46 Daniel A. Wollin & Glenn M. Preminger Division of Urology, Department of Surgery, Duke University Medical Center, Durham, NC, USA With endoscopic technology allowing excellent access to urinary stones, ureteroscopic treatment of nephrolithiasis has become a primary option in many cases. According to the 2016 American Urological Association and Endourological Society Guidelines for Surgical Management of Stones, ureteroscopic treatment is first‐line for all ureteral calculi, as well as for intrarenal stones less than 20 mm [1, 2]. While smaller stones can be extracted intact, urinary calculi frequently require fragmentation prior to removal. As flexible and semirigid ureteroscopic technology provides better access to stones, adjunctive tools, including stone manipulation devices and lithotripters, have similarly become more advanced. In addition to efficiency and safety of intracorporeal lithotrites, newer fragmentation devices require reduced size and increased flexibility to allow for use with third‐generation ureteroscopes. Given the wide array of intracorporeal lithotrites, the endourologist must be familiar with each device, including mechanism of action, to appropriately choose the treatment for stones of various sizes, compositions, and locations (Figure 46.1). In this chapter, techniques for ureteroscopic lithotripsy will be discussed, with focus on the differences between each method, including their advantages and disadvantages in various clinical situations. Figure 46.1 Mechanisms of lithotripsy. (a) Electrohydraulic lithotripsy, (b) ultrasonic, (c) pneumatic/ballistic, (d) laser. Electrohydraulic lithotripsy (EHL) was the first method for intracorporeal lithotripsy utilized in clinical applications. The technology, first invented by Yutkin in 1955 at the University of Kiev, was used for upper tract stones during an open nephrolithotomy in 1975 [3, 4]. Since that time, EHL probes have been miniaturized for use in rigid and flexible ureteroscopes for treatment of ureteral stones [5, 6]. Despite its effectiveness and low cost, it is rarely used because of the high risk of ureteral injury. Yet, its low cost still makes EHL an option in certain situations. The mechanism for EHL fragmentation involves discharge of electrical energy in a liquid medium. The probe is composed of two electrodes of different polarities; a current passes between the electrodes and the energy release forms a plasma channel and vaporization of nearby water. This vaporization creates a shock wave and cavitation bubble, whose collapse creates secondary shock waves or high‐speed microjets, all of which cause stone fragmentation. The advantage of EHL is the low cost of components. The EHL generator and probes are currently less expensive than other intracorporeal lithotripters, with one or two probes used per procedure depending on stone composition [7, 8]. Additionally, modern EHL probes are small and flexible, allowing for treatment of stones through all types of ureteroscopes. The main disadvantage of EHL is high risk of intraoperative injury. Initial experience of EHL reported high complication rates, as the lithotripsy was performed with large probes (9 Fr) and without endoscopic guidance [4, 9]. With improvement in endoscopic visualization, decrease in probe size (to 1.6 Fr), and improved control of energy output, EHL has become safer, although the complication rate remains high, between 6 and 17.6% [6, 10–12]. Complications of EHL range from ureteral perforation and hemorrhage to mucosal erosion, inflammation, and ureteral stricture. Studies have been performed to understand the mechanisms by which EHL causes ureteral injury. In addition to direct injury to the ureteral wall from firing of the probe onto mucosa, expansion of the cavitation bubble may induce a burst effect on the ureter [11]. With energy of more than 1300 mJ, the cavitation bubble can reach 1.5 cm in size; thus, decreasing the energy can reduce capacity for injury. One in vitro study demonstrated that 24 pulses to the ureteral wall were sufficient for perforation from 0.5 mm, highlighting the care needed while using EHL [13]. The stone fragmentation and clearance rates are not significantly higher for EHL than for newer lithotripters. In addition to fragment retropulsion, which appears more common than with other methods, EHL produces more and larger fragments, reducing stone clearance [14]. EHL is less effective with harder and larger stones (>1.5 cm) and the stone‐free rates range widely from 67 to 98% [7]. Given its risks, EHL should be performed under careful attention and with the lowest power settings that can produce fragmentation. Smaller probes (1.6–1.9 Fr) should be used to decrease perforation risk and to improve irrigation. Prior to use of the lithotripter, insulation layers of the probe should be examined to ensure they are intact. The distal tip of the probe should be placed 2–5 mm from the end of the ureteroscope to reduce damage to the lens, while the probe should be kept 1 mm from the stone to improve shock‐wave interaction [15]. Visualization of the stone must be maintained, along with adequate irrigation to distend the ureter away from the probe, to reduce risk of injury. Lithotripsy should begin with low voltage (50–60 V) and single pulses with a slow increase in generator output until the fragments are small enough for basket removal. After 60 seconds of firing, the probe should be examined for loss of insulation, as a new probe may be required. Care must be taken to avoid firing upon any safety wire or retrieval device, as these are damaged by EHL energy. Similar to EHL, ballistic and pneumatic lithotripsy is an older method of fragmentation. These lithotripters come in many sizes and types of energy production and all necessitate a straight probe during use. The rigid probes decrease the practicality of this technology for upper tract stones requiring flexible ureteroscopy. Still, ballistic and pneumatic lithotripsy remains safe and effective when used in the proper setting. Ballistic and pneumatic lithotrites pass energy from a projectile to the probe (0.8–3.0 mm) and then directly to the stone with a “jackhammer” effect. When fired repeatedly (on the order of 12 Hz with automatic lithotripters), this production of 2–30 atm at the stone surface causes cracking and fragmentation. Production of this ballistic energy varies between lithotripters, with use of large‐scale compressed air (Lithoclast, Electromedical Systems/Boston Scientific), handheld high‐pressure CO2 cartridges (StoneBreaker, LMA Urology/Cook Medical), or electromagnetic acceleration of a magnetic core (LithoBreaker electrokinetic probe, Electromedical Systems). In each case, the probe is held in contact with the stone to transmit the ballistic energy. This method requires energy transfer through a rigid probe; bowing of the device reduces power transmission, limiting the utility of this method to unflexed endoscopes. Ballistic lithotripsy is a low‐cost option. Disposable costs are minimal, as probes have a long life span, unlike laser and EHL [10]. While free‐standing pneumatic lithotripters require more expensive consoles compared to EHL, there is minimal maintenance and no disposable costs. The handheld pneumatic lithotripter is less expensive and the disposable CO2 cartridges, which deliver approximately 80 shocks each, add minimal cost to the device. Ballistic and pneumatic lithotripsy, utilizing direct mechanical energy transfer, are safe compared to other technologies. In contrast to EHL, ultrasonic lithotripsy, and holmium laser, ballistic lithotripsy causes less injury and has lower risk of ureteral perforation [16]. After 6 minutes of direct application of pneumatic lithotripsy to a ureteral wall in vitro, no perforation was seen [13]. Additionally, thermal injury is unlikely as minimal heat is produced. Compared to the relatively slow comminution of laser fragmentation (which is discussed later), ballistic lithotripsy has a more rapid stone clearance time [17, 18]. Despite its safety and low cost, ballistic lithotripsy has slightly lower success than other methods, likely secondary to its inability to fragment stones when torqued and the high risk of stone retropulsion. Stone‐free rates after ballistic or pneumatic lithotripsy range from 70 to >90%, although the lower rates occur in settings of probe deflection and retropulsion [19–21]. Through a flexible ureteroscope, deflection of more than 24° will significantly reduce energy transmission; distal semirigid ureteroscopy allows for decreased need for flexion and decreased power loss [22]. Thus ballistic lithotripsy is very effective through a semirigid ureteroscope for mid/distal ureteral stones. The other disadvantage of ballistic lithotripsy is its retropulsion rate, which has been reported in 2–17% of ureteroscopic treatments. Due to this high rate of retropulsion, especially given the rigid lithotripter, migration into the proximal ureter or kidney can render stone fragments untreatable with this method alone. Many tools have been developed to prevent stone migration, including stone‐trapping devices (such as stone baskets, gel polymeric plugs, and ureteral balloons) and suction devices to be used alongside the lithotripter [23, 24]. These methods reduce the risk of retropulsion and improve fragmentation efficiency and stone‐free rates, although careful use of the lithotripter may preclude the need for such techniques [25, 26]. As with all lithotripters, endoscopic visualization of the stone is a prerequisite for treatment. The stone should be pinned in place for fragmentation and to decrease retropulsion, whether held against urothelium or using a trapping mechanism. Bending of the endoscope or probe should be avoided in order to maintain power during lithotripsy. The probe must be in contact with the stone and it is recommended to work from the periphery to separate fragments small enough for spontaneous passage. Given the minimal flexibility of this device, if larger fragments migrate proximally, chasing and treating such pieces becomes problematic. Ultrasonic lithotripsy was described by Mulvaney in 1953, with the first ureteral applications in the 1970s and 1980s [27, 28]. This method uses ultrasonic vibrations to fragment calculi; many ultrasonic lithotripter probes include a suction channel to allow simultaneous fragment removal. Similar to ballistic lithotripsy, this requires a rigid device, reducing the utility of ultrasonic lithotripsy during ureteroscopy. Smaller probes (2.5 and 4.5 Fr), with or without suction capabilities, can be used through a semirigid ureteroscope with success and safety. The ultrasonic method of lithotripsy utilizes an electrically charged piezoceramic plate within the transducer that resonates at high frequency (23 000–25 000 Hz) and generates ultrasonic waves. These longitudinal and transverse waves are passed along a hollow steel probe, which makes contact with and fragments the stone. Fragmentation occurs as noncompliant stone material is vibrated at a high frequency such that the stone breaks; compliant tissue (e.g. urothelium) does not resonate in this manner and inadvertent damage is minimal [3, 29]. Due to vibration of the ultrasonic probe, heat development is a concern, but most probes allow for concurrent suction through their core, which enables irrigation flow and heat dispersion. With adequate irrigation/suction and intermittent probe firing, temperature increase can be minimized [30]. The probes are reusable but will incur damage: periodic replacement is required. Of note, combination lithotripters exist that allow for ultrasonic and ballistic fragmentation (Lithoclast Ultra/Select, Electromedical Systems/Boston Scientific) or dual‐probe ultrasonic lithotripsy (CyberWand, Gyrus ACMI/Olympus). These technologies allow for improved fragmentation and suction, but are only available in the percutaneous setting given their size and rigidity [31, 32]. The advantages of ultrasonic lithotripsy are rapid fragmentation along with concomitant fragment removal. Studies report stone‐free rates from 88 to 98%, making this an effective method [33–35]. These success rates are seen throughout the literature using ureteroscopic probes of 4.5 Fr (hollow probe with suction) or 2.5 Fr (solid probe without suction). Still, the capability for simultaneous removal of small fragments during lithotripsy is beneficial. This suction ability is particularly useful in a setting of steinstrasse, as the stone debris can be continuously evacuated. An advantage discussed previously is the relative safety of the ultrasonic mechanism. Due to the method of vibrational energy transmission, flexible material – such as ureteral tissue – is not damaged easily. In vitro, the ultrasonic device has the longest average time to urothelial perforation, with injury occurring at 118 seconds of continuous firing in direct wall contact [29]. Probe temperature rise is a concern, but is managed with adequate irrigation. Despite these advantages, this method is only utilized in semirigid ureteroscopy. If suction is necessary, a ureteroscope with a large working channel is required for passage of the 4.5 Fr probe. While the 2.5 Fr solid probe is smaller, semirigid ureteroscopy is still required for use of this device. Given its inability to be used with a flexible ureteroscope, this technology is typically restricted to percutaneous surgery, where rigid nephroscopes allow passage of larger and more powerful ultrasonic probes. Endoscopes with minimal flexion and large working channel are required for rigid probe use. Gentle pressure against the stone is required, although suction capabilities or simultaneous use of a stone‐trapping device can reduce the need for pressure and decrease the risk of urothelial injury. With larger ureteroscopic probes, suction allows for concurrent removal of small stone fragments during lithotripsy. Fragmentation is most efficient when performed along the stone periphery to produce smaller pieces that are suctioned away. To reduce heat production, continuous high‐flow irrigation is recommended along with intermittent probe firing. Nanosecond electropulse lithotripsy (NEPL) is the newest method of intracorporeal lithotripsy, described in 2007 [36]. It utilizes similar technology to EHL, although due to different characteristics of energy discharge it has a better safety profile. Early data show similar ease of access and flexibility compared to laser/EHL, along with excellent fragmentation, making this a promising new method for lithotripsy. While EHL and NEPL probes contain two distal electrodes across which current is applied, the NEPL probe differs due to the voltage and time over which energy is released. In contrast to EHL discharge time of hundreds of microseconds at low voltages, NEPL releases higher voltages in less than 50 nanoseconds. This method of energy release, when the probe is touching a non‐conducting material such as urinary calculi, produces spontaneous conduction and direct electrical breakdown of the target. In the absence of a solid target, the probe performs similarly to EHL and releases energy into the surrounding fluid, creating a shock wave and cavitation bubble. The focus of electrical energy into the target (instead of the surrounding fluid) is what provides the proposed safety of NEPL. NEPL has become the focus of recent studies given the potential for improvement over previous technology. In vitro studies have shown improved energy and time efficiency compared to EHL and laser lithotripsy [37–39]. Stone‐free rates are comparable to other methods, with 91–100% success depending on the stone location (mid ureter with better success than upper ureter) [36]
Ureteroscopy Energy Sources
Introduction
Electrohydraulic lithotripsy
Overview
Technology
Advantages/disadvantages
Technical tips
Ballistic/pneumatic lithotripsy
Overview
Technology
Advantages/disadvantages
Technical tips
Ultrasonic lithotripsy
Overview
Technology
Advantages/disadvantages
Technical tips
Nanosecond electropulse lithotripsy
Overview
Technology
Advantages/disadvantages
Stay updated, free articles. Join our Telegram channel

Full access? Get Clinical Tree
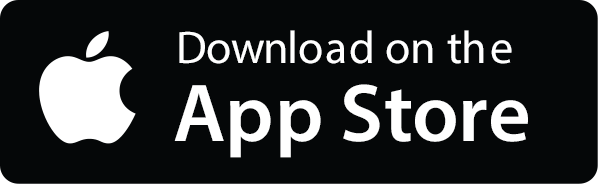
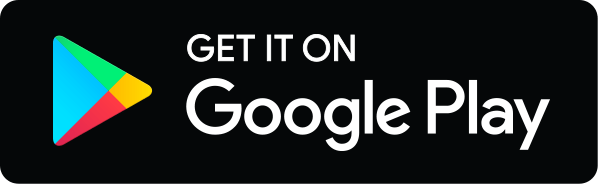