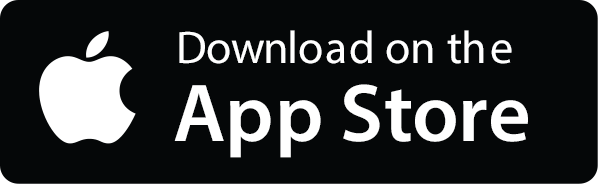
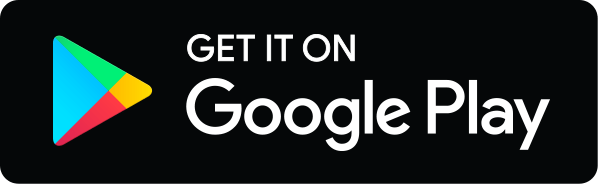
