17 Cholangioscopy
David Lee, Juliana Yang, and Ali A. Siddiqui
17.1 Introduction
The direct visualization of the biliary tree has long been out of reach for endoscopists. However, with recent advances in technique and equipment, cholangioscopy is gaining increased adoption for a wider variety of indications. From the direct visualization of benign versus malignant strictures to the removal of large or complicated biliary stones, cholangioscopy has become an essential tool in the toolkits of many endoscopists. In this chapter, we describe the recent advances which have led to the modern cholangioscope, as well as its most utilized indications. We also describe the variety of instruments available for use in conjunction with the cholangioscope, as well as the potential complications which can arise in cholangioscopy.
17.2 Overview of Cholangioscopy
In the modern era of endoscopy, the management of pancreaticobiliary ductal diseases utilizes multiple modalities, including endoscopic retrograde cholangiopancreatography (ERCP), endoscopic ultrasound (EUS), intraductal ultrasound (IDUS), and magnetic resonance cholangiopancreatography (MRCP). 1 , 2 , 3 These modalities are vital to the delineation of anatomy and evaluation of pancreaticobiliary ductal disease. 4 However, such imaging modalities are indirect methods and therefore suffer from the common problem of not allowing for direct visualization of biliary mucosal abnormalities. 1 , 5
Peroral cholangioscopy is a noninvasive endoscopic method that is increasingly being utilized as a tool for the diagnosis and treatment of various biliary disorders. 6 , 7 , 8 Peroral cholangioscopy is performed by using a cholangioscope that is advanced through the duodenoscope accessory channel, or by direct insertion of a small endoscope into the bile duct. With the introduction of the SpyGlass direct visualization system, peroral cholangioscopy has now become universally adopted for the diagnosis and therapy of biliary tract disease. Studies have demonstrated the improved clinical efficacy of peroral cholangioscopy in characterizing benign versus malignant natures of biliary strictures, diagnosing intraductal tumors, better defining unknown biliary pathologies, and treating difficult to remove biliary stones. 9 , 10 , 11
17.3 General Diagnostic Techniques
17.3.1 Two-Operator Systems: Mother-Baby Scopes
The first cholangioscope was described in 1941 and was used to exclude choledocholithiasis after cholecystectomy. 1 Twenty years later, this equipment was refined into a flexible choledochoscope that would gain access via a percutaneous, transhepatic approach. The peroral approach was introduced in the early 1970s, 12 , 13 , 14 utilizing a “mother-baby” system which required two endoscopists and a cumbersome operating system. 4 , 15 , 16 , 17 The “mother-baby” system (Olympus America, Center Valley, Pennsylvania, and Pentax, Montvale, New Jersey) was developed by Takekoshi et al, Nakajima et al, and Rosch, and had a smaller cholangioscope (“baby”) that passed through the instrument channel of a larger duodenoscope (“mother”). 18 This technology allowed for direct visualization of the pancreaticobiliary system, but was plagued by poor image acquisition, high cost, and lengthy procedures. These procedures were notorious for being very labor intensive and technically challenging (▶Table 17.1).
17.3.2 Single-Operator System: SpyGlass Cholangiopancreatoscopy
Peroral cholangioscopy became more widespread following the introduction of the SpyGlass direct visualization system (Boston Scientific, Natick, Massachusetts, United States) in 2006, which improved the prior two-person (“mother-baby”) operating system into a single-operator system. 18 The design of the system involves a SpyScope access and delivery catheter which is a single-operator, disposable catheter controlled by two dials allowing for four-way steer. It is 230 cm in length and has four channels: one 1.2-mm instrument channel, two 0.6-mm air and irrigation channels, and a 0.9-mm channel for fiberoptic probes. 8 , 16 , 19 , 20 These channels allow for simultaneous air insufflation and irrigation. The SpyGlass access catheter measures 10 F (3.3 mm) in outer diameter, and is a multiple-use device which conducts light to biliary ducts and transmits fiberoptic endoscopic images. It has a lens connected at the distal end of the image bundle that visualizes a 70-degree field of view.
In 2014, the new SpyGlass DS direct visualization system (Boston Scientific, Natick, Massachusetts, United States) was launched. This system consists of a fully integrated SpyScope DS access and delivery catheter, a single-use scope to eliminate probe reprocessing and image degradation (▶Fig. 17.1). It also has an integrated digital sensor that now allows for superior imaging, improved resolution, and a 60% wider field of view as compared to the first-generation system. At the time of this writing, there are only limited case reports published using the SpyGlass DS system, but the body of available literature is expected to expand greatly as this second-generation system gains adoption over the first-generation SpyGlass system (Video 17.1, Video 17.2).

17.3.3 Direct Cholangioscopy
With direct peroral cholangioscope (DPOC), an ultraslim (5–6 mm) upper endoscope is inserted via the mouth, advanced to the duodenum, and then manipulated across the major papilla and into the bile duct for evaluation of the mucosa and the lumen of the biliary tract (▶Fig. 17.2). 21 It requires the presence of biliary sphincterotomy and/or sphincteroplasty (papillary balloon dilation) in order to maneuver the scope into the bile duct. Upon initial insertion, there is often a significant amount of looping of the endoscope, especially in the gastric fundus and the duodenum, 21 and insertion of a guidewire into the bile duct is often required prior to introducing the scope so as to give the endoscope traction. Because of the difficulty in performing DPOC, this technique is rarely utilized outside of specialized or academic settings (Video 17.3).

An anchoring balloon has been trialed previously which would fix inside the intrahepatic duct branch to facilitate the advancement of the ultraslim scope. 20 , 21 Preliminary data indicated positive results, increasing the success rate from 45 to 95%. However, this device was voluntarily withdrawn from the market by the manufacturer due to concerns of increased risk of fatal air embolism. 21 , 22
17.4 Accessory Devices and Techniques
17.4.1 Confocal Microscopy
The most recent development in cholangioscopy is probe-based confocal laser endomicroscopy (pCLE). This technology uses a flexible probe-based confocal aperture to focus light on a single spot. High contrast imaging is obtained by injecting a volatile organic compound, such as fluorescein, which in turn stains the extracellular matrix of the bile duct epithelium and allows for real-time microscopic images of the bile duct mucosa to be obtained. Abnormalities of the biliary epithelium, such as vascular congestion, dark granular patterns, thickened reticular structures, increased interglandular space, as well as blood flow and contrast uptake, can be directly visualized with this technique. This technique has been shown to be more sensitive and accurate at diagnosing cholangiocarcinoma in patients with indeterminate biliary strictures than conventional ERCP with tissue sampling (▶Fig. 17.3). 23 The pCLE technique is currently cited in the American Society of Gastrointestinal Endoscopy (ASGE) guidelines as a useful option in the work-up of biliary malignancy. 24

17.4.2 Lithotripsy Probes
One of the most useful aspects of cholangioscopy is the ability to directly visualize and break up large bile duct stones. Several accessory devices are available from which the endoscopist may select for this purpose, including laser lithotripsy (LL) and electrohydraulic lithotripsy (EHL) systems. These accessories may be passed through the working channels of a cholangioscope (▶Fig. 17.4).

Several laser lithotripsy systems have been approved by the Food and Drug Administration (FDA) in the United States for biliary and pancreatic ductal indications. These probes focus a high-density laser light on the surface of a stone, locally creating a plasma bubble that propagates as compressive shock waves, shattering the adjacent stone. 25
Holmium: yttrium aluminum garnet (YAG) lasers focus light in the near-infrared spectrum (2,100 nm), with pulses in the range of 500 to 1,000 mJ. 25 , 26 Typical power settings range from 0.6 to 1 J at 6 to 10 Hz, with a typical lithotripsy procedure using total laser energy of around 12 kJ. 25 , 27 , 28 Direct visualization is usually recommended to prevent inadvertent ductal damage. Holmium:YAG lasers have shown good efficacy in clearance of both biliary and pancreatic stones. 29
Another popular system for laser lithotripsy is the so-called frequency-doubled, double-pulse neodymium:YAG (FREDDY) system. The FREDDY system uses a dual laser pulse. The recommended initial settings are 120 mJ single pulse at 3 to 5 Hz, increasing up to 160 mJ and 10 Hz if needed. The major purported advantage of the FREDDY system is that the green laser is absorbed by bile stones and not surrounding tissue, thereby minimizing the potential for ductal damage. 25
EHL systems are comprised of a charge generator unit and a bipolar probe. A charge is released across the two electrodes of the probe, resulting in a spark and a hydraulic shock wave which propagates to shatter the adjacent stone. Optimal positioning of the probe tip is about 1 to 2 mm from the stone, about 5 mm from the tip of the endoscope, and aimed directly at the stone. 25 EHL does require the use of continuous saline irrigation to provide a medium for shock wave propagation, as well as to clear debris. 25 EHL systems too have shown good effect in stone clearance. 6
17.4.3 Intraductal Biopsy Forceps
Cholangioscopy-directed biopsy to target indeterminate strictures of the biliary tract can be performed by passing a miniature Spybite biopsy forceps through the working channel under direct endoscopic visualization. The instrument is 270 cm in length, and has an outer diameter of 1 mm. The forceps’ jaw can open to a 4.1-mm diameter at a 55-degree angle, and has a central spike which allows for targeted biopsies under direct visualization.
Another technique entails cholangioscopy-assisted biopsy by which the target biopsy site is first localized using cholangioscopic visualization, after which a conventional biopsy forceps is then passed through the working channel of the duodenoscope in order to obtain tissue samples under fluoroscopic guidance (▶Fig. 17.5).

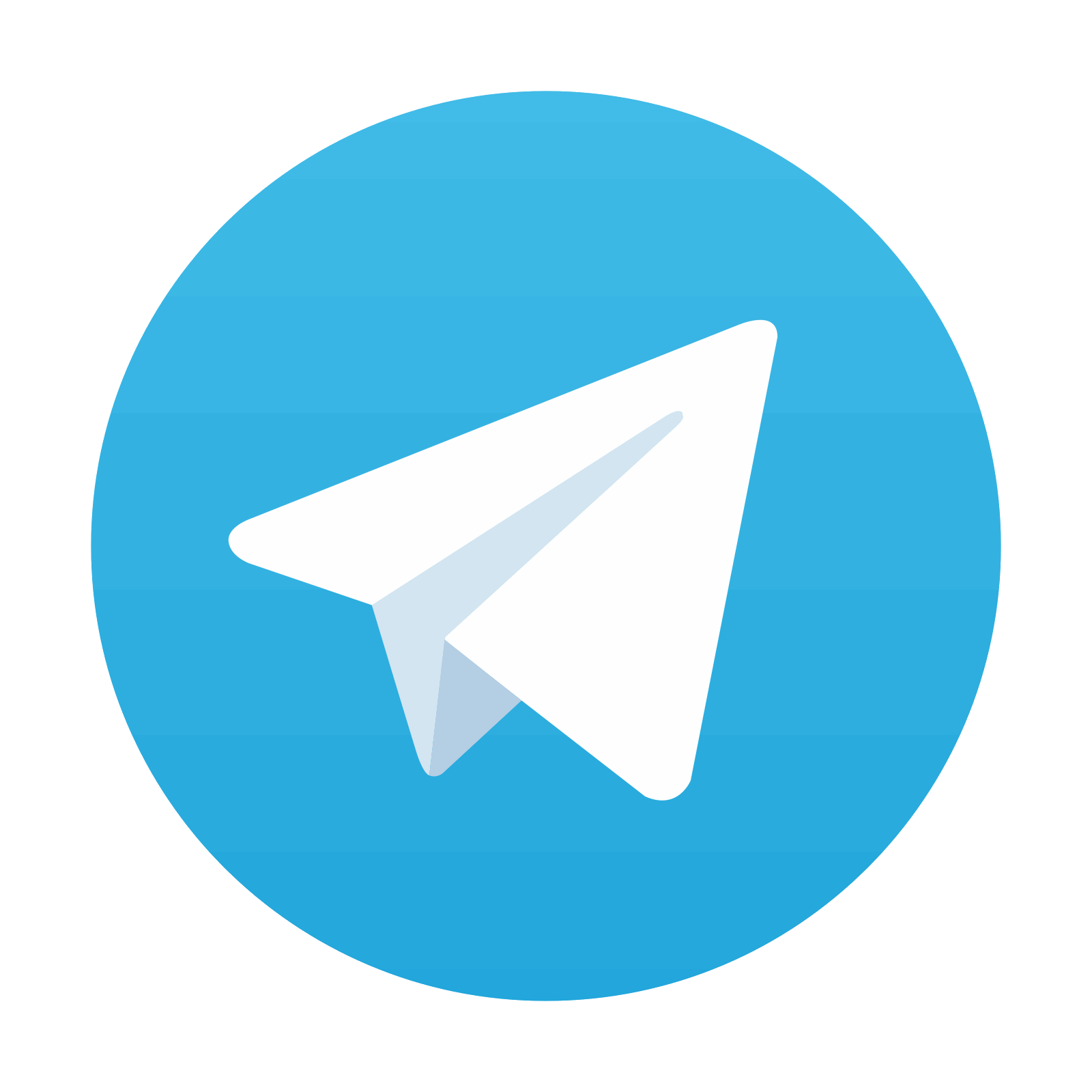
Stay updated, free articles. Join our Telegram channel

Full access? Get Clinical Tree
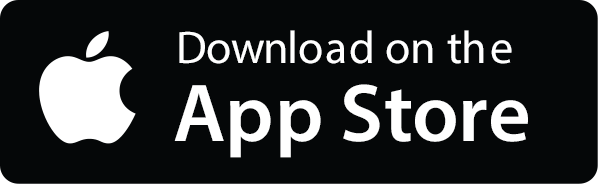
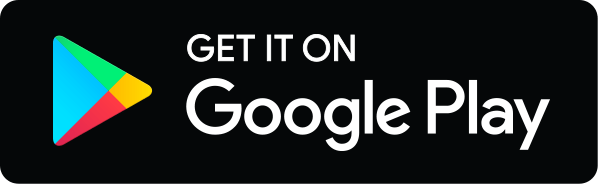
