Transplantation Immunobiology
Didier A. Mandelbrot
Mohamed H. Sayegh
The immune response to a transplanted graft can be divided into three phases: recognition of foreign antigens, activation of antigen-specific lymphocytes, and the effector phase of graft rejection. If the transplant is between genetically different individuals of the same species, it is referred to as an allogeneic graft, or allograft. This allograft stimulates an immune response (alloresponse), which is mediated by alloreactive lymphocytes. This chapter focuses on the immune response to allografts. However, grafts can also be autologous (from an individual back onto that same individual), syngeneic (between genetically identical individuals), or xenogeneic (between individuals of different species).
The principal function of the immune system is to defend against infections. Fundamental to this function is the capacity of the immune system to discriminate between self and nonself antigens. The system is highly evolved in its ability to defend against various microbial infections and normally does not react against itself—the tissues of the host. The immune response to allografts is one example of a response to nonself antigens. Because transplantation is an unlikely event in the life of any organism, it has long been surprising that the immune system retains such a powerful ability to recognize and reject transplanted allografts. The explanation can now be provided in considerable detail.
THE RECOGNITION OF ALLOGRAFTS
Genetics of Allograft Rejection
Studies of the acceptance and rejection of tissue grafts exchanged between inbred mice established the following four basic principles that govern graft rejection:
1. Grafts exchanged between members of one inbred strain (syngeneic grafts) will survive.
2. Grafts between animals of different inbred strains (allografts) will be rejected.
3. Grafts from a parent to an Fl offspring of two different strains will survive (because the Fl offspring recognizes the graft from the homozygous parent as “self”).
4. Grafts from an Fl offspring to a parent will be rejected (because the parent recognizes the graft from the heterozygous Fl as “nonself”).
These results led to the realization that graft rejection was controlled by genes whose inheritance followed simple mendelian rules. The genes that determine the rejection or acceptance of tissue grafts are present in a locus on chromosome 6 that was named the major histocompatibility complex (MHC) (Fig. 2.1A). The gene products are MHC antigens or molecules. In humans, syngeneic grafts are those between identical twins—they survive because donor and recipient have identical MHC molecules. When donors and recipients of grafts differ in their histocompatibility antigens, the allogeneic grafts are rejected, unless the recipient receives immunosuppression. Genes other than MHC play
smaller roles in graft rejection and are called minor histocompatibility genes. MHC incompatibilities lead to more vigorous rejection than minor incompatibilities, and in general, the greater the differences in the MHC, the more rapid the rejection.
smaller roles in graft rejection and are called minor histocompatibility genes. MHC incompatibilities lead to more vigorous rejection than minor incompatibilities, and in general, the greater the differences in the MHC, the more rapid the rejection.
Major Histocompatibility Complex Molecules
The central event in the initiation of an alloresponse is the recognition of MHC/peptide complexes by a T-cell receptor. The MHC encodes a group of highly diverse cell surface proteins. Although these gene products were found in the 1940s to be the principal determinants of graft rejection, and thus named “histocompatibility” genes, their broader importance in controlling all immune responses to foreign antigens was only established in the 1960s. Many details of the structure and function of MHC molecules have since been established, and these are relevant to all responses to self and nonself antigens. Unlike antibody molecules, which can bind to essentially any class of molecule, including carbohydrates, lipids, and proteins, the antigen receptors of T cells primarily recognize peptides that are bound to MHC molecules. Consequently, T-cell activation is critically dependent on the MHC.
There are two types of MHC molecules: class I and class II molecules. In humans, the MHC genes are called human leukocyte antigen (HLA) genes and include the class I genes HLA-A, HLA-B, and HLA-C and the class II genes HLA-DP, HLA-DQ, and HLA-DR. There are two critical features of MHC molecules that determine their importance as histocompatibility antigens. First, they
are highly polymorphic: unlike most proteins, which are the same in all humans, such as most structural proteins and enzymes, each MHC locus can express any one of hundreds of different molecules. For example, HLA-A1, HLA-A2, HLA-A3, and so on are different enough from each other to determine self and nonself, and different molecules are expressed in each individual. Each of the MHC loci is polymorphic, and the set of different MHC molecules, or alleles,expressed on one chromosome is called a haplotype. A genotype is the sum of two haplotypes. The second critical feature of the MHC is that its genes are codominantly expressed—an individual expresses alleles from both chromosomes at each locus. Therefore, the MHC genotype of an individual consists of 12 different MHC molecules (two alleles from each of six loci). This explains why grafts from an Fl offspring are rejected by each parent: the Fl will express MHC molecules derived from both parental alleles, and the parent will recognize the other parent’s alleles as foreign.
are highly polymorphic: unlike most proteins, which are the same in all humans, such as most structural proteins and enzymes, each MHC locus can express any one of hundreds of different molecules. For example, HLA-A1, HLA-A2, HLA-A3, and so on are different enough from each other to determine self and nonself, and different molecules are expressed in each individual. Each of the MHC loci is polymorphic, and the set of different MHC molecules, or alleles,expressed on one chromosome is called a haplotype. A genotype is the sum of two haplotypes. The second critical feature of the MHC is that its genes are codominantly expressed—an individual expresses alleles from both chromosomes at each locus. Therefore, the MHC genotype of an individual consists of 12 different MHC molecules (two alleles from each of six loci). This explains why grafts from an Fl offspring are rejected by each parent: the Fl will express MHC molecules derived from both parental alleles, and the parent will recognize the other parent’s alleles as foreign.
The evolutionary benefit of the extensive polymorphism of MHC molecules is that a wide variety of microbial peptides can be bound and presented to T cells, thus successfully initiating responses to infections. However, this same polymorphism creates a practical barrier to successful organ transplantation because the chances of matching the MHC of an unrelated organ donor to that of a recipient is low. The degree of MHC matching between an allograft donor and recipient plays a role in determining the chances of a successful graft survival. In clinical transplantation, the most important MHC genes are HLA-A, HLA-B, and HLA-DR because donor-recipient mismatches at these loci have a much greater effect on risk for rejection than mismatches at the other MHC loci. The antigenic determinants of these six alleles are the focus of attempts at HLA matching to improve graft survival (see Chapter 3 and Fig. 3.3).
The molecular structure of MHC molecules has been meticulously defined. A class I molecule is composed of a polymorphic a or heavy chain (44 kDa) and a noncovalently associated invariable (nonpolymorphic) light chain, β2microglobulin (12 kDa) (Fig. 2.1B). A class II molecule is composed of polymorphic α and β chains of similar molecular weight (32 kDa), covalently bound to each other (Fig. 2.1B). A critical feature of both classes of MHC is the presence of a peptide-binding groove (see front cover). The specific amino acids that line this groove determine which specific peptides can bind for presentation to T cells. Class I molecules bind peptides that are 9 to 11 amino acids long, whereas class II molecules bind peptides that are 13 to 30 amino acids long.
Class I and class II MHC molecules have different expression patterns. Class I molecules are expressed on essentially all nucleated cells, whereas class II molecules are expressed only on professional antigen-presenting cells (APCs), including dendritic cells, B lymphocytes, and macrophages. Cytokines play an important role in modifying the expression of MHC molecules. For example, interferons (IFNs), particularly IFN-γ, upregulate levels of MHC class I. IFN-γ also increases MHC class II levels on macrophages and can induce class II expression on cells not traditionally considered APCs, including endothelial and epithelial cells. Thus, essentially all cells in a graft can express both MHC class I and class II molecules and be potential targets of an alloresponse.
Both class I and class II molecules are stably expressed at the cell surface only if peptides are present in their binding grooves. Class I molecules bind peptides derived from proteins in the cytoplasm of cells, which are often proteins that are synthesized intracellularly, such as viral proteins (the cytosolic, or endogenous, pathway). In contrast, class II molecules bind extracellular proteins that have been brought into a cell’s vesicles by endocytosis (the vesicular, or exogenous, pathway). In both cases, the complex of MHC molecule and peptide is recognized by a
T-cell receptor (TCR) to initiate T-cell activation. In the absence of a microbial infection or foreign graft, the peptides presented by MHC molecules are derived from self proteins, and some of these peptides have been shown to be derived from self-MHC molecules. The significance of the self-MHC peptides present on MHC molecules is unclear, but their possible immunologic role has led to efforts to design MHC peptide molecules as therapies to block the rejection of allografts.
T-cell receptor (TCR) to initiate T-cell activation. In the absence of a microbial infection or foreign graft, the peptides presented by MHC molecules are derived from self proteins, and some of these peptides have been shown to be derived from self-MHC molecules. The significance of the self-MHC peptides present on MHC molecules is unclear, but their possible immunologic role has led to efforts to design MHC peptide molecules as therapies to block the rejection of allografts.
Pathways of Alloantigen Presentation
Although the immune system evolved primarily to respond to foreign microbial peptides presented by self-MHC molecules, the strong response to allografts is highly conserved in evolution. In humans, between 1% and 10% of T cells respond to a given allogeneic MHC molecule. During their maturation in the thymus, T cells that respond to self antigens are deleted (negative selection), whereas those that are specific for foreign peptides displayed by self-MHC molecules are allowed to develop (positive selection). These same positively selected T cells also recognize foreign MHC molecules in grafts and induce graft rejection. The nature of the MHC molecules recognized by alloreactive T cells varies according to the pathway of alloantigen presentation.
Direct Antigen Presentation
The response of recipient T cells to intact MHC/peptide complexes on APCs from a graft is called direct allorecognition. That is, the APCs in the graft directly present alloantigens (the foreign MHC molecules) for recognition by alloreactive T cells. The high alloreactivity of the T-cell repertoire is a result of cross-reactivity: T cells that normally recognize self-MHC/microbial peptide complexes (Fig. 2.2A) are also capable of recognizing foreign MHC/peptide complexes because the complexes are structurally similar. The T cells responding to direct antigen presentation recognize determinants on the allogeneic MHC molecule itself (Fig. 2.2B) as well as structures determined by both MHC and peptide (Fig. 2.2C). The peptides presented by foreign MHC molecules may be derived from polymorphic proteins (i.e., MHC antigens) or nonpolymorphic proteins (e.g., an enzyme from a metabolic pathway).
Indirect Antigen Presentation
A recipient’s T cells can also respond to donor MHC peptides presented on the recipient’s own APCs. This pathway is called indirect allorecognition, to distinguish it from the direct response to intact MHC on donor APCs (Fig. 2.3). In indirect antigen presentation, donor MHC molecules are shed from their cell surface, taken up by recipient APCs, processed, and presented as peptides on recipient MHC molecules. The indirect alloresponse is analogous to the immune response to invading microbes, which are also presented to T cells as peptides displayed by self-MHC molecules. Several types of experiments have demonstrated the importance of indirect allorecognition in graft rejection. For example, skin grafts from MHC class II-deficient mice are rejected in a CD4 T-cell-dependent manner. In this experiment, CD4 T cells (which are class II MHC restricted) cannot be stimulated by the direct pathway because no MHC class II is present on donor cells. Therefore, they must be responding to recipient APCs presenting allopeptides on recipient class II molecules.
It is generally assumed that indirect antigen presentation is more important for activating CD4 T cells rather than CD8 T cells because class II molecules present peptides derived from exogenous sources, unlike class I molecules, which usually present peptides derived from endogenous sources. However, cross-priming of CD8 cells has been demonstrated, whereby MHC class I molecules can be loaded with peptides from exogenous sources. This suggests that CD8 cells also may be able to respond to indirect antigen presentation.
Acute rejection of an allograft is primarily dependent on direct allorecognition, with the ratio of T cells reactive by the direct pathway-to-indirect pathway ratio, estimated at 100:1. However, experimental evidence suggests that in chronic rejection, the indirect pathway may be more important. Because chronic rejection is a major cause of graft loss (see Chapter 10), there is great interest in understanding the indirect pathway with the goal of developing therapeutic approaches to block this pathway.
Minor Histocompatibility Antigens
Although the MHC is the major barrier to allotransplantation, studies in humans and mice have demonstrated that non-MHC molecules, referred to as minor histocompatibility antigens, can also mediate rejection. In fact, some mouse models of skin and cardiac transplantation have demonstrated that minor incompatibilities can lead to rejection that is as rapid as that seen in grafts with MHC mismatches. The clinical importance of minor antigens is demonstrated by the fact that MHC-matched siblings, unlike identical twins, require immunosuppression to prevent graft rejection. By definition, this alloresponse is targeting minor antigens. The mechanism of the alloresponse to minor antigens is similar to the response to microbial antigens, in that host MHC molecules present foreign peptides to host T cells.
One example of a minor antigen is the MHC class I-related chain A (MICA) antigen. This antigen is structurally similar to MHC class I antigens and can be expressed on a variety of cells, including monocytes, epithelial cells, and endothelial cells. MICA is polymorphic and can elicit production of antibodies in transplant recipients, more so than the closely related MHC class I-related chain B (MICB) antigen. The development of anti-MICA antibodies may be associated with worse graft survival, especially in recipients of well MHC-matched kidneys.
Sites of T-cell—Antigen-Presenting Cell Interactions
After transplantation, the first foreign antigens encountered by recipient T cells are on the vascular endothelium of the graft. Resting endothelium expresses MHC class I, and activated endothelium also expresses MHC class II molecules. Not surprisingly, inflammation of the endothelium, referred to as endotheliitisor vasculitis, is one of the hallmarks of acute graft rejection (see Chapter 14).
In addition, when an organ is transplanted, donor leukocytes are carried to the recipient as passenger leukocytes with the graft. These leukocytes are found in lymphoid tissue and in most other organs of the body, scattered within the parenchyma. The most important of these cells are dendritic cells, which are potent APCs because of their ability to express high levels of MHC molecules as well as critical accessory molecules that facilitate T-cell activation. Passenger leukocytes play an important role in allograft rejection, and in several models, the removal of these cells before transplantation prolongs graft survival. For example, both the culture of thyroid cells before transplantation and the use of antibodies to deplete dendritic cells from islet allografts prolong graft survival.
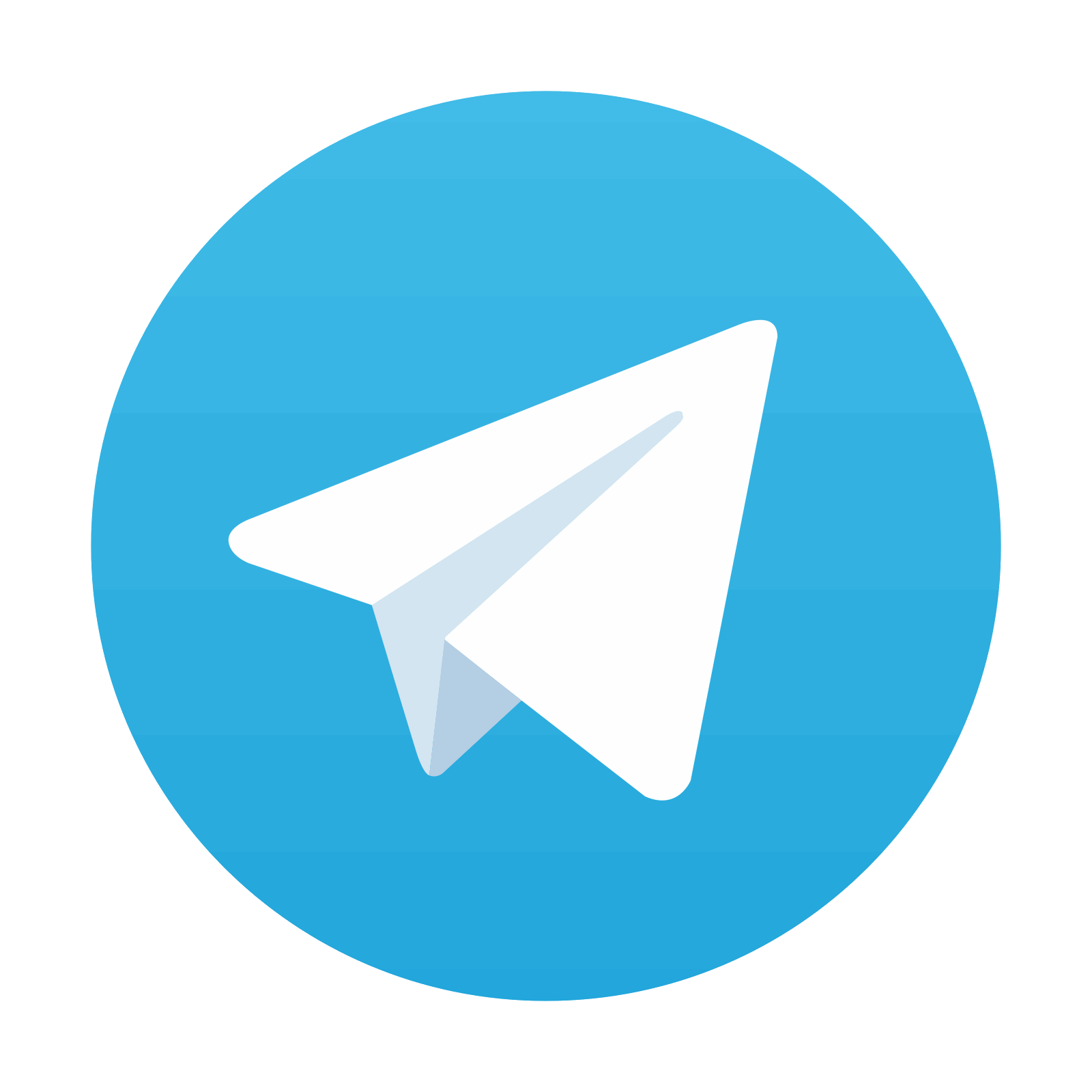
Stay updated, free articles. Join our Telegram channel

Full access? Get Clinical Tree
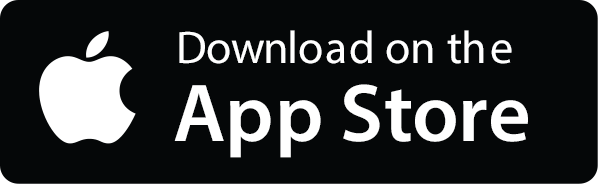
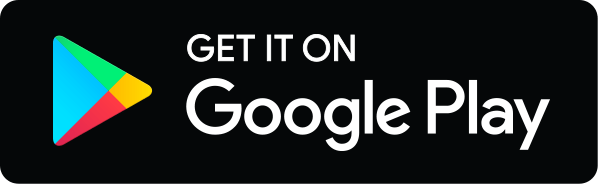