Janus kinase (JAK) inhibitors have emerged as a novel orally administered small-molecule therapy for the treatment of ulcerative colitis and possibly Crohn disease. These molecules are designed to selectively target the activity of specific JAKs and to offer a targeted mechanism of action without risk of immunogenicity. Based on data from clinical trials in rheumatoid arthritis and phase 2 studies in inflammatory bowel disease, tofacitinib and other JAK inhibitors are likely to become a new form of medical therapy for the treatment of inflammatory bowel disease.
Key points
- •
Janus kinase (JAK) inhibitors represent a novel small-molecule therapy that will likely become a new medical treatment for inflammatory bowel disease (IBD).
- •
JAK inhibitors target small intracellular molecules responsible for transducing signals from inflammatory cytokines believed to be involved in the pathogenesis of IBD.
- •
Based on phase 2 clinical trials, tofacitinib, a JAK1 and JAK3 inhibitor, has a dose-dependent effect in ulcerative colitis and potentially an effect in Crohn disease.
- •
Tofacitinib is associated with a potential risk of opportunistic infections, lipid abnormalities, bone marrow suppression, and lymphoma; however, the safety profile appears similar to that of the current therapies for IBD.
- •
Multiple JAK inhibitors with different specificities and side-effect profiles are being studied in the treatment of IBD.
Introduction
The current treatment options for inflammatory bowel disease (IBD) include aminosalicylates, immunosuppressives, corticosteroids, and monoclonal antibodies to tumor necrosis factor (TNF)-α. Progress in understanding the pathogenesis of IBD, together with innovations in technology, have led to the introduction of new monoclonal antibodies directed at other inflammatory cytokines and leukocyte trafficking molecules. Most recently, small-molecule inhibitors have emerged as an appealing therapy, given their potential for oral administration, lack of immunogenicity, and less interpatient pharmacokinetic variability in comparison with monoclonal antibodies.
Janus kinase (JAK) inhibitors have emerged as a new small-molecule therapy for autoimmune disease. These drugs simultaneously target multiple cytokine signaling pathways known to be involved in the pathogenesis of inflammatory and autoimmune diseases. Tofacitinib, a JAK inhibitor targeting JAK1 and JAK3, was recently approved for the treatment of rheumatoid arthritis (RA), and is currently under evaluation for the treatment of both ulcerative colitis (UC) and Crohn disease (CD). With the continued development of drugs that can specifically target JAK proteins individually and in combination, JAK inhibitors, termed JAKINIBs, are an increasingly appealing therapy for autoimmune diseases, allowing clinicians the ability to tailor drug specificity to optimize the balance between desired and adverse effects.
Introduction
The current treatment options for inflammatory bowel disease (IBD) include aminosalicylates, immunosuppressives, corticosteroids, and monoclonal antibodies to tumor necrosis factor (TNF)-α. Progress in understanding the pathogenesis of IBD, together with innovations in technology, have led to the introduction of new monoclonal antibodies directed at other inflammatory cytokines and leukocyte trafficking molecules. Most recently, small-molecule inhibitors have emerged as an appealing therapy, given their potential for oral administration, lack of immunogenicity, and less interpatient pharmacokinetic variability in comparison with monoclonal antibodies.
Janus kinase (JAK) inhibitors have emerged as a new small-molecule therapy for autoimmune disease. These drugs simultaneously target multiple cytokine signaling pathways known to be involved in the pathogenesis of inflammatory and autoimmune diseases. Tofacitinib, a JAK inhibitor targeting JAK1 and JAK3, was recently approved for the treatment of rheumatoid arthritis (RA), and is currently under evaluation for the treatment of both ulcerative colitis (UC) and Crohn disease (CD). With the continued development of drugs that can specifically target JAK proteins individually and in combination, JAK inhibitors, termed JAKINIBs, are an increasingly appealing therapy for autoimmune diseases, allowing clinicians the ability to tailor drug specificity to optimize the balance between desired and adverse effects.
Janus kinase family
The JAK proteins are a family of nonreceptor tyrosine kinases that possess a highly conserved kinase domain responsible for its enzymatic activity. The family consists of JAK1, JAK2, JAK3, and tyrosine kinase 2 (TYK2); these proteins associate with the intracellular portion of cytokine or hormone receptors. The family has been shown to play a central role in the signal transduction pathways for multiple cytokines, including proinflammatory cytokines involved in the pathogenesis of autoimmune diseases.
Type I and Type II Cytokine Receptors
The JAK family mediates signals from transmembrane type I and type II cytokine receptors that are expressed on cells capable of responding to cytokines. Type I cytokine receptors have a conserved structure defined by an extracellular WSXWS amino acid motif and intracellular domain through which receptors selectively associate with JAKs. Many receptors possess common subunits, such as the γ-chain (CD132), β-chain (or CD131), and glycoprotein 130 (gp130 or CD130). Receptors with a common γ-chain bind to interleukin (IL)-2, IL-7, IL-9, IL-15, and IL-4, and signal through JAK1 and JAK3. The β-chain–containing receptors bind to granulocyte macrophage colony stimulating factor (GM-CSF), IL-3, and IL-5, and signal through JAK2. Receptors containing gp130 bind to IL-6, IL-11, and IL-12, and signal primarily through JAK1 with the exception of IL-12, which uses TYK2. Other type I receptors are homodimer receptors for hormones, such as growth hormone, prolactin, erythropoietin, thrombopoietin, and GM-CSF, and signal through JAK2.
Type II cytokine receptors are a similar family of receptors that do not possess the amino acid WSXWS motif. Type II receptors bind to cytokines such as interferon (IFN)-α, IFN-β, IFN-γ, IL-10 family cytokines, and IL-20, and primarily signal through JAK1, with the exception of IFN-γ, which uses both JAK1 and JAK2. Together, type I and type II receptors associate with JAK family proteins to mediate the effects of cytokines or hormones ( Table 1 ).
Type | Cytokine Common Chain | Cytokines | JAK Signaling Molecules | STAT Signaling Molecules |
---|---|---|---|---|
Type I | γ-Chain | IL-2, IL-7, IL-9, IL-15 | JAK1, JAK3 | STAT5 |
IL-4 | JAK1, JAK3 | STAT6 | ||
β-Chain | GM-CSF, IL-3, IL-5 | JAK2 | STAT5 | |
gp130 | IL-6, IL-11 | JAK1, JAK2, TYK2 | STAT3 | |
IL-12 | JAK2, TYK2 | STAT4 | ||
Other | GH, Epo, PRL, TPO | JAK2 | STAT5 | |
Type II | IFN-α, IFN-β | JAK1, TYK2 | STAT1, STAT2 | |
IFN-γ | JAK1, JAK2 | STAT1 | ||
IL-10 | JAK1, TYK2 | STAT3 |
Mechanism of JAK Signaling
Although JAK proteins are associated with distinct cytokine and hormone receptors, common intracellular signaling pathways are used to initiate transcriptional changes. On cytokine binding, cytokine receptor subunits form multimers, bringing the conserved intracellular tails of the subunits with associated TYKs in close proximity to one another, and JAKs phosphorylate tyrosine residues on the cytokine receptor. Phosphorylation of the cytokine receptor tail creates a docking site for transcription factors called signaling transducers and activators of transcription (STATs). STATs are a family of transcription factors that initiate the transcription of genes that mediate the effects of cytokine signaling. STATs are recruited to the cytokine receptor complex, and JAKs phosphorylate a tyrosine residue in the SRC homology 2 (SH2) domain of the STAT molecule. This process leads to STAT dimerization, translocation to the nucleus, and transcriptional activation of target genes downstream from cytokine signaling. STAT family members selectively associate with JAK proteins in different combinations to initiate the unique transcriptional changes induced by a specific cytokine or hormone ( Table 2 ).
Receptor | Associated JAK Family Member | |||
---|---|---|---|---|
JAK1 | JAK2 | JAK3 | TYK2 | |
IL-2 Receptor | + | − | + | − |
IFN-γ Receptor | + | + | − | − |
IFN-α Receptor | + | − | − | + |
IL-12 Receptor | − | + | − | + |
IL-23 Receptor | − | + | − | + |
IL-6 Receptor | + | + | − | + |
Epo Receptor | − | + | − | − |
JAK Deficiency
Although the JAK family uses overlapping signaling pathways, each JAK has a unique role in modulating the immune system. Mice or humans completely deficient in a specific gene serve as important models for defining the function of each JAK family member. For example, complete deficiency of JAK1 or JAK2 is lethal. JAK1 is expressed not only in immune cells but also in precursors of the nervous system. As a consequence, deficiency of JAK1 leads to significant neurologic deficits and lymphoid developmental abnormalities with a perinatal lethal phenotype. By contrast, mice lacking JAK2 have defective erythropoiesis and consequently die during embryogenesis. Given the lethality of these mutations, complete deficiencies of JAK1 or JAK2 have not been described in humans; however, activating, gain-of-function mutations in JAK2 have been described in polycythemia vera and myeloproliferative disorders.
Lack of TYK2 does not seem to be lethal, although only 2 documented cases of TYK2 deficiency have been described in humans with similar phenotypes. Both patients appeared to have increased susceptibility to bacterial and viral infections that were most likely related to deficient IFN and IL-12 signaling, which are mediated by TYK2. However, only 1 patient had elevated immunoglobulin E concentration with clinical features of atopy. Based on mouse models, TYK2 appears to be play a central role only in IL-12 and IFN signaling but also in the production of IL-12 and IL-23 by dendritic cells involved in directing the adaptive immune response.
Deficiency of JAK3, which is primarily expressed in immune cells, has been described extensively in humans, causing severe combined immune deficiency as a result of absent γ-chain cytokine receptor signaling. The phenotype of humans lacking either γ-chain cytokine receptors or JAK3 is nearly identical: profound T-lymphocyte and natural killer (NK) deficits leading to life-threatening susceptibility to infections. The immune deficits are a result of impaired T-lymphocyte development and proliferation. Although B-lymphocyte numbers are preserved, significant functional deficits exist in antibody production and class switching. Lymphocyte abnormalities appear to be driven by lack of JAK3-dependent IL-7 signaling that under normal conditions drives development of T and B lymphocytes. Similarly, IL-15 signaling through JAK3 serves as a survival signal for NK cells. Furthermore, JAK3 signaling also drives CD4 + T-cell differentiation into specialized type 1 helper T (Th1) and type 2 helper T (Th2) cells. IL-4 signaling mediated by JAK3 drives the differentiation of Th2 cells, and JAK3-dependent alterations in the IFN-γ promoter lead to Th1 differentiation. JAK3, perhaps more so than its JAK family counterparts, appears to play a critical, nonredundant role in driving lymphocyte development, proliferation, and differentiation.
Genome-Wide Association Studies and JAK Signaling
JAK-STAT signaling is known to be critical in regulating and modulating the immune response based on numerous in vivo and in vitro models. Moreover, genome-wide association studies have identified single-nucleotide polymorphisms (SNPs) in the genome that are associated with increased risk of disease and provide a definitive link between the JAK-STAT pathway and human autoimmunity. SNPs associated with an increased risk of both UC and CD have been identified throughout the JAK-STAT cytokine signaling pathway, including cytokines (eg, IL-12β), cytokine receptors (eg, IL-23R), JAKs (eg, JAK2), and downstream STAT proteins (eg, STAT3). Furthermore, studies of IBD patients revealed excess production of cytokines initiating JAK-STAT signaling, such as IL-1β, IL-6, IL-12, and IL-23. Together with the animal studies, these genetic studies underscore the importance of JAK-STAT signaling in the immune system, identifying this pathway as a potential therapeutic target.
Tofacitinib: JAK inhibitor
Knowledge of the JAK-STAT signaling pathways has been applied to the development of orally administered small-molecule inhibitors, which are being tested in clinical trials for the treatment of autoimmune diseases. Tofacitinib (CP-690550) was the first small-molecule JAK inhibitor tested in clinical trials for the treatment of autoimmune diseases, such as psoriasis, RA, prevention of allograft rejection, and IBD. Tofacitinib interferes with the JAK-STAT signaling by competing with adenosine triphosphate for binding to the kinase domain of JAKs and inhibits JAK1, JAK2, and JAK3. In vitro studies, however, showed preferential inhibition of JAK1 and JAK3 with less effect on JAK2 ( Fig. 1 ).
Preclinical mechanistic studies of tofacitinib showed a reduction in production of inflammatory cytokines and differentiation into cell lineages associated with autoimmunity. In vitro studies confirmed that tofacitinib disrupted signaling downstream of JAK3-dependent γ-chain cytokine receptors, including signals dependent on IL-2, IL-4, IL-7, IL-15, and IL-21. Treatment with tofacitinib reduced JAK1- and JAK2-dependent signaling by IL-6, IFN-γ, and IL-12. Tofacitinib also inhibited differentiation of naïve murine CD4 + T cells into Th1, Th2, and Th17 cells, subsets that have been implicated in autoimmunity and the pathogenesis of IBD. In addition, tofacitinib disrupted lipopolysaccharide signaling, an important activator of the innate immune system. In these mechanistic studies, tofacitinib had significant effects on dampening both the adaptive and innate immune responses that appear to be overactive in IBD and autoimmunity.
Tofacitinib in Autoimmune Diseases
Based on the immune modulation seen in mechanistic studies, tofacitinib has been studied in the treatment of numerous autoimmune diseases. The greatest progress has been in the treatment of RA, for which phase 3 clinical trials demonstrated the effectiveness of tofacitinib in improving clinical scores and physical function of patients with RA. The trials have been consistent in demonstrating clinical efficacy as monotherapy in patients with inadequate response to biological or nonbiological disease-modifying antirheumatic drugs (DMARDs). Subsequently, another study showed that the combination of tofacitinib with methotrexate was not inferior to adalimumab and methotrexate, the standard of care, for the treatment of active RA. The Food and Drug Administration (FDA) has approved tofacitinib for use in RA at a dose of 5 mg twice daily. A dose of 10 mg twice daily was not approved by the FDA, and neither the 5-mg nor the 10-mg doses were approved by the European Medicines Agency, pending requirements for additional safety information.
Ulcerative Colitis and Tofacitinib
A recent phase 2 randomized controlled trial of tofacitinib demonstrated efficacy in patients with moderately to severely active UC ( NCT00787202 ). The study enrolled 194 patients with moderately to severely active UC with a baseline Mayo Clinic disease activity score of 8 who were randomized to tofacitinib 0.5 mg, 3 mg, 10 mg, 15 mg, or placebo twice daily. Tofacitinib was administered for 8 weeks twice daily without concomitant immune modulators or biologics. The primary end point was clinical response at 8 weeks, as defined by a decrease in the baseline Mayo score of 3 or more, a 30% reduction from baseline in the Mayo score, and at least a 1-point reduction in the rectal bleeding subscore or absolute rectal bleeding subscore of 0 or 1. There was a dose-dependent effect, with clinical response observed in 32%, 48%, 61%, and 78% of patients treated with tofacitinib 0.5 mg, 3 mg, 10 mg, and 15 mg, respectively, compared with 42% of patients on placebo. Clinical remission at 8 weeks, as defined by Mayo score of 2 or lower without any subscore greater than 1, was observed in 13%, 33%, 48%, 41% of patients treated with tofacitinib 0.5 mg, 3 mg, 10 mg, and 15 mg, respectively, compared with 10% on placebo. Overall, the study showed promising differences in clinical response and remission between tofacitinib and placebo.
The endoscopic end points measured in this phase 2 trial provide additional evidence of efficacy for tofacitinib in the treatment of UC. Specifically, endoscopic response at 8 weeks was also observed in a dose-dependent manner in 52%, 58%, 67%, and 78% of patients treated with tofacitinib 0.5 mg, 3 mg, 10 mg, and 15 mg, respectively, compared with 46% on placebo, with significant differences between the 15-mg dose and placebo ( P = .001). Endoscopic remission, a more stringent end point defined as Mayo subscore of 0 or 1, was achieved in 10%, 18%, 30%, and 27% of patients treated with tofacitinib 0.5 mg, 3 mg, 10 mg, and 15 mg, respectively, compared with 2% on placebo. The differences in the proportion of patients who achieved endoscopic remission in the 3 higher doses in comparison with placebo were significant. Overall, the dose-dependent endoscopic results provide additional support for the proposal that tofacitinib is effective for the treatment of moderately to severely active UC.
Additional support for the efficacy of tofacitinib in the treatment of UC comes from the reduction in inflammatory biomarker concentrations that occurred during treatment. Significant reductions in the log-transformed concentration of serum C-reactive protein (CRP) were seen in the 15-mg group when compared with the placebo arm ( P <.001), and reductions in the log-transformed concentration of fecal calprotectin were also observed in the 10-mg and 15-mg groups in comparison with the placebo arm ( P = .01, P <.001, respectively). The reductions in CRP and fecal calprotectin seen in patients treated with tofacitinib provide additional evidence for its efficacy in UC.
Overall, this phase 2 study provides promising data that tofacitinib at doses of 10 and 15 mg twice daily is effective in patients with moderately to severely active UC. Phase 3 studies are currently under way to evaluate the efficacy of tofacitinib 10 mg twice daily as induction therapy in patients with active UC, and 5 and 10 mg twice daily as maintenance therapy in patients with UC who respond to induction therapy with tofacitinib.
Crohn Disease and Tofacitinib
Results from a phase 2 randomized controlled trial of tofacitinib in CD ( NCT00615199 ) were also recently published. One hundred thirty-nine patients with moderate to severe CD with a baseline CD activity index (CDAI) of 220 to 450 were enrolled. The patients were not treatment-naïve but were prohibited from receiving concomitant immunomodulators or biologics. Patients were randomized to tofacitinib 1 mg, 5 mg, 15 mg, or placebo twice daily for a total of 4 weeks. The primary end point was clinical response at week 4 as defined by a reduction in CDAI score from baseline of 70 or more points, and the secondary clinical end point was clinical remission, defined by a CDAI score of less than 150.
Given the previously described results in UC, the results from this study in CD were surprising, in that the primary end point of clinical response was achieved in 36%, 58%, and 46% of patients in the 1-, 5-, and 15-mg tofacitinib arms, respectively, and were not significantly different from the 47% response rate in the placebo group. Clinical remission occurred in 31%, 24%, and 14% of the patients in the 1-, 5-, and 15-mg tofacitinib arms, respectively, compared with 21% in the placebo group. Similarly, the changes from baseline to week 4 in mean CDAI scores were similar in each of the groups. While there were no significant differences in clinical response or remission rates between tofacitinib and placebo, there were dose-related reductions from baseline to week 4 in the concentrations of CRP and fecal calprotectin, and the greatest changes were observed in the group receiving the highest dose (15 mg) of tofacitinib. Although the clinical responses did not show compelling dose-dependent responses, the reduction in the objective inflammatory biomarker concentrations suggested that tofacitinib might have a biological effect in CD.
The response and remission rates for CD at 4 weeks in the placebo arm were higher than anticipated for a short-duration 4-week study, and higher than those reported in comparable trials with biologics. High clinical response and remission rates in the placebo arm, together with the high rate of screening failure (41%), raises the possibility that patients may have been enrolled in the study based on symptoms that were not related to active CD. The primary inclusion criteria relied on CDAI scores, which do not always correlate with active inflammatory or endoscopic disease. Only 65% of the patients in the placebo arm had elevated CRP concentrations, and only 56% had elevated fecal calprotectin concentrations. These results were analogous to those from a 6-week induction trial of certolizumab pegol in which the primary clinical end points were not met, potentially because of high placebo response rates associated with a significant proportion of patients with a normal CRP concentration at baseline.
Although the clinical end points were not met, the improvement in objective biomarker concentrations in the tofacitinib 15-mg group supports the possibility that there was a biological effect in CD. With the knowledge gained from the first trial in CD, a phase 2b tofacitinib induction ( NCT01393626 ) trial in CD is ongoing. The study relies on confirmation of active CD with endoscopy or radiography before enrollment, with the goal of reducing high placebo response rates. However, it is also possible that differences in the pathogenesis between CD and UC account for the divergent clinical responses to tofacitinib, and perhaps a JAK inhibitor with different selectivity will be more efficacious in CD.
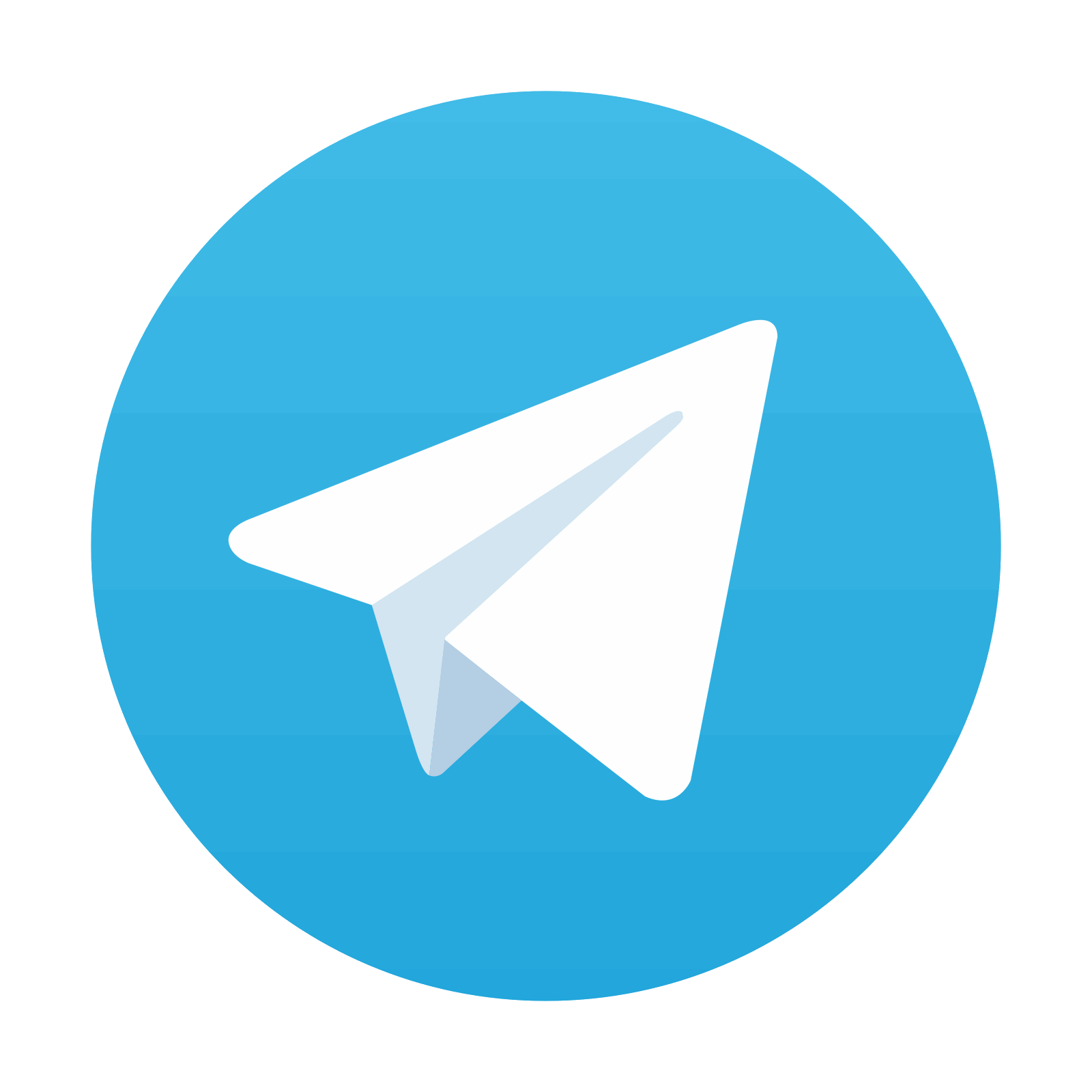
Stay updated, free articles. Join our Telegram channel

Full access? Get Clinical Tree
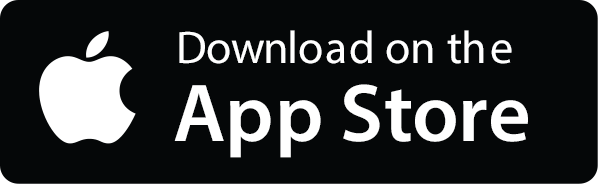
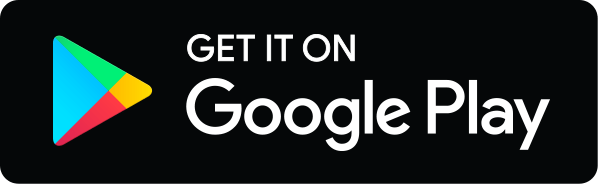