(1)
The James Buchanan Brady Urological Institute and Department of Urology, The Johns Hopkins School of Medicine, 800 Orleans Street, Marburg 420, Baltimore, MD 21287, USA
(2)
Department of Urology, University of Chicago, Chicago, IL, USA
(3)
Department of Urology, Albert Einstein College of Medicine, New York, NY, USA
(4)
Department of Urology, The Johns Hopkins Hospital, Baltimore, MD, USA
Keywords
Stem cellsUrotheliumRegenerative medicineTissue engineeringScaffoldIntroduction
There is a large interest in developing tissue-engineered urinary diversions (TEUDs) in order to reduce the significant morbidity that results from utilization of the alimentary tract in the urinary system. The gastrointestinal tract has been used for urinary diversion for over 150 years with the first reported ureteroproctostomy by Simon in 1858 [1]. Although it is also a tubular structure designed for material transport, the absorptive qualities of the alimentary tract result in significant morbidity when exposed to urinary waste. Invariably, some degree of metabolic disequilibrium and anorexia develop, in addition to the operative sequela of bowel surgery. Additional long-term sequela may include urolithiasis, infection, disruption of the enterohepatic circulation, anemia, and chronic metabolic acidosis. Furthermore, incorporated bowel segments do not have the mechanical properties and innervation necessary to recapitulate the complex function of coordinated urinary storage and emptying.
Given the clear need for a new approach, it is no surprise that reconstruction of the urinary tract has been sought after since the early years of tissue engineering [2, 3]. There are four essential components of any tissue engineering program to replace a whole organ:
- 1.
Identification of the most appropriate biomaterial
- 2.
Identification of a cell type for seeding of the biomaterial/scaffold
- 3.
Creation of a stimulus from host microenvironment or from implanted cells
- 4.
Identification of a blood supply to bring nutrients, oxygen, and growth factors for the tissue-engineered organ to maturate and regenerate
Studies continue to identify novel scaffold materials and cell populations that are combined to generate TEUDs. Scaffold composition ranges from synthetic material to decellularized bladder tissue. Cell types vary from fully differentiated adult populations such as smooth muscle cells isolated from the bladder to stem cell populations including mesenchymal stem cells and induced pluripotent stem cells. Each scaffold and cell type has its advantages and disadvantages with no clear superior component having been identified. Recent clinical trials have been disappointing, supporting the need for additional investigation. The successful marriage of these components into a biologically successful tissue-engineered organ is challenging. The various types of scaffolds and cells that are currently showing clinical promise in urinary diversion strategies will be reviewed.
Scaffolds
As its name implies, scaffolds provide the backbone for TEUDs. Cells grown in culture do not have enough structural integrity to be used by themselves, although sheets of cells have been used to treat injury to native bladder tissues [4, 5]. The ideal scaffold provides mechanical support for cells to engraft, completely degrades over time, and elicits minimal to no foreign body reaction while performing the complex physiological task of storing and voiding urine [6]. Cells can be seeded or migrate in from neighboring host tissues. To do so, they need the proper cues to aid cells in differentiating and organizing themselves to serve their physiological function, i.e., urothelium to form on the luminal surface and smooth muscle within the walls [7]. These cues can come from the inherent properties of the material used or from growth factors incorporated into the scaffold [8]. For example, collagens comparable to those that make up the basement membrane of the bladder can be used in guiding urothelial cell attachment and expansion [9].
Biomaterials for TEUDs can be organized into three general categories:
- 1.
Acellular tissue matrices, such as bladder and small intestinal submucosa
- 2.
Natural materials, such as collagen, alginate, and silk
- 3.
Synthetic polymers, such as poly(glycolic acid) (PGA) and poly(lactic acid) (PLA)
There are advantages and disadvantages to each. Acellular tissue matrices maintain the complicated microstructure organization of the extracellular matrix optimized by nature for cell engraftment. They contain the natural mixture of structural proteins including laminin, collagens I and IV, glycosaminoglycans, and embedded bioactive proteins including transforming growth factor beta 1, vascular endothelial growth factor, and fibroblast growth factor [8, 10–12]. Their drawbacks include tissue harvesting and the inherent complications and cost thereof from cadavers or animals. This also makes the material heterogenic, which may affect their structural integrity and how consistently the products are implemented. They often do not have sufficient rigidity and collapse when used in tubular applications [13]. Acellular matrices can also induce a significant inflammatory reaction, which is influenced by the source of material and how it is processed [14].
Scaffolds derived from natural materials can be fabricated to fit a particular application. Materials are chosen based on the ease of their manipulation and their similarity to the extracellular matrix they are replacing. The most commonly used materials for TEUDs are collagen, alginate, and silk, with collagen being the most common of the three (Fig. 7.1). Structural strength and cell seeding ability are greatly impacted by how the scaffolds are constructed and often are competing variables. A tightly woven collagen lattice may have excellent tensile strength but may be suboptimal for cell implantation, which require porosity to allow for cellular movement and nutrient diffusion [15–19]. Scaffolds can be engineered to have a mix of substrates (such as collagen I and elastin) to further modify the mechanical properties as well as incorporate various growth factors to promote cell incorporation [20].


Fig. 7.1
(a) Collagen I-based scaffold molded into a tube. (b) Scanning electron microscopy cross section of molded collagen scaffold demonstrating laminar organization of collagen filaments. Scale bar represents 20 μM
Synthetic scaffolds can be precisely constructed given the consistency and predictability of the synthetic polymers they are derived from. This lends a high degree of consistency between scaffolds [16, 21]. The most commonly used synthetic polymers are PGA, PLA, polyanhydrides, poly(ortho esters), and poly(lactic co-glycolic acid) (PLGA). PGA, PLA, and PLGA are FDA approved and have been used in the clinical arena for decades as suture material [22]. Synthetic scaffolds can incorporate growth factors and a variety of building components including natural materials (i.e., collagen and elastin) to augment their physical and cell engraftment properties. Synthetic scaffolds can elicit a foreign body reaction. This is due, in part, to the release of acidic byproducts when they are degraded [23]. New materials that do not form acidic by products are being actively investigated. No ideal scaffold material or combination of materials has been identified. This is a quickly evolving field, and many of the issues encountered are likely to be addressed by advances in material science and greater understanding of what cells need to successfully engraft TEUDs.
Why Are Cells Needed?
Early studies in animals demonstrated the importance of incorporating cells into implanted scaffolds [24, 25]. In a rabbit model of urethral replacement, unseeded tubular collagen matrices were uniformly structured, whereas collagen matrices seeded with autologous bladder cells did not. Histological analysis of the seeded grafts demonstrated a normal urethral architecture 1 month following implantation including intact innervation and the capacity for contractility in organ bath studies [24]. Similarly, bladder augmentation in rats with unseeded scaffolds was shown to have reduced overall cellularity and lack of complete epithelialization compared to grafts seeded with fibroblasts [25]. Although incorporating cells adds challenges of tissue harvest, cell expansion, and risk of rejection if using allogenic material, there are important benefits to integrating cells into TEUDs [26]. The seeded cells “jump-start” the process of replacing injured tissue when compared to unseeded scaffolds (Fig. 7.2). Incorporated cells facilitate neoangiogenesis, protect the graft from caustic urinary waste, and signal and facilitate incorporation of host tissues into the graft [25, 27].


Fig. 7.2
Seeded collagen scaffolds. (a) Hematoxylin and eosin (HE) staining of seeded collagen scaffold showing cellular layering on exterior surfaces of scaffold after 3 days of seeding (40×). (b) HE staining demonstrating layering of cells on external and internal surfaces of collagen-based scaffold following 7 days of seeding (100×). (c) Membrane dye immunoflourescent imaging of adipose-derived stem cells (ADSCs) growing on a collagen-based scaffold. Green demonstrates viable cells; red demonstrates nonviable cells (100×). (d) Scanning electron microscopy of ADSC on the surface of a collagen-based scaffold. Scale bar represents 10 μM
Characteristics of the Ideal Cell Type
No ideal cell type has been identified for use in TEUDs. This is, in part, due to the unique properties required by the chosen cell type. Indeed, it is likely that one cell type may not suffice but a combination of cells will be used to recapitulate the human urothelial tract. The two main cell types that constitute the bulk of native human urothelium are (1) urothelial cells (UCs), which are epithelial in origin and form an impermeable barrier to allow for the transport and storage of toxic urinary waste, and (2) smooth muscle cells (SMCs), which provide structure and strength and allow for contraction and relaxation of genitourinary structures. Other important cell types include neurons, fibroblasts, and immune cells. In addition to performing these physiological functions, the cell type(s) must be amenable for tissue engineering. Specifically, they must be (1) simple to obtain, (2) have proliferative potential allowing expansion in culture, and (3) elicit little to no immune response when applied in recipient tissues. These multifaceted characteristics often juxtapose each other, i.e., performing a differentiated physiological function while being expandable in culture, which has made identification of the ideal cell type(s) difficult.
Ideally, engineered tissues would arise from the recipient in order to avoid tissue rejection and to mitigate the risk of disease transmission. However, harvesting tissue from the recipient imposes several limitations. There is the availability and morbidity associated with tissue harvest. A significant proportion of urinary diversion procedures are in context of urothelial cancer. In this setting, it is not acceptable to use recipient urothelial tissues. Additionally, tissue expansion prior to implementation requires significant effort and time. This adds to treatment complexity by requiring coordination of tissue harvest, ex vivo TEUD development, and definitive surgical repair, therefore precluding off-the-shelf availability. Importantly, not all recipients are the same nor is the quality of their tissues. Inherent patient characteristics including age, common comorbidities, such as diabetes and cardiovascular disease, tobacco use, and genetic abnormalities may reduce engineering tissue capacity, introducing variability in TEUD quality and reducing successful implementation [28–32].
Cell proliferative capacity is both an inherent function of the cell and influenced by culturing conditions. Differentiated cells such as UCs and SMCs have a limited replication potential in culture. However, manipulation of culturing conditions including serum-free media and enzyme-free techniques has allowed culturing of human UCs to 16 passages allowing the expansion in culture of a biopsy-sized specimen to that of a football field [18, 33–35]. Although they expressed differentiation markers consistent with urothelium, such as cytokeratin 7, they had a non-barrier-forming phenotype suggestive of a progenitor cell-like state [35]. They formed multiple layers consistent with fully differentiated uroepithelium when seeded onto scaffolds, which were implanted into animals. Stem cells are characterized by their ability to self-renew, potential to differentiate into various tissue types, and ability to form clonal populations without difficulty [36]. They have been under intense investigation for their use in TEUDs given their inherent characteristics. The various cell populations will be expanded upon below.
Cellular immunogenicity plays a critical role in tissue engineering applications. With or without cells, scaffolds can elicit an immune response, which the incorporated cell population can further exacerbate or attenuate. Autologous cells produce minimal to no immune response and do not trigger tissue rejection. Allogenic differentiated tissues harbor major histocompatibility complexes (MHCs), similar to organ transplantation, and are subject to the same rejection process. Several stem cell populations, most notably mesenchymal stem cells (MSCs), have been shown to have an immune modulatory capability and are immune evasive allowing allogenic transplantation without the need for powerful antirejection medications [37]. This has been shown to be in part to expression of inducible nitric oxide synthase (iNOS in mouse MSCs), indoleamine 2,3-dioxygenase 9 (IDO in human MSCs), prostaglandin-E2, interleukin-10, hemeoxygenase-1, and programmed cell death 1 ligand [38]. However, host antibodies against allogenic MSCs have been shown to develop, and it is unclear how these will play a role in MSC-seeded TEUDs that become a permanent part of the host [37]. MSCs have been shown to lose their immune-privileged status with differentiation, which has been attributed to altered expression including upregulation of interleukin-6 and altered expression of their MHCs [39, 40].
Cell Types
Urothelial Cells
Urothelial cells have been cultured for well over 40 years [41]. Normal urothelium is a transitional epithelium comprised of three layers: (1) superficial layer of umbrella cells, which establish an impermeable barrier to urine; (2) the intermediate layer; (3) and the basal layer, which is a single-cell layer in contact with the basement membrane [42]. An agreed upon, well-demarcated stem cell that can recapitulate all tissues of the bladder has yet to be identified [43]. The basal cell layer is generally considered the source of urothelial progenitor cells and is capable of rapid proliferation in the setting of injury [44–46]. More recent studies have identified a subpopulation of basal cells that are cytokeratin-5 and P63 positive and use the hedgehog/wnt pathway to recapitulate urothelial tissues [43, 44, 47]. The cytokeratin-5 population has not been evaluated for TEUD applications. Typically, UCs are obtained by tissue biopsy in humans and bladder digestion in animals [48]. Autologous UCs can be used for noncancer-related TEUDs.
Smooth Muscle Cells
Whereas the urothelium provides a barrier from urine, SMCs provide structural integrity and the ability for the bladder to contract and relax. They are not frequently used as a sole cell population in TEUDs and are often co-seeded with UCs. They are commonly harvested by bladder biopsy in humans and by bladder digestion in animal studies [24, 49–52]. In order to avoid a urinary source, SMCs have also been isolated from other tissues including adipose and peripheral blood [53]. Additionally, as mentioned earlier, smooth cells have been derived from other cells, notably MSCs [17, 54–56]. SMCs from native bladder have been shown to migrate into implanted acellular grafts; however, it incompletely repopulates the grafts and takes 4 weeks [11].
Stem Cells
Given their proliferative nature and plasticity, stem cells have been under intense investigation for use in TEUDs [57]. They are broadly characterized as embryonic stem cells (ESCs), adult stem cells, or induced pluripotent stem cells (iPSCs). ESCs are derived from the inner cell mass of blastocysts less than a week after ovum fertilization [58, 59]. They are pluripotent allowing them to form tissues from all three germ layers – ectoderm, mesoderm, and endoderm. Classic teaching dictates that the bladder trigone develops from the mesoderm and the remainder of the bladder develops from the endodermal urogenital sinus, with the innervating neurons of ectodermal origin [60, 61]. Therefore, ESCs are inherently capable to regenerate all tissues important to urothelial tract. Several studies have shown their ability to do so using both animal and human ESCs [62–64]. A recent report demonstrated high efficiency differentiation of human ESCs into urothelium without the need of culturing the cells in the presence of other cells or on urothelial inducing matrices [62]. These cells hold promise for TEUDs; however, they have not been sufficiently evaluated for this purpose. In addition to the well-known ethical controversies surrounding human ESCs, they have been shown to form teratomas in vivo [65–67]. Additionally, as with any allogenic stem cell, once differentiated they begin expressing their allogenic MHCs and can induce a rejection response, although studies suggest ESCs generate less of a response [68–70].
Circumventing the issues of ethics and rejection, iPSCs use cellular reprogramming to dedifferentiate an individual’s adult cell into a pluripotent stem cell [71]. Urothelial differentiation from iPSCs has been demonstrated [62, 72, 73]. Similar to ESCs, these differentiated cells have not been evaluated for TEUD applications. iPSCs also harbor tumorigenicity, in part due to the genes, which are often manipulated to generate iPSCs (c-Myc, Oct4, and Sox2) and are shared with various malignancies [74]. Advancements in iPSC technology, such as no longer requiring DNA manipulation for their induction, have reduced their tumor-generating potential [74].
The majority of stem cell-based tissue engineering studies have used adult stem cell populations. They have the advantages over ESCs and iPSCs of being more easily obtained, avoid ethical concerns, do not elicit rejection (when used autologously), and do not form tumors. These benefits come at the cost of decreased proliferative and differentiation potential. MSCs have been the workhorse stem cell of tissue engineering applications including their use for TEUDs [36]. First discovered in the bone marrow over 40 years ago, analogous cell populations have since been isolated from many different sources including muscle, dermis, trabecular bone, adipose tissue, periosteum, pericyte, blood, synovial membrane, and amniotic fluid [75, 76]. Although similar to bone marrow-derived MSCs (BM-MSCs), these populations can have unique characteristics including CD markers, gene expression profiles, and differentiation propensities toward specific tissue types and are often given unique terminology. MSCs have been shown to have many characteristics; however, scientific consensus identifies them as adherent, fibroblast-like cells, which express CD105, CD73, and CD90 surface proteins and do not express hematopoietic surface markers CD45, CD14, CD11b, CD79α, and HLA-DR [77]. They can differentiate into myogenic, adipogenic, osteogenic, chondrogenic, neurogenic, and urothelial lineages in vitro when cultured under specific conditions [78, 79]. Allogenic human umbilical cord MSCs have been used in clinical trials for treatment of Sjogren’s syndrome for their immune-modulating properties with promising results [80]. In TEUD applications where allogenic MSCs are expected to form differentiated tissues and persist, it is unclear if rejection to the MSCs will develop.
Adipose Stem Cell
Although initially believed to be equivocal to BM-MSCs, adipose stem cells (ASCs) have since been recognized as a unique stem cell population [79, 81, 82]. They have a similar differentiation profile as BM-MSCs and are easily expandable in culture [83]. ASCs are more easily obtained than BM-MSCs given the availability of adipose tissue and their relative abundance. They represent up to 3% of adherent cells in adipose tissue aspirates, whereas BM-MSCs represent less than 0.001% of adherent cells from bone marrow aspirates [17, 79, 81] (Fig. 7.3). Several studies have demonstrated ASCs ability to differentiate into UCs and SMCs with lineage-specific culturing conditions [17, 84, 85]. Human ASCs were differentiated into SMCs in vitro and seeded onto a poly-lactic-glycolic acid scaffold, which was used as a bladder augment following removal of half of the bladder in nude rats [17]. The seeded grafts maintained pre-cystectomy capacity, and muscle strips isolated 12 weeks following implantation contracted during tissue bath stimulation.


Fig. 7.3
Schematic demonstrating the steps involved in adipose-derived stem cell collection for scaffold seeding purposes. Subcutaneous fat is harvested by incisional biopsy or liposuction. The stromal vascular fraction (SVF) is isolated by gentle digestion, and cells are allowed to adhere to culture dishes where they are expanded and seeded onto scaffolds
Urine-Derived Stem Cells
Recently, stem cells with significant proliferative potential (60–70 population doublings) were identified in voided urine from 17 healthy volunteers aged 5–75 years [86]. A similar cell population was isolated from upper tract urine obtained from patients undergoing pyeloplasty [87]. In both studies, urine-derived stem cells (USCs) express MSC markers (CD44, CD73, CD105, CD133, STRO-1, and SSEA-4) as well as pericyte markers (CD146, NG2 proteoglycan, platelet-derived growth factor receptor beta) [86, 87]. Both cell populations were inducible to become differentiated UCs and SMCs and were able to be grown on collagen scaffolds and a urinary conduit [48, 87]. The ease of obtainment and autologous source makes them a good choice for tissue engineering applications; however, their urothelial source precludes their use for TEUDs in the setting of cancer.
Additional stem cell populations recently investigated for urinary tissue reconstruction include endometrial stem cells, amniotic fluid stem cells, and hair stem cells [23, 88–92]. Similar to prior studies using different cell types, scaffolds seeded with stem cells isolated from the follicular bulge (CD34, p63, and Ki-67 positive) demonstrated UC and SMC differentiation and improved tissue recellularization than acellular grafts alone [91]. Although promising, additional studies are needed to evaluate their use for TEUDs.
Growth Factors
Several growth factor stimuli serve as a source for the regenerative potential of an endogenous tissue-engineered organ. These stimuli include the microenvironment, endogenous production from cells seeded on the biomaterial, or ex vivo gene modification to secrete and overexpress growth factors that influence the milieu necessary for regeneration. An extracellular microenvironment is crucial for cell growth and development [57, 93]. Vascular endothelial growth factor (VEGF) has crucial roles in the formation of blood vessel networks [94]. Prior studies have examined the optimal VEGF concentration and found that those treated with 2 ng/g of tissue of VEGF have superior microvascular density and experience the most profound smooth muscle cell proliferation of the bladder in a porcine model [95].
Insulin-like growth factor-1 (IGF-1) is known to play a major role in development and cell proliferation. Lorentz et al. evaluated the effect of sustained IGF-1 delivery to a bladder lesion with recombinant IGF-1 [96]. The IGF-1 treatment group had twofold increased proliferation of SMCs compared to the control group. Accordingly, IGF-1 can potentiate SMC proliferation in tissue engineering with adult somatic cells.
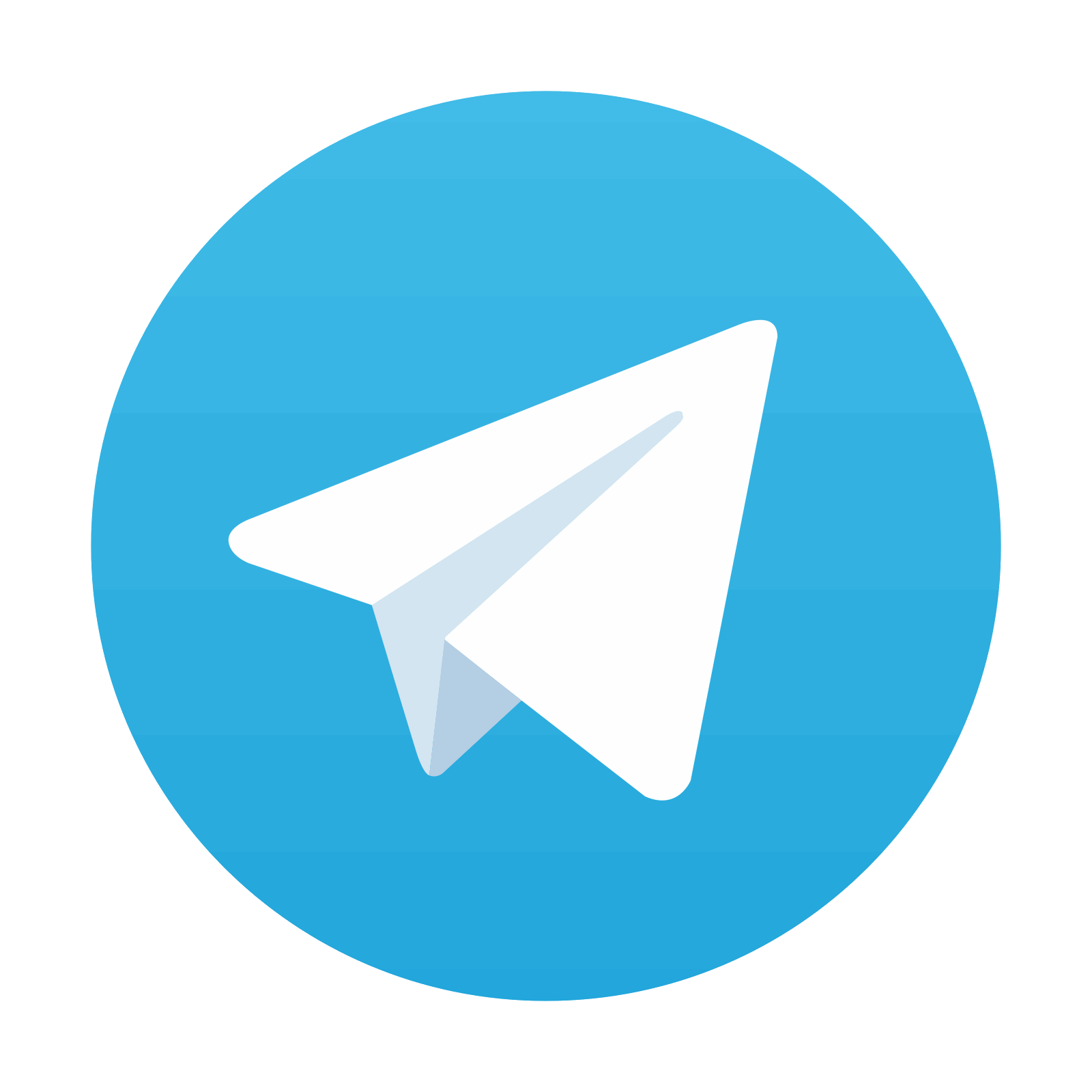
Stay updated, free articles. Join our Telegram channel

Full access? Get Clinical Tree
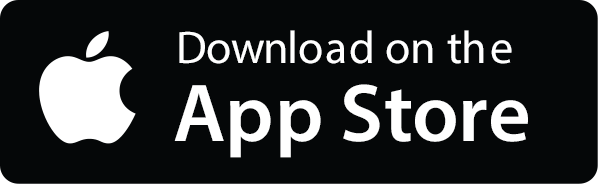
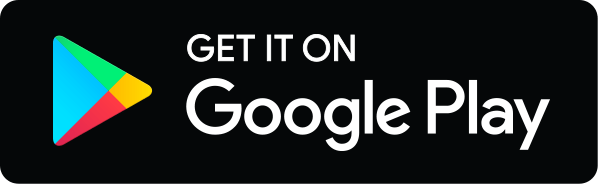