Fig. 14.1
(a) Representation of unhindered ordinary diffusion demonstrating a non-uniform distribution and movement throughout the solvent. (b) Representation of convection without diffusion moves all molecular species equally and does not result in separation
Diffusive therapies rely on several phenomena including ordinary diffusion and forced diffusion. Ordinary (or Fickian) diffusion describes the molecular movement induced by random movements coupled to the non-uniform distribution in space of the species. This is shown in Fig. 14.1b, where eventual uniform distribution of molecular species will be seen within a solution. The rate of this process is defined by the diffusive flux. The flux is defined as the number of molecules that pass through a unit area in a unit time. Clearly the flux is also dependent on any concentration gradient involving the diffusing substance and this process, for any given molecular species A, is described by Fick’s law [3]:
where
is the gradient and D is the Fickian diffusivity. Ordinary diffusion effectively scatters molecular species throughout the medium and is dependent on numerous factors including molecular size and properties of the solution. Separation, however, is determined by the introduction of a further element such as a membrane or gel. These will affect diffusion coefficients significantly, allowing molecular separation to occur, which in turn is limited by the available concentration gradients. Forced diffusion describes the application of an external force which acts differently on the molecular species present facilitating separation. Thus in a dialysis machine separation is enhanced not only by the membrane but by concentration gradients (ordinary diffusion) but also by pressure changes through the application of blood pumps (forced diffusion). In practice this results in the passage of a molecular species along a concentration gradient, and this solute transport can be expressed as:
where DTA are the diffusion coefficient (which varies with the cubed root of the molecular weight), temperature and the surface area of the membrane and ∂c is the concentration gradient across the membrane with ∂d being the membrane thickness. This differs from convective based treatments (Fig. 14.2) where clearance of a molecular species is, as indicated, driven by hydrostatic pressures and convection. The hydrostatic pressure driving convection is that pressure generated across the membrane (TMP). This transmembrane pressure is defined as:
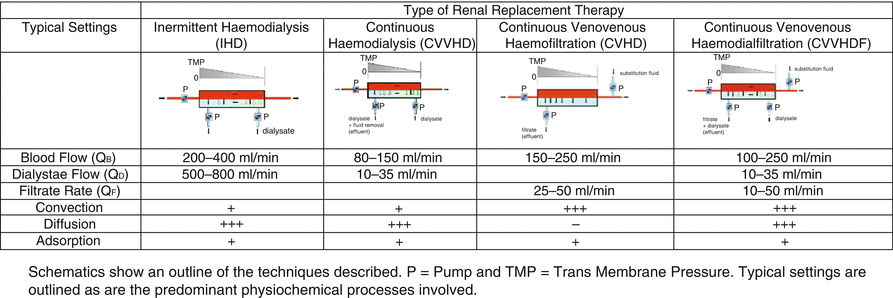
where Pb is the hydrostatic pressure in the blood compartment and Pd is the hydrostatic pressure on the ultrafiltrate side of the membrane. The oncotic pressure is given by π. It follows from this equation that convective flux (Jf) of any given molecular species will be given by:
with Kf being the membrane permeability coefficient. The rate at which molecular species cross the membrane depends on the membrane rejection coefficient (σ) which is effectively zero for small species such as urea but approaches 1 for larger molecules such as albumin. The sieving coefficient (Sc) is given by:

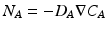

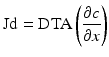
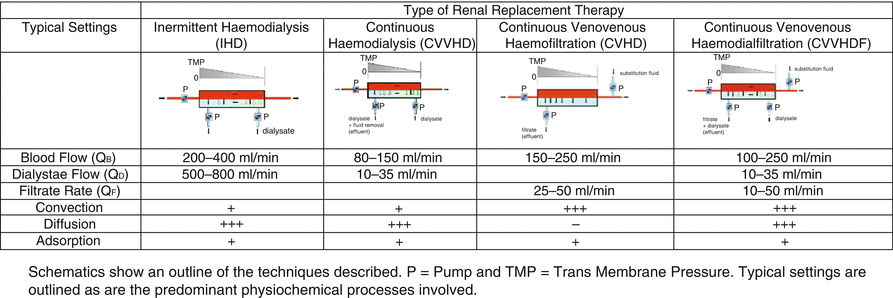
Fig. 14.2
Schematics show an outline of the techniques described. P Pump and TMP Transmembrane Pressure. Typical settings are outlined, as are the predominant physiochemical processes involved
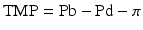
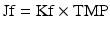

This can be determined by measuring the concentration of a given solute in the plasma water and the ultrafiltrate. Thus a simple view of solute clearance (K) in convective treatments is the product of:
where Qf is the ultrafiltration rate. It follows that where Sc = 1, the clearance is equal to Qf. Solute clearance using diffusion-based systems may be calculated from:
with Qdo and Cdo being the dialysate effluent flow and solute concentration in the effluent dialysate (that leaving the dialyser). Cbi is the concentration of the solute of interest entering the dialyser [4]. In summary, diffusion provides the main basis for the separation of molecular species in dialysis aided by convection, whereas in filtration convection is aided by diffusion, and as such the two processes often act simultaneously with any division being somewhat artificial.


Key Messages
Convection and diffusion are essential processes needed to drive molecular separation.
Convective therapies transport solutes and solvent across a semipermeable membrane through the application of a transmembrane pressure gradient.
Diffusive therapies rely on solute transport across a semipermeable membrane driven by a concentration gradient such that the solute concentration will tend to reach equilibrium in the available distribution space on both sides of the membrane.
Convection and diffusion often occur simultaneously and as such any distinction is somewhat artificial.
14.2 Types of Renal Replacement Therapy for AKI: Intermittent versus Continuous
RRT for AKI may be applied in several guises. In essence these can be simplistically thought of as being continuous therapies, intermittent therapies and more recently hybrid technologies. Although each technique may have its proponents, there are advantages (and disadvantages!) of each mode. All extracorporeal techniques share many features including access to the circulation as well as an extracorporeal circuit offering molecular separation the nature of which is technique dependent. There are many acronyms used when describing the various techniques to provide renal support. The consensus recommends the use of the term renal replacement therapy (RRT) which may be intermittent or continuous and described as IRRT or CRRT, respectively. The extracorporeal circuit type is then described, for example, pumped venovenous—VV. This is followed by the method of blood purification, for example, haemofiltration—H, haemodiafiltration—HDF and haemodialysis—HD. Therefore, continuous venovenous haemodiafiltration would be represented by CVVHDF [5, 6].
14.2.1 Intermittent Techniques
Intermittent techniques, specifically intermittent haemodialysis, are the standard treatment modality for renal replacement in patients with end stage renal disease. In intermittent haemodialysis, blood is pumped into a dialyser containing two fluid compartments with blood in the first compartment being pumped along one side of a semipermeable membrane while a crystalloid solution (dialysate) is pumped along the other side in a contraflow fashion. As described, the concentration gradients of solute between blood and dialysate lead to the desired biochemical changes. In order to prevent filtration of the dialysate back into the bloodstream, this compartment is under negative pressure relative to the blood compartment.
Compared to continuous techniques, relatively high blood flows are used (200–400 mL/min) coupled with dialysate flow rates of 500–800 mL/min (see Fig. 14.2). Such flows enable high solute clearance rates over a relatively short period of time which may be associated with complications in the critically ill patient. For example, rapid removal of urea during dialysis may be associated with the dialysis disequilibrium syndrome. This is a clinical phenomenon of acute central nervous system dysfunction attributed to cerebral oedema occurring during or just after renal replacement therapy. Although generally accepted that cerebral oedema plays a major role in the development of the dialysis disequilibrium syndrome, the definitive pathophysiology is incompletely described [7, 8]. Of the mechanisms proposed, the increased urea removal from the plasma over that of the cerebrospinal fluid resulting in movement of water into the brain—the so-called reverse urea effect hypothesis—is probably the most universally accepted. Features of the dialysis disequilibrium syndrome include nausea, headache, vomiting, tremors and seizures [9]. There is no treatment as such for the dialysis disequilibrium syndrome, and despite a lack of evidence base, preventive measures include shorter session length, lower blood flow rates and use of smaller surface area filters. Low-dose continuous therapies could also be applied.
Perhaps, in critically ill patients, intermittent therapies result in higher rates of hypotension, which is significantly influenced by the amount of fluid removal required during each dialysis session and often prevents achievement of desired fluid balance (Table 14.1). To minimize the adverse haemodynamic effects of intermittent therapies, several groups have described techniques whereby modifications are made to avoid the dialysis disequilibrium syndrome as well as haemodynamic intolerance [10]. These include:
Table 14.1
Outline of techniques viewed suitable for RRT in AKI
Therapeutic aim | Patient characteristics | Suitable techniques < div class='tao-gold-member'>
Only gold members can continue reading. Log In or Register a > to continue
![]() Stay updated, free articles. Join our Telegram channel![]() Full access? Get Clinical Tree![]() ![]() ![]() |
---|