disease will manifest clinically primarily with liver disease, especially in children and younger adults, typically between 5 to 35 years old. However, initial presentation as severe liver disease has been reported in children <2 years old and in older adults.2,3 The clinical presentation of liver disease varies significantly and may include recurrent jaundice because of acute or chronic hemolysis, acute self-limited hepatitis, autoimmune hepatitis, fulminant hepatic failure, chronic liver disease with portal hypertension, or fatty liver disease. Kayser-Fleischer rings owing to copper deposition in Descemet’s membrane of the cornea can be observed in 50% to 90% cases.
findings can range from almost normal liver parenchyma to massive necrosis. The histopathologic changes can be categorized into the following patterns:
and simple and fast staining techniques. Orcein and Victoria blue stains are not specific for copper, as they detect copper-associated proteins in lysosomes, which may or may not contain copper. The Timm silver sulfide and rubeanic acid stains are more sensitive than Rhodanine but are not widely used. The Timm silver sulfide stain needs a longer (24-hour) deparrafination time, and rubeanic acid requires a 72-hour incubation to reach the best results.6,7
all liver biopsies when working up the etiology of unexplained liver disease, especially in children and younger adults. Several patterns can raise suspicion for Wilson disease, including fatty liver disease in younger individuals with no risk factors, cryptogenic cirrhosis in young or middle aged adults with no risk factors, or an acute unexplained hepatitis or acute liver failure in young individuals. Even in cases where the clinical and pathological findings suggest autoimmune hepatitis, clinicians should still be reminded to rule out Wilson disease.
Table 18.1 Inclusions, Deposits, or Crystals in Live of Common Genetic Disorders | |||||||||||||||||||||||||||||||||||||||||||||||||||||||||||||||||||||||||||||||||||||||||||||||||||||||||
---|---|---|---|---|---|---|---|---|---|---|---|---|---|---|---|---|---|---|---|---|---|---|---|---|---|---|---|---|---|---|---|---|---|---|---|---|---|---|---|---|---|---|---|---|---|---|---|---|---|---|---|---|---|---|---|---|---|---|---|---|---|---|---|---|---|---|---|---|---|---|---|---|---|---|---|---|---|---|---|---|---|---|---|---|---|---|---|---|---|---|---|---|---|---|---|---|---|---|---|---|---|---|---|---|---|
|
transplantation is not recommended in those cases with severe neuropsychiatric illness, as its benefit in this setting is uncertain.
![]() Figure 18.2 Wilson disease. Autoimmune hepatitis-like pattern with marked lymphoplasmacytic infiltrate, interface activity, and necrosis. |
Tyrolean infantile cirrhosis, and idiopathic copper toxiocosis. Most individuals with these diseases present before the age of 2 years with histories of progressive lethargy, increased infections, and hepatomegaly. The etiology of these diseases is still not fully understood and both genetic defects in copper metabolism and excessive copper intake may have roles in the excess copper accumulation. The histologic changes in the liver appear to be similar in these diseases, though published descriptions remain sparse. Essentially all cases are diagnosed at the cirrhotic stage and characteristically have a micronodular pattern composed of very tiny nodules. The lobules typically show marked hepatocyte ballooning degeneration, abundant Mallory body formation, and scattered acidophil bodies. Steatosis is typically absent. The inflammation is mild and composed of lymphocytes, histiocytes, neutrophils, and a few plasma cells. Cholestasis can be prominent. The copper accumulation starts in periportal hepatocytes and then extends to the entire lobules and is typically marked and diffuse by the time the liver is cirrhotic. In addition to cirrhosis, the trichrome stains can show marked central vein fibrosis and marked pericellular fibrosis.
extrahepatic biliary atresia. The liver biopsy can show marked cholestasis, bile ductular proliferation, mild portal and lobular inflammation, periportal steatosis, and occasional giant cell transformation (Fig. 18.12). Variable degrees of fibrosis are often present and occasional cases may have bridging fibrosis or even cirrhosis in the initial biopsy, which is indicative of rapid progression of liver disease.
![]() Figure 18.7 α-1-Antitrypsin deficiency. Round intracytoplasmic α-1-antitrypsin globules in variable sizes seen on H&E section as eosinophilic globules. |
![]() Figure 18.9 α-1-Antitrypsin deficiency. Bright magenta PAS-positive globules with diastase resistance. |
![]() Figure 18.10 α-1-Antitrypsin deficiency. The globules may not be recognizable on routine H&E section. |
![]() Figure 18.11 α-1-Antitrypsin deficiency. The globules are readily revealed by PASD stain in the same case showed in Figure 18.10. |
granular particles but the granules are smaller than A1AT globules, are not eosinophilic on H&E and are reddish brown color on PASD stains (Fig. 18.17). Macrophages can contain large granules, mimicking A1AT globules on PASD stain (Fig. 18.18). Immunoglobulin globules are round and eosinophilic on H&E staining and can be highlighted by immunoglobulin G (IgG) or immunoglobulin M (IgM) immunostains (Fig. 18.19). In most cases, these result from elevated serum immunoglobulin levels, so are found in autoimmune hepatitis, primary biliary cirrhosis, and other conditions associated with high serum immunoglobulin levels. The fibrin globules seen in fibrinogen storage disease are often larger than A1AT globules and are PASD-negative. They sometimes will contain dark cores and can be highlighted by phosphotungstic acid hematoxylin (PTAH) stains (Fig. 18.20) or immunostains for fibrinogen.
![]() Figure 18.19 IgG globules (arrows) in macrophages in autoimmune hepatitis (inset, IgG immunohistochemistry). |
4, FGA, FGB, and FGG. In afibrinogenemia, the fibrinogen levels are less than 0.1 g/L and manifests clinically primarily as bleeding, which can range from mild to severe. Patients with hypofibrinogenemia are usually asymptomatic, with no spontaneous bleeding episode, or have mild bleeding. Both can also be associated with thrombosis. Laboratory testing shows abnormal coagulation tests with low or absence of fibrinogen. Replacement of the fibrinogen is the main treatment.
![]() Figure 18.22 Afibrinogenemia. Abnormal fibrinogens stained black with phosphotungstic acid hematoxylin (PTAH) stain (inset, inclusions negative for α-1-antitrypsin immunohistochemistry). |
![]() Figure 18.23 Glycogen storage disease type I. Diffusely enlarged hepatocytes with pale-staining cytoplasm and centrally located pyknotic nuclei. |
is common in type IV glycogen storage disease and some cases may develop cirrhosis.
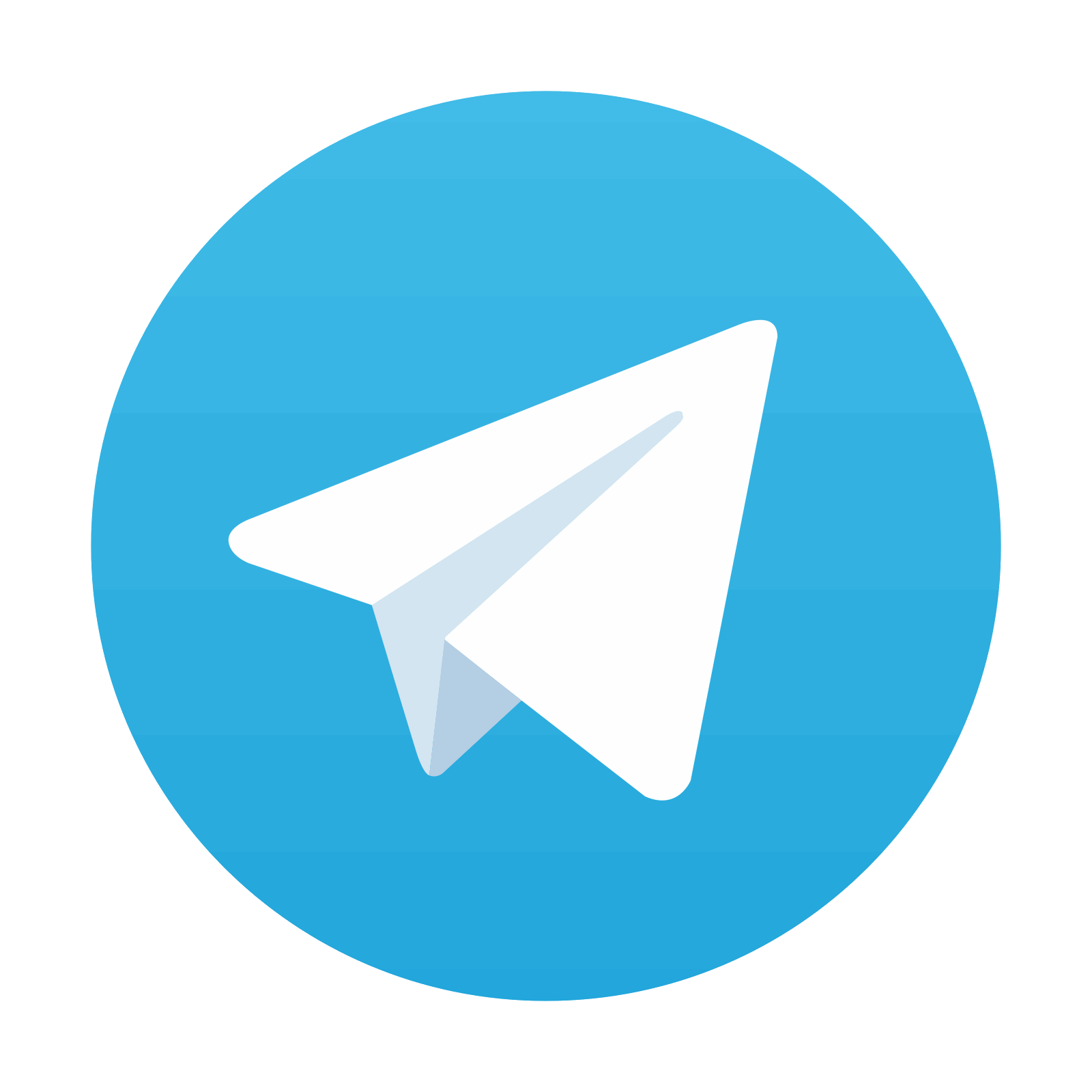
Stay updated, free articles. Join our Telegram channel

Full access? Get Clinical Tree
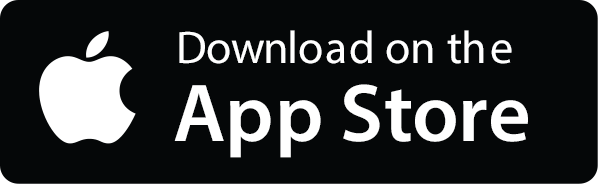
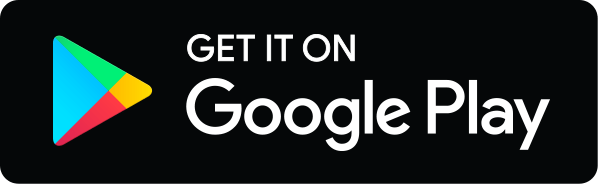
