Fig. 3.1
Structure of the epithelial barrier . (a) Transmission electron micrograph depicting the apical junctional complex between two mouse enterocytes. From apical to basal, the apical junctional complex consists of the zonula occludens, also referred to as the tight junction (TJ); the zonula adherens, also referred to as the adherens junction (AJ); and macula adherens, also referred to as the desmosome. Microvilli (Mv) line the luminal surface of intestinal epithelia and are labeled for orientation. (b) The tight junction is spanned by occludin and claudin family members, which dynamically regulate permeability between cells. The plaque protein zonula ocludens-1 (ZO-1) interacts with transmembrane tight junction proteins and with the actomyosin cytoskeleton to regulate tight junction function. Myosin light chain kinase (MLCK) controls junction-associated myosin phosphorylation and myosin ATPase activity to regulate barrier function. (c) The cytosolic tight junction scaffolding protein ZO-1 is essential for cortical actin organization. Fluorescence microscopy (top two panels) and scanning electron microscopy (bottom two panels) of confluent epithelial monolayers show that ZO-1 deletion results in marked abnormalities of apical structure. Top panels are maximum intensity projections of confocal z-series through the entire epithelial monolayer. Scale bars = 5 μm
Molecular Anatomy of the Tight Junction
The adherens junction is formed by homotypic interactions between the epithelial cadherin isoform E-cadherin (Fig. 3.1B). The cytoplasmic domains of the cadherins interact with the catenins, which in turn bind to the actin cytoskeleton to regulate cell structure and function [33–35]. The tight junction consists of many transmembrane proteins, including occludin and claudins [36–39] (Fig. 3.1b). Transmembrane tight junction proteins are linked to one another and to the actomyosin cytoskeleton by the cytosolic scaffolding protein zonula occludens (ZO)-1, thereby forming an elaborate protein network at the apical junctional complex [40–43]. The extracellular loops of claudins mediate homotypic interactions to form high-capacity size- and charge-selective paracellular pores [44, 45]. In contrast, occludin , ZO-1, and the actomyosin cytoskeleton are known to regulate leak pathway permeability [46–49]. These molecular components interact and can regulate both pathways, as occludin and ZO-1, which primarily regulate the leak pathway, can also affect claudin pore function [50]. ZO-1 may affect paracellular permeability through regulation of cortical actomyosin organization and contractile activity [43, 51]. Moreover, by integrating cortical actomyosin function, ZO-1 directs a diverse array of cellular processes; ZO-1 depletion has marked effects on epithelial morphology (Fig. 3.1c).
In vitro and in vivo studies have revealed that tight junction assembly is closely linked to adherens junction integrity, as confluent epithelial monolayers lacking E-cadherin or alpha-catenin are unable to efficiently recruit tight junction proteins or form effective barriers to ions and macromolecular flux [52–55].
Intercellular Junctions and IBD
The critical role of the adherens junction in intestinal physiology was demonstrated using chimeric mice in which some, but not all, villous enterocytes expressed a dominant-negative cytoplasmic tail of N-cadherin [52]. Expression of the dominant-negative N-cadherin tail disrupted E-cadherin function and resulted in the loss of intercellular junctions, aberrant epithelial differentiation, Crohn’s-like disease, and epithelial dysplasia [52]. E-cadherin may also be relevant to human disease as data from recent genome-wide association studies (GWAS) suggest that polymorphisms within CDH1, the gene encoding E-cadherin, may be associated with ulcerative colitis [56].
Despite this critical role of the adherens junction , the tight junction is the primary determinant of paracellular permeability . It is, therefore, not surprising that alterations in tight junction structure and function are associated with increased permeability in IBD. For example, the normally complex anastomosing network of tight junction strands seen by freeze-fracture electron microscopy is simplified in IBD [26]. Moreover, the expression and distribution of individual tight junction proteins is altered in IBD [21, 22, 25]. Finally, increased expression and enzymatic activity of myosin light chain kinase, a key regulator of the perijunctional actomyosin ring and tight junction permeability, have been associated with active IBD [24]. As discussed below, this appears to be the primary intracellular signaling pathway by which TNF and related cytokines disrupt tight junction barrier function [48, 49, 57, 58].
Discovery of the claudins , a large family of proteins now recognized to define tight junction ion selectivity and to be essential for the development of the paracellular barrier, was a major breakthrough in tight junction biology [38]. The availability of reagents suitable for analyzing individual claudin proteins in human specimens revealed that expression of claudin-2 was upregulated in IBD [21, 23]. In parallel, in vitro studies demonstrated that claudin-2 expression increased the paracellular flux of cations, e.g. Na+, and small uncharged molecules [59–61]. Furthermore, in vitro and in vivo studies have shown that claudin-2 upregulation alone is responsible for the increased pore pathway flux induced by IL-13 [61]. Thus, increased flux through claudin-2 is a potential mechanism for the elevated pore pathway paracellular permeability observed in IBD. IL-13 may not be the only mediator of claudin-2 induction in IBD [62, 63], but it is interesting to note that claudin-2 expression is greater in UC than CD, and that there is a greater elevation of IL-13 production by lamina propria mononuclear cells from UC, relative to CD [22, 23]. However, as discussed below, increased claudin-2 expression cannot fully explain the barrier defects present in IBD, and it remains uncertain whether this represents a beneficial adaptive, or detrimental maladaptive, process.
While elevated levels of IL-13 are highly relevant to IBD, the impact of IL-13 neutralization on intestinal permeability and disease activity in human IBD is untested. In contrast, TNF neutralization reduces disease severity and also restores intestinal barrier function in IBD patients [64]. While this does, in part, reflect healing of mucosal ulcers and global immune downregulation , TNF also plays a critical role in tight junction regulation. For example, studies in mice have shown that acute T cell activation causes a TNF-dependent increase in intestinal permeability. This is associated with endocytosis of the tight junction protein occludin and redistribution of the cytoplasmic plaque protein ZO-1 [47, 49]. Occludin internalization is directly related to TNF-induced barrier loss, as occludin overexpression in transgenic mice limits increases in permeability and prevents diarrhea following acute TNF exposure [47]. More recent in vitro studies have shown that occludin knockdown also protects from such barrier loss as occludin deficient monolayers are insensitive to TNF exposure. This barrier regulation relies on direct interactions between occludin and ZO-1 [46, 65]. This failure of occludin-deficient epithelia to regulate tight junction permeability following TNF exposure is likely explained by the fact that these monolayers have increased tight junction leak pathway permeability at baseline [46]. Notably, occludin expression is reduced in chronic experimental colitis as well as human IBD [22, 66].
TNF-induced barrier loss has also been shown, in vitro and in vivo, to require myosin light chain kinase- (MLCK-) dependent myosin II regulatory light chain (MLC) phosphorylation [48, 49]. TNF-induced MLCK activation precedes occludin internalization , as genetic or pharmacological MLCK inhibition prevents occludin redistribution, barrier loss, and diarrhea [49]. In contrast, inhibition of occludin endocytosis prevents barrier loss but does not block MLC phosphorylation. Beyond enzymatic activation, TNF increases MLCK transcription and protein synthesis in vitro and in vivo [67, 68]. Moreover, MLCK expression and enzymatic activity are both increased in human IBD, particularly in association with active disease [24]. MLCK activation is also required for barrier dysregulation by other cytokines relevant to IBD, including lymphotoxin-like inducible protein that competes with glycoprotein D for herpes virus entry on T cells (LIGHT), and interleukin-1β (IL-1β) [58, 69, 70]. As discussed below, these and other data [28] indicate that MLCK inhibition may have therapeutic efficacy in IBD, particularly as a maintenance therapy.
The ability of pro-inflammatory cytokines to regulate tight junction barrier function may explain the presence of increased permeability in human IBD. For example, the permeability increases that precede CD reactivation may be secondary to limited immune activation. This model is also consistent with the observation that barrier defects in first-degree relatives of CD patients are associated with mutations in NOD2, a known immunoregulatory gene [20]. However, the fact that these relatives, as well as other individuals with reduced barrier function, do not have disease as well as the absence of spontaneous disease in animal models of impaired intestinal tight junction barrier function demonstrate that increased intestinal permeability alone is insufficient to cause IBD [10, 71, 72]. How is it possible to integrate these data and understand the relationship between intestinal barrier function and disease? One animal model that clarifies the relationship between immune signaling and barrier function in disease is the interleukin-10 knockout (IL-10 KO) mouse . This model is relevant to human disease, as polymorphisms of both the IL-10 promoter and the IL-10 receptor have been linked to ulcerative colitis and very eraly onset IBD [73, 74].
IL-10 knockout mice develop spontaneous colitis when housed under ‘normal’ specific pathogen-free conditions. However, like human IBD, development of disease in IL-10 KO mice is clearly multifactorial. Disease penetrance and presentation vary with genetic background and among different animal facilities, and disease does not develop at all in germ-free mice. Moreover, when disease does occur in IL-10 KO mice, clinical symptoms are not present at birth, but develop only after weeks or months [75]. Intestinal barrier defects are detectable in a large proportion of IL-10 KO mice within 4 weeks of birth, but are absent in antibiotic-treated or germ-free mice [76]. Thus, given that the primary defect in these mice is loss of the immunoregulatory cytokine IL-10 , one can conclude that intestinal permeability defects arise secondary to an interaction between the immune system and luminal microbiota. This suggests that the barrier defects in IL-10 KO mice are a sensitive indicator of mucosal immune activation. Another study suggests that enhancing mucosal barrier function by undefined mechanisms may limit disease in IL-10 KO mice [77]. Thus, early permeability defects may contribute to disease progression in IL-10 KO mice.
Another observation that suggests a contribution of barrier defects to disease evolution in IL-10 KO mice is the synchronization of disease onset by treatment with the nonsteroidal anti-inflammatory drug (NSAID) piroxicam [78]. While the mechanisms by which piroxicam exerts these effects are unclear, NSAIDs are known to cause epithelial injury, including erosions that can enhance mucosal bacterial invasion in IL-10 KO mice. Thus, one might hypothesize that piroxicam triggers disease in IL-10 KO mice by enhancing intestinal permeability, although not by increasing tight junction permeability . This suggests that a transient elevation of intestinal permeability in a genetically susceptible host, e.g. IL-10 KO mice , is sufficient to initiate chronic disease. This model could also explain the observation, made in humans, that an acute episode of bacterial gastroenteritis is associated with increased risk of developing IBD [79]. Thus, the IL-10 KO mouse model emphasizes the interaction between genetic susceptibility, immune activation, intestinal microbiota, and epithelial barrier function in development of human IBD.
Barrier Defects and Mucosal Immune Regulation
The data discussed above suggest that barrier function can be a sensitive indicator of mucosal immune activation and that increased permeability may be able to amplify the effects of such immune activation. However, while the role of primary epithelial barrier dysfunction in IBD pathogenesis remains controversial, the associations are striking. For example, barrier defects precede clinical manifestations of disease in IL-10 KO mice , in which the primary defect is immunological [76]. Increased intestinal permeability has also been reported prior to clinical disease onset in the outbred SAMP1/YitFc mouse model of IBD [80]. Bone marrow chimera studies suggest that the primary defect in these mice is present within a radioresistant , non-bone marrow-derived cell population [80]. However, the precise abnormality that causes disease in SAMP1/YitFc mice has not been identified.
Studies of mice with targeted barrier defects provide further evidence that tight junction permeability may contribute to disease progression. One informative example comes from mice lacking junctional adhesion molecule A (JAM-A) , an immunoglobulin superfamily member that facilitates tight junction assembly and leukocyte transmigration across endothelia and epithelia [72, 81]. Loss of JAM-A expression within intestinal epithelia led to increased colonic epithelial apoptosis in colitis, providing one potential mechanism by which JAM-A deficiency augments disease. Consistent with this, colonic neutrophil accumulation was increased in JAM-A KO mice , and these mice were also hypersensitive to dextran sulfate sodium- (DSS-) induced epithelial injury and colitis [72]. Because JAM-A is normally expressed ubiquitously, defects in these mice could not be linked directly to loss of intestinal epithelial JAM-A . However, endothelial specific JAM-A KO mice did not demonstrate increased DSS susceptibility, demonstrating that endothelial JAM-A loss was not responsible for the observed phenotype. In addition, the observation that intestinal epithelial, but not endothelial, JAM-A expression is reduced in human and experimental IBD supports the hypothesis that loss of intestinal epithelial JAM-A is responsible for the phenotype of JAM-A KO mice [72]. Although the phenotype of the JAM-A KO mice is informative and implicates tight junction proteins in epithelial repair processes, the cause of JAM-A loss in human and experimental IBD remains unclear. Moreover, despite the experimental simplicity of the model, the DSS model of colitis primarily reflects responses to acute epithelial damage and does not reflect the pathogenesis of human IBD. Some workers have attempted to correct this using a chronic DSS model, but that model suffers from the same problem in that it is caused by ongoing direct epithelial injury, which is unlikely to be the cause of human IBD.
Increased transcription and enzymatic activity of intestinal epithelial MLCK are well-documented in human and experimental IBD, and are regulated by proinflammatory cytokines such as TNF. Moreover, MLCK is required for in vitro and in vivo TNF-induced barrier loss [48, 49]. To model this form of barrier loss, transgenic mice that express constitutively active MLCK (CA-MLCK ) from an intestinal epithelial-specific promoter were created [71]. These mice have increased small intestinal and colonic paracellular permeability and exhibit mucosal immune activation, as evidenced by elevated mucosal IFNγ (gamma), TNF, IL-10, and IL-13 transcription and increased numbers of colonic lamina propria T cells. However, despite mucosal immune activation, the mice thrive and do not develop spontaneous disease. While this absence of disease may reflect the activity of immunoregulatory cells, such as those responsible for the increase in IL-10 transcription, expression of CA-MLCK in RAG1-deficient (RAG1−/−) mice, which lack regulatory T cells, also fails to induce disease [71]. However, when effector T lymphocytes are introduced into CA-MLCK/RAG1−/− mice, using the CD4+CD45Rbhi T lymphocyte adoptive transfer model of colitis, the onset of disease is accelerated and severity is increased relative to RAG1−/− littermates that lack CA-MLCK [71]. Therefore, these CA-MLCK transgenic mice recapitulate the observation in humans that primary barrier dysfunction is insufficient to cause disease, e.g. in healthy relatives of CD patients, and also provide evidence that barrier dysfunction can accelerate the onset and enhance the severity of immune-mediated colitis.
This interplay between TNF exposure, immune activation, MLCK, and intestinal barrier function has been further studied using immune-mediated adoptive transfer colitis in RAG1 knockout mice lacking either TNF receptor 1 (TNFR1) or TNFR2 [28]. CD4+CD45Rbhi T lymphocyte adoptive transfer into RAG1 deficient mice results in increased MLCK protein expression along with increased transcription of TNFR2 but not TNFR1 [28]. Adoptive transfer into RAG1 knockout mice lacking TNFR2 failed to induce MLCK expression, and mice lacking either TNFR2 or MLCK were at least partially protected from immune-mediated colitis [28]. These data, along with in vitro observations showing that TNF-induced, MLCK-dependent barrier loss requires TNFR2 function indicate that it is epithelial TNFR2, and not TNFR1, that drives MLCK transcription [28]. Remarkably, colitis-associated claudin-2 upregulation was also limited in MLCK-deficient mice (Fig. 3.2), demonstrating the in vivo interplay between paracellular leak and pore pathways [28]. Nevertheless, mice lacking either epithelial MLCK or TNFR2 did eventually develop colitis and unrestricted pathway barrier loss [28]. This occurred as a consequence of immune-mediated epithelial damage. Consistent with this, epithelial MLCK knockout mice were not protected from acute, TNF-induced epithelial apoptosis or DSS colitis [28]. These data reinforce the notion that MLCK inhibition may be beneficial in preventing relapse or progression to advanced IBD but may not be useful in treatment of active disease with ulcerations.


Fig. 3.2
Both leak and pore paracellular pathways are altered in immune-mediated colitis. All mice are RAG1−/− to allow for adoptive transfer colitis. Myosin light chain is phosphorylated in immune-mediated colitis in an MLCK-dependent manner (top panels). Transgenic, intestinal epithelial-restricted expression of constitutively active-MLCK restores disease-associated MLC phosphorylation to long MLCK−/− mice. Claudin-2 expression parallels MLC phosphorylation in CD4+CD45RBhi colitis (bottom panels). Increases in MLC phosphorylation and claudin-2 expression correspond with worsened clinical outcomes in early stages of colitis. Scale bar = 10 μm. From Su et al., 2013
Isolated Barrier Defects Are Insufficient to Cause Disease
While data above link increased intestinal permeability to disease severity and relapse, mild barrier defects may actually trigger regulatory processes that prevent inappropriate immune responses in immunocompetent hosts. The clearest example of this may be data indicating that, despite the presence of increased permeability in patients with infectious gastroenteritis, most of these individuals recover fully and do not develop IBD [79]. Recent studies in mice have provided some insight into the immunoregulatory mechanisms that prevent development of chronic disease in this situation. In wild-type mice, when mucosal damage was induced by intrarectal ethanol administration, the resulting transient increase in permeability induced a population of regulatory T cells characterized by the surface expression of the TGFβ pro-peptide latency-associated peptide (LAP) and also increased IL-10 production by lamina propria mononuclear cells [82]. Moreover, this mild mucosal damage protected mice from colitis induced by subsequent intrarectal TNBS administration. While the detailed mechanisms by which transient increases in permeability invoke immunoregulatory responses are incompletely characterized, it is notable that mucosal IL-10 production was also increased in CA-MLCK transgenic mice [71]. Thus, chronic barrier defects may also induce immunoregulatory responses that prevent disease. On this basis, it can be inferred that, in an immunocompetent host , limited barrier defects induce a robust immunoregulatory response that prevents disease. If correct, this hypothesis suggests that the difference between CD patients and their healthy relatives with increased permeability may be the quality of this regulatory response and the ability to manage inflammation induced by barrier loss. Overall, these studies emphasize the need for further clarification of the complex interactions between the intestinal epithelial barrier and mucosal immune system.
A Multifactorial Model of IBD
The data discussed above suggest a model of IBD in which paracellular defects, mucosal immune activation, and the luminal microbiota are interrelated and act cooperatively in the prevention, induction, and progression of disease (Fig. 3.3). The epithelial barrier balances the interaction between immunostimulatory luminal materials and the mucosal immune system. In immunocompetent subjects, mild barrier defects activate regulatory immune responses that compensate for and prevent excessive immune activation. In contrast, barrier defects may trigger inappropriate immune activation and cytokine release in subjects who are unable to elicit effective immunoregulatory responses. These cytokines may then signal to epithelial cells to cause further increases in paracellular permeability, which, in turn, allows further transmucosal passage of immunostimulatory materials and an even greater degree of immune activation. Thus defects in either barrier function or immune activation may initiate the cycle of barrier-immune dysregulation, but defects in both are necessary for perpetuation of this cycle and disease pathogenesis. This model also suggests that, in addition to the immunosuppressive and immunomodulatory agents that have been central to IBD therapy, it may be possible to break the cycle by restoring barrier function prior to onset of clinical disease.


Fig. 3.3
The epithelial barrier regulates the balance between the luminal environment and mucosal immunity . Breaches in the epithelial barrier allow luminal material to cross the epithelium, where it may then activate mucosal immune responses. Under conditions of appropriate immune regulation, regulatory T cells (Tregs) produce anti-inflammatory signals to counter the pro-inflammatory immune response. A subset of these Tregs, those expressing latency-associated peptide (LAP), secrete IL-10 and TGF-β to attenuate disease. In contrast, under disease conditions, luminal material may activate Th1 or Th2 T cells. Th1 cells secrete TNF and IFNγ, which increase paracellular leak pathway permeability via MLCK-dependent mechanisms. Alternatively, Th2 cells secrete IL-13, which increases pore pathway permeability by upregulating claudin-2 expression. These increases in paracellular permeability may allow the passage of additional luminal material across the barrier. Amplification of this cycle of inflammation eventually leads to apoptosis of epithelial cells. Under these conditions, intestinal permeability is largely tight-junction independent and unrestricted. Due to the tight junction-independent nature of this unrestricted pathway in more advanced disease, therapeutics designed to restore tight junction barrier function will likely be most useful in early disease or in maintaining remission. Adapted from Su et al. 2013
Barrier Restoration as a Future Therapeutic Intervention
Current immunosuppressive and immunomodulatory approaches are often effective in IBD management, but they treat the inflammation responsible for tissue damage rather than the underlying cause of inflammation. It has been recognized for some time that the epithelial barrier can be dysregulated by proinflammatory stimuli. The model described, which explains available data from in vitro and in vivo models as well as human studies, suggests that the barrier also regulates the immune response. Although increased paracellular permeability is a normal physiological response that is often beneficial and, in an immunocompetent host, triggers an immunoregulatory response, reduced barrier function may also be critical to disease pathogenesis. This is particularly true in individuals with immunoregulatory defects. For example, barrier loss precedes colitis in IL-10-deficient mice. Conversely, barrier restoration may blunt disease development. Similarly, genetic deletion of intestinal epithelial long MLCK, which is necessary for TNF-induced barrier loss, partially protects immunodeficient mice from colitis induced by CD4+CD45Rbhi adoptive transfer. These data suggest that barrier restoration may be therapeutically useful, particularly in patients with IL-10 receptor mutations or single nucleotide polymorphisms (SNPs) within the IL10 promoter.
Development of barrier restorative agents will require targeting the pore, leak, and unrestricted paracellular pathways, all of which are disrupted at different stages of colitis. While claudin-2 must be responsible for some of the increases in pore pathway permeability in colitis, no modulators of claudin-2 pore function are currently available.
Epithelial MLCK is clearly an attractive druggable target to restore leak pathway permeability early during disease development and promote maintenance of remission. However, the different MLCK isoforms expressed in smooth muscle and nonmuscle cells are encoded by a single gene and, therefore, have common catalytic and calmodulin-dependent regulatory domains [83, 84]. Knockout mouse studies clearly show that inhibition of smooth muscle MLCK has disastrous consequences [84]. Thus, alternatives to currently available MLCK inhibitors , all of which target the catalytic domain, will be necessary if MLCK is to be a viable therapeutic target.
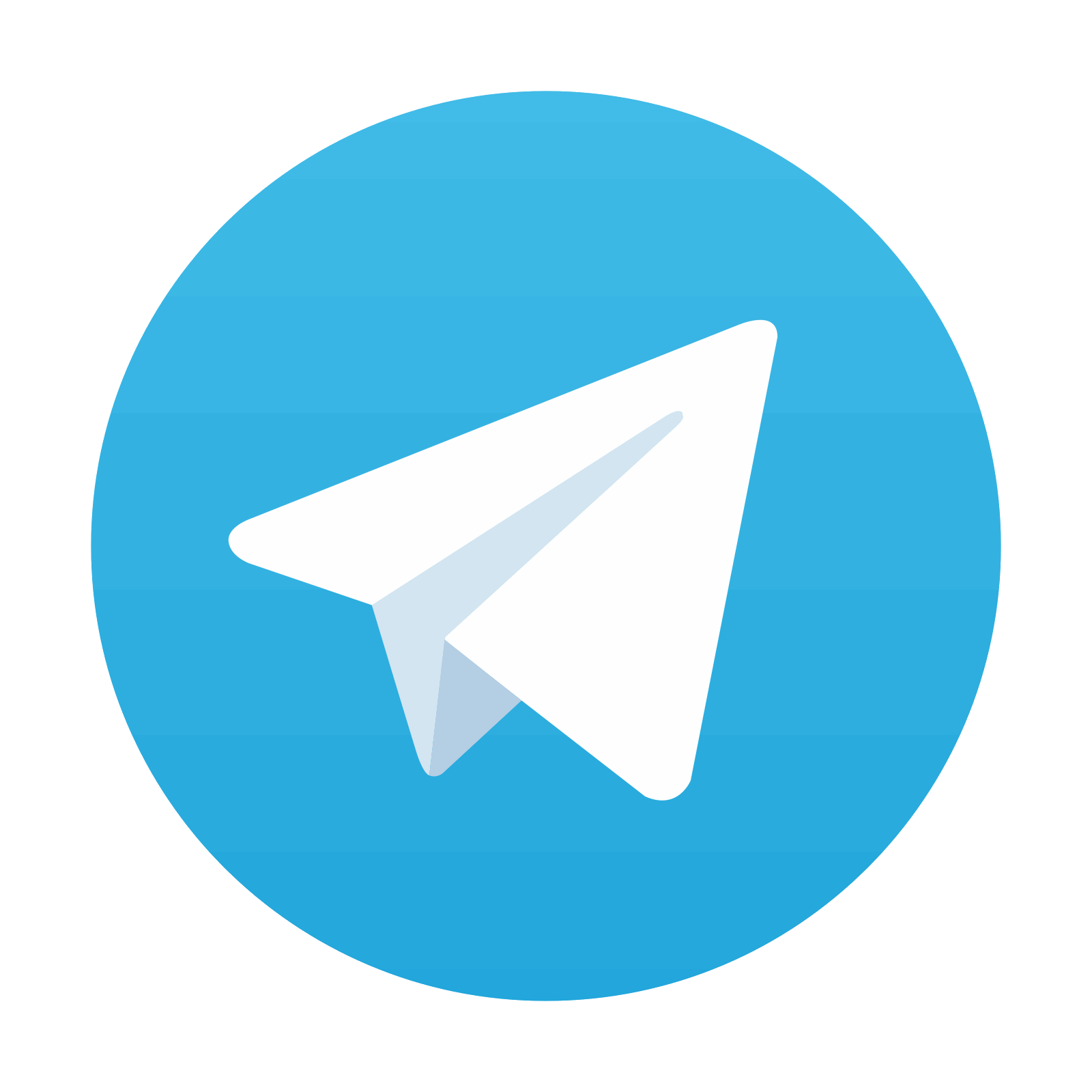
Stay updated, free articles. Join our Telegram channel

Full access? Get Clinical Tree
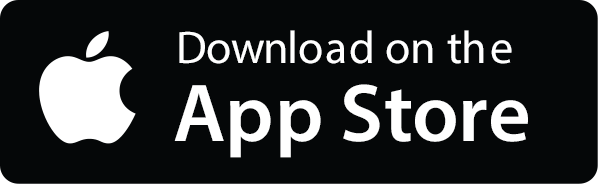
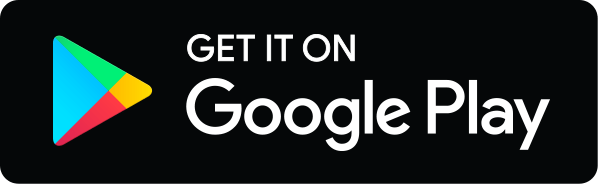