Advantages
Disadvantages
Safety based on non-pathogenicity
Limitation of transgene cassette length (up to 5 kb)
Wide range of target tissue
Long-term transgene expression
High prevalence of neutralizing antibody
Stability of the vector capsid
Complicated procedure for vector production
Table 6.2
Major clinical trials using AAV vector
Target tissue | Diseases | Genes |
---|---|---|
CNS | Leukodystrophy (Canavan disease) | Aspartoacylase |
Alzheimer’s disease | NGF | |
Parkinson’s disease | GAD, AADC | |
Lipofuscinosis | CLN2 | |
Temporal lobe epilepsy | NPY | |
Eye | Leber’s congenital amaurosis | RFP65 |
Muscle | Muscular dystrophy | Sarcoglycan |
Cardiomyopathy | SERCA2a | |
Respiratory | Cystic fibrosis | CFTR |
Alpha-1 antitrypsin deficiency | hAAT | |
Metabolic | Hemophilia B | Factor IX |
Others | Prostate cancer | GM-CSF |
6.3 A History of AAV Vector Development
6.3.1 Discovery and Vector Development of AAV1–6 (First Generation: Naturally Occurring Serotypes)
AAV was first discovered in adenovirus stocks as a contaminating virus [1]. In the 1960s, AAV2–4 were subsequently discovered and studied intensively. As it appeared to be nonpathogenic, the interest from the virological point had been disappeared. After that, a limited number of groups investigated this virus chiefly as vectors. During that period, AAV5 was discovered from human pathological site [2] and AAV6 from laboratory strain [3]. At that time, early attempts to develop vectors from these serotypes were conducted [4–8].
6.3.2 AAV Serotypes Discovered from Monkey and Human Tissues (Second Generation: Selected Naturally Occurring Serotypes and Clones)
Following the isolation of AAV1–6, significant attempts were made to discover other serotypes. Among these, most influential studies were the development of AAV7–9 [9, 10]. AAV7 and AAV8 were isolated from monkey tissues and AAV9 from humans. As performance of these vectors were tested and selected using mice, in vivo experiments using these serotypes were likely to be successful. Many other serotypes and clones have been reported thereafter. Practically, it is not possible to test the performance of all these derivatives in vivo.
6.3.3 Development of Novel Vectors Suitable for Specific Purposes (Third Generation: Ongoing)
In order to overcome the limitations of naturally occurring serotypes and clones, a number of efforts have been made to modify the capsid domains through shuffling or mutation [11]. Although not many of these derivatives have been integrated into the research community, the use of these techniques may eventually lead to the invention of the ultimate therapeutic vector.
6.4 A Brief History of Hemophilia Gene Therapy
6.4.1 Overview of Hemophilia
Hemophilia is the largest bleeding disorder of genetic origin. Based on the responsible gene, it is named as A and B, which correspond to factors VIII and IX, respectively. As these genes are located on X chromosome, males are usually affected. Worldwide, an estimated one in every 5,000–7,000 live male births is affected by hemophilia A, while one of every 25,000–30,000 is affected by hemophilia B [12]. The disease severity is classified based on levels of blood coagulation factor (Table 6.3). Gene therapy is often beneficial in severe form patients. Hemophilia is an ideal target for gene therapy, as the supplementation of a single gene product should theoretically improve disease status. In reality, achieving this goal has proved challenging [13].
Table 6.3
Severity of hemophilia
Disease status | Coagulation activity (% of normal) |
---|---|
Mild | >5 |
Moderate | 5>, >1 |
Severe | 1> |
6.4.2 Advantages of Gene Therapy as a Therapeutic Modality
In severe form patients, regular injection of blood products is costly and painful, and such treatments may still not completely prevent further bleeding episodes. In this case, gene therapy may hold the key toward minimizing unwanted aspects while keeping the levels of coagulation factor constant.
6.4.3 Attempts Using Various Vectors for Hemophilia A
As the size of the cDNA of factor VIII is much greater than the packaging capacity of AAV, early attempts have utilized various vector systems, including plasmid, adenovirus vectors, and retrovirus vectors (Table 6.4).
Table 6.4
A list of clinical trials for hemophilia
Type | Target | Vector | Start | Cases | Outcome |
---|---|---|---|---|---|
A | Fibroblast | Plasmid | 1998 | 6 | Low efficiency |
B | Muscle | AAV type 2 | 1999 | 8 | Low efficiency |
A | Liver | Retrovirus (MLV) | 1999 | 13 | Low efficiency |
B | Liver | AAV type 2 | 2000 | 8 | Transient efficacy |
A | Liver | Adenovirus (helper dependent) | 2001 | 1 | Toxicity |
B | Liver | AAV type 8 | 2008 | 10+ | Clinical benefit |
6.4.4 Attempts Using AAV2 Vectors for Hemophilia B
Early clinical trials have targeted both the skeletal muscle [14] and the liver [15]. Although transient expression was observed in some patients, neither study resulted in significant clinical benefit. The main reason for the insufficient efficacy is now attributed to the incomplete performance of AAV2 vectors in these organs.
6.4.5 Clinical Trials Incorporating the AAV8 Vector
The next clinical trial was conducted for hemophilia B patients utilizing liver-tropic AAV8. The vector was administered intravenously to patients, and sustained level of coagulation factor was observed even the amount of blood product was decreased or discontinued [16]. Three different doses of vector were tested, and the most significant benefit was observed in the highest dose patients. Based on the success of this protocol, several new patients have been enrolled in the study at the highest dose. This success stimulated the field of hemophilia gene therapy, and it is reported that other clinical trials are currently proposed using AAV8-based vectors.
6.5 Considerations for Optimizing AAV Vector Administration
6.5.1 General Considerations
As in the case with many therapeutics, determining the optimum route of administration has been a point of emphasis for AAV vectors. While simple injection into the target tissue is sufficient in some cases, including the use in the central nervous system [17], there are several other methods and procedures that have been developed and optimized for other target organs.
6.5.2 Liver-Mediated Gene Transfer
To achieve efficient liver transduction, vector injection into the portal vein would be the obvious deliver method. However, injection into the main part of the portal vein is invasive and poses a high risk of development of difficult bleeds. Therefore, injection into a more peripheral portion, such as the mesenteric vein, is safer and preferred. In one clinical trial of hemophilia B, vector was delivered into the hepatic artery [15]. In that trial, some patients showed significant level of expression, although the duration of expression was limited. In the case of more liver-tropic vectors such as AAV8, simple intravenous injection seems to be a sufficient means for liver transduction.
6.5.3 Skeletal Muscle Transduction
6.5.3.1 Direct Injection into Muscle
The natural choice for muscle transduction would be direct injection. Indeed, this has proven efficacious in rodents; however, in larger animals there are complications. First, a robust immune response often results as the tissue injury caused by the needle provokes innate immune responses. Additionally, due to uneven distribution of the vector within muscle tissue, there is often an equally uneven transduction efficiency, which often results in incomplete posttranslational modification. Obviously, it is impossible to transduce entire muscle tissues by this method. In an early clinical trial for hemophilia B, vector was administered intramuscularly at over 100 sites in the highest dose patients [14]. Intramuscular injection was discouraged as a route for clinical use especially after the demonstration of anemia due to immune reaction against erythropoietin, which was the original transgene aiming to promote red blood cell formation [18, 19].
6.5.3.2 Other Methods for Muscle Transduction
Whole-muscle transduction in vivo has been reported using adult mice [20]. This method utilized simple IV injection of AAV6-mediated vectors. At first, VEGF was administered simultaneously to enhance vascular permeability. Subsequently, it was shown that VEGF was not essential if the vector dose was higher by ten times. Detailed analysis revealed widespread transduction of muscle tissues throughout the body. The only drawback of this method was the necessary vector dose (1013 vg per mice), which accounts for nearly 100-fold excess of regular applications. Although various attempts have been made to reduce the vector dose, this method still requires much higher vector dose than regular approaches and therefore hard to translate.
6.5.3.3 Limb Perfusion
In the field of cancer treatment, this technique has been developed to maximize therapeutic efficacy by concentrating the delivery of chemotherapeutic agents. A tourniquet is applied to cease blood flow through the local arteries and veins, and then vector is injected intravenously into the isolated area. Histamine or other drugs may be included to promote vessel permeability. This method is effective for the muscles of extremities; however, it is not feasible to apply this technique for muscles of the trunk, head, and neck area [21]. Moreover, as this approach requires intensive exposure of the vector to the blood supply, presence of NAb against the vector capsid also diminishes the efficacy of such a treatment (see next section for details).
6.6 Neutralizing Antibody (NAb) Against AAV Vector
6.6.1 The Impact of NAb Upon AAV-Mediated Gene Transfer
The presence of neutralizing antibodies against vector capsid is one of the most important issues in AAV-based therapies, especially for liver transduction in vivo [22, 23]. The antibody is considered as a consequence of natural infection. As wild-type AAV may distribute on earth, worldwide prevalence of NAb against AAV would be of interest.
6.6.2 Prevalence of NAb Against AAV Serotypes Around the World
The actual procedures for NAb measurement is described in Fig. 6.1. Several studies illuminated prevalence of NAb against various AAV serotypes in different locations around the world [24–31]. The results indicate that a fraction of the population possesses NAb against all serotypes tested. Given the complexity of this assay, there is some inherent variability, although it is clear from the findings that NAbs are prevalent and pose a serious challenge for AAV-based therapies in every country tested.
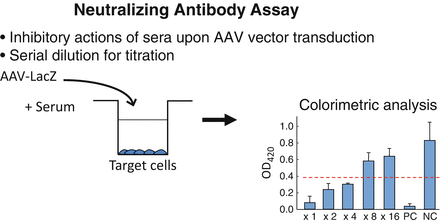
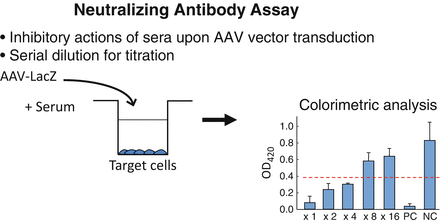
Fig. 6.1
Assay for NAb
6.6.3 Analysis of Japanese Population
We tested the prevalence of NAb in Japan in both healthy and hemophilia populations [31]. Our results indicate that the overall prevalence in Japanese population is similar level to the rest of the world. There was not a significant difference between the healthy population and the hemophiliacs. Surprisingly, there was a huge difference in the prevalence if the population was classified by age; those who were born before 1970 showed remarkably high prevalence (80 % or higher) in both groups (Fig. 6.2). On the other hand, younger populations showed low prevalence with a decreasing trend year by year. We speculate that wild-type AAV is on the way of elimination, presumably by the improvement of public hygiene status. Our results indicate that the proportion of NAb-negative population will increase over the years, although another round of study is necessary in the different time point to warrant the conclusion.
