Fig. 8.1
Information derived from the analysis of human samples in viral hepatitis
In Vivo Models of HBV Infection: What Do They Mimic?
HBV infection causes acute and chronic liver diseases of variable severity. Since the virus is not directly cytopathic, the host immune system play an essential role in modulating the level of liver inflammation and in controlling the extent of virus spread and eve ntual resolution [1]. Distinct variables such as viral load, virus genotypes, route of the infection, age, sex and genetic makeup of the infected hosts are likely to influence HBV infection pathogenesis and their analyses will be greatly facilitated by reliable animal models. However, besides humans, HBV infects only chimpanzees and tree shrews but strong ethical constraints, handling difficulties as well as the high costs restrict HBV studies in chimpanzees. Related studies in tree shrews are hindered by the low infectivity of the virus and the transient nature of HBV infection in addition to the lack of reagents to analyze the immune system [2].
Important insights on the effect of virus and host variables were obtained from studies of animal species (ducks and woodchucks) that are the natural hosts of other hepadnaviruses: woodchuck hepatitis B virus (WHBV) and duck hepatitis B virus (DHBV) [3, 4]. Nevertheless, there are shortcomings of such models. WHBV and DHBV are similar but not identical to HBV and cause a different spectrum of liver diseases. For example, woodchucks infected by WHBV develop liver tumors at a much higher rate than humans as a result of a “specific” mechanism of insertional mutagenesis [5, 6], but do not express the spectrum of liver disease (asymptomatic carrier, chronic active hepatitis, liver cirrhosis) observed in HBV-infected humans. More importantly, the analysis of pathogenic mechanisms related to viral control and liver damage is hampered both in ducks and woodchucks by the lack of appropriate reagents necessary to study the complexity of host–virus interaction.
The necessity of an easy to maintain, well-defined, inbred, small-animal model for studying immune control and immunopathogenesis during HBV infection led to the production of different mouse models of HBV infection: HBV transgenic mouse, HBV hydrodynamically transfected mouse and chimeric mouse with humanized liver.
These different models have gre atly contributed to our understandings of different aspects of HBV pathogenesis. HBV transgenic mice were instrumental to determine the role of HBV-specific T cells in viral control through non-cytopathic mechanisms and to analyze the importance of other cellular components of the immune system like macrophages, chemokines, and platelets in liver damage [7, 8]. Studies in HBV mouse model established by hydrodynamic transfection of replication competent HBV genome have also clarified the role of different cellular components of the immune system (CD8T, CD4T, NK cells) [9, 10], while HBV chimeric mouse reconstituted with human hepatocytes were instrumental in determining innate immune activation in HBV infected hepatocytes [11] and to test new therapeutic strategies [12].
These models, however, cannot completely recapitulate the virological and immunological events of the natural infection. HBV transgenic mice are generated through microinjection of HBV-DNA (a partial or complete genome) into fertilized murine eggs (allowing chromosomal integration of viral DNA into host genome) and subsequent implantation of the eggs into pseudo-pregnant female mice [13, 14]. The usage of the HBV endogenous or liver-specific promoter allows hepatocytes to express viral proteins, ensuring HBV transgenic mice to express either a particular viral antigen or the complete HBV genome within the hepatocytes. Nevertheless, HBV does not directly infect murine hepatocytes, thus a true infection does not occur in the HBV transgenic mice even with the presence of circulating virus [15]. Mice transgenic for hNTCP, the receptor of HBV entry, were engineered but these animals are still not susceptible to HBV infection as cccDNA formation seems to be a blocking step in this species [16]. Second, HBV transgenic mice are immunologically tolerant to viral antigens [17]. Hence, the original transgenic model does not display any classical induction of antiviral immunity and also sign of chronic liver disease. The immunological tolerance physiologically present in these mice is bypassed through adoptive transfer of syngeneic, unprimed splenocytes [18] or adoptive transfer of HBsAg-specific CD8+ T cells into thymectomized, irradiated, and bone marrow-reconstituted HBV transgenic mice [19]. This adoptive transfer creates a model of immune mediated chronic hepatitis, but different from the natural chronic hepatitis B infection. The immune cells transferred in the HBV transgenic mice are acting in a co mpletely normal liver environment, and as such the possible influence of the liver microenvironment affected by a chronic inflammation cannot be evaluated. Last, HBV covalently closed circular DNA (cccDNA), the primary viral transcriptional template during infection, is not found in the whole genome HBV-replicating transgenic mice [20] Hence, host attempts to clear HBV cccDNA or the role of HBV cccDNA in HBV persistence cannot be studied.
Similar limitations are also present in HBV hydrodynamic transfected mouse. Unlike transgenic mice where the hepatic expression of the HBV genome is controlled primarily by either endogenous (HBV) or liver-specific promoters, and is already present at birth, the systemic administration of the HBV plasmid under hydrodynamic conditions preferentially, but not exclusively, delivers the HBV transgene into the hepatocytes [9]. Integration of the HBV genome is then mediated through the action of the inverted terminal repeats of adeno-associated virus or by the Sleeping Beauty transposon system, while expression is controlled by the endogenous or liver-specific promoters [21]. Hence, this technique provides a relatively simple and convenient method to produce mice with HBV-expressing hepatocytes for the analysis of viral dynamics and anti-HBV immune response. Indeed, since HBV-expression is not present at birth these mice are not tolerant to HBV antigens and they can mount an immune response against HBV.
Nevertheless, in addition to the fact that these mice are not permissive for HBV infection (virions are produced from the transfected hepatocytes but cannot reinfect the mouse hepatocytes), the technique is only partially specific for the liver, often resulting in off-target transfections. How these unintended transfections of other cells could affect the viral kinetics and antiviral immunity found in this model is difficult to predict. Furthermore, hydrodynamic transfection typically reaches peak transgene expression after approximately 8 h post injection of plasmid DNA and expression levels decreases thereafter [9, 10]. This transient transfection property of the technique precludes the generation of persistently HBV transfected mice without additional manipulations and is not mimicking the kinetics of viral replication of the natural infection.
Figure 8.2 depicts the differences in the kinetics of viral replication and antiviral immunity between natural infection in human (and chimpanzees), HBV-tran sgenic mice and hydrodynamically transfected mice. The limitation of using such models to study viral replication kinetics and immune response after primary infection is highlighted.


Fig. 8.2
Comparative HBV kinetic in human/chimpanzee (a) and hydrodynamic HBV Mouse models (b)
The human chimeric mouse represents a further advancement of HBV mouse models. In these mice, xenotransplantation of human hepatocytes progressively repopulate the mouse liver, with the goal of replacing the entire mouse organ with functional human hepatocytes. Different from the transfection mouse models where HBV virions were produced artificially without infection, these chimeric mice were fully permissive for HBV infection, creating a reproducible small animal model of true HBV infection and replication [22, 23]. This combination of bona fide HBV infection in a mouse model provides, at the moment, the most physiologically relevant platform for the analysis of human HBV infection, virology and antiviral testing. However, due to the immunodeficient nature of the model, the analysis of HBV immunopathogenesis is restricted. The technical difficulties in standardizing the number of grafted human hepatocytes and the limited availability of human hepatocytes represent significant constraints for the production of this model. A further recent development is a human chimeric mice reconstituted with fetal human hepatocytes [24]. These chimeric mice are repopulated not only with human hepatocytes but also with human immune cells (monocytes, T and NK cells) with identical genetic background. Whether such immune cells fully reconstitute a normal immune system needs to be carefully evaluated but certainly this model might represent a great advantage for the study of the anti-HBV host immunity. Recent work using this model have shown that liver chronic inflammation can be induced after HBV infection while the ability to induce a HBV-specific adaptive immuni ty seems to be greatly compromised [24]. Improvements of this model are likely needed to fully recapitulate the immune features induced by HBV in infected patients.
In Vitro Models of HBV Infection: What Do They Mimic?
Human hepatocytes are the natural target cells of HBV and HDV. These cells can be isolated from liver resections and retain susceptibility to HBV infection for a short period in culture [25]. However, the accessibility to fresh human liver resections, the quality and the variability of the individual preparations limit their use. In the mid-1990s, several laboratories showed that primary hepatocytes of Tupaia belangeri were also susceptible to HBV infection [26, 27]. Although primary tupaia hepatocytes are valuable to study HBV infection, the difficulty to rear these animals, and the absence of tupaia-specific reagents for functional studies limit their use. To bypass the hurdles to using primary cell cultures, human hepatoma Huh7 and HepG2 cell lines were used for many years to perform in vitro experiments on HBV. Although these cells are permissive to HBV replication and viral particle assembly, they are not susceptible to infection due to the lack of expression of the receptor (s) and thus only allow study of post-transcriptional steps of the HBV life cycle after plasmid transfection. Since the recent discovery of sodium taurocholate co-transporting polypeptide (NTCP) as a HBV/HDV receptor [28, 29], HepG2 and Huh7 cell lines (over)-expressing NTCP have been generated. These cells are susceptible to HBV and HDV infections but their capacity to allow virus propagation remains to be determined. Because of their transformed nature, their relevance for studies of virus–host cell interactions has to be considered with caution. Alternatively, the HepaRG cell line can be used for in vitro studies. HepaRG cells are liver progenitors that become susceptible to HBV and HDV infection after differentiation in culture [30]. However, infection rates are low and virus spread in cultured cells is not observed. Finally, a recent study showed that micropatterning and coculturing (MPCC format) of primary human hepatocytes or hepatocyte-like cells differentiated from induced-pluripotent stem cells (iPSC) with fibroblasts maintains prolonged HBV infection [31], a model eventually amenable to study virus–host interactions and antiviral drugs affecting early infection steps.
Several aspects should be discussed regarding the biological relevance of these study models. Besides the susceptibility to infection and replication, the propagation of the virus in the cell culture seems to be limited. Although these cells are overall susceptible to HBV infection, this limitation in the capacity to disseminate to other cultured cells might be linked to several factors including the low yield of HBV production from infected cells, the restriction of infection by innate responses of hepatocytes to HBV infection, and the fact that hepatocytes may de-differentiate with time in culture. Furthermore, these models are quite static as the most physiologic systems rely on nondividing differentiated cells limiting the possibility to generate a dynamic model in which daughter cells resulting from cell division could be infected.
Another important parameter is the capacity of these cells to support cccDNA formation, amplification and recycling. Indeed, a system allowing a dynamic formation of cccDNA is highly desirable for the identification of host cell factors involved in its formation, chromatinization, and stability, as well as for the study of the mechanism of selection of escape mutants which involves at least the spread of the mutants to susceptible cells and the establishment of a pool of mutant cccDNA. Unfortunately, the levels of HBV cccDNA in infected cultured hepatocytes remain low (approx. one copy per cell), and are consistently lower than that obtained with DHBV whether it is cultured in chicken or human hepatoma cells [32].
The host cell response to infection is also an important aspect that is amenable to experimental studies in cultured hepatocytes. Regarding innate responses of hepatocytes to HBV infection, primary human hepatocytes and HepaRG cells are capable of mounting interferon responses that can limit the rate of viral replication and the spread of the virus [31, 33]. In contrast, the transformed HepG2 cells exhibit a blunted activation of IFN signaling pathways allowing the persistence of high levels of viral replication [33]. These are important differences between these cell types that should be taken into consideration when performing studies of hepatocyte response to HBV infectio n (Table 8.1).
Table 8.1
Human cells for HBV study in vitro
Immortalization | Transformation | Availability | Variability | Rate of infection | DMSO for infection | cccDNA levelsa | HBV propagation | Innate immunity | Maintenance | |
---|---|---|---|---|---|---|---|---|---|---|
Primary human hepatocytes | − | − | + | +++ | 20–100 % | 1.8–2 % | 1–2 copies per nuclei | − | +++ | 2–3 weeks |
Differentiated HepaRG cell line | + | − | +++ | ++ | 5–20 % | 1.8–2 % | 0.2–0.5 copies per nuclei | − | +++ | >6 months |
HepG2/Huh7 cell lines | + | + | +++ | + | 0 % | 0 % | − | − | − | − |
NTCP-HepG2 cell line | + | + | +++ | + | 50–100 % | 2.5–3.5 % | 1–5 copies per nuclei | − | − | 10 days |
The Importance of Clinical Samples in the Study of HBV Infection
We have summarized the features of in vivo and in vitro models of HBV infection. We will now discuss how analysis of clinical samples can help in addressing questions related to HBV infection and immunity.
Acute Versus Chronic Infection of Hepatocytes
The study of HBV biology in hepatocyte cultures is limited particularly in relation to the role of hepatocyte turnover. The access to liver samples of patients or from relevant animal models remains critical.
The outcomes of HBV infection are highly dependent on interactions between the virus and the host immune system. Indeed, whereas 95 % of immuno-competent adults clear the infection, only 5–10 % of children are able to do so. Infection of hepatocytes is thought to be non-cytopathic in the short-term. As hepatocytes are long lived (half-life of ~6 months) and have self-renewing properties, the liver loses genetic complexity over time. By increasing cell death and subsequent hepatocyte regeneration, hepatitis increases this loss of complexity and increases hepatocyte clonality. Chronic infection will thus have a major impact on complexity loss by raising the daily rate of hepatocyte turnover. The loss of hepatocyte complexity and/or the increase of DNA damage in proliferating hepatocytes could trigger hepatocyte transformation and tumor development. Transient infection causes one liver turnover, and does not have a significant impact in the long-term. It is also interesting to see that in chronically infected individuals, clonal expansion of hepatocytes not expressing viral antigens is observed. This may result from the survival advantage of these infected cells generated by liver turnover in the context of chronic immune killing. This clonal expansion may represent an important factor involved in liver tumor development [34–36] and this phenomenon cannot be mimicked in vitro or in an in vivo model. This is why the study of HBV pathobiology in liver samples from infected patients at different phases of the infection remains cru cial.
The Quantity of HBV Infected Hepatocytes
Many clinically relevant questions regarding the number of infected hepatocytes remain unresolved. One important question is whether all hepatocytes are equally infectable. Observations from immunostaining of liver samples from chronically infected patients often show a high number of cells stained for HBsAg (usually >50 %), but a much lower number of hepatocytes stained for HBcAg (usually <50 %) [37]. It is not clear whether this discrepancy is due to a differential expression of these viral proteins, to differences in trafficking in the infected cell or to methodological issues in the detection of these proteins.
The number of hepatocytes stained for HBV antigens depends also on the phase of the disease, with a much higher number in the so-called immune tolerant phase and a progressive decline through the immunoactive phase and the inactive carrier stage. In UPA-SCID mice or FRG mice with a humanized hepatocyte population, the number of hepatocytes stained for both viral antigens is usually consistent and >90 % [38]. This mainly reflects the situation of an acute infection in a host harboring defective immune responses. In chronically infected patients, the immune response (1) either by cell killing and hepatocyte turnover, or (2) by repressing the expression of viral proteins in a non-cytolytic manner, may contribute to a decreased number of cells expressing viral antigens. In the first scenario, the number of cells harboring cccDNA would be decreased, while in the second scenario the number of infected cells and copy number of cccDNA could be more or less stable. Only few studies have addressed the issue of the kinetics of cccDNA evolution over time during the natural or treated history of infection. Currently, the quantitative PCR based assays that have been developed to quantify cccDNA report results on a bulk of liver cells present in the liver samples (ranging from 0.01 copy/cell to approximately 1–5 copy/cell depending on the level of viral load and the phase of the infection) [35]. In the case of high HBV replication levels, it is not yet clear if all hepatocytes harbor cccDNA or if a small fraction of these cells is free of this viral DNA form. From studies performed in animal models (woodchuck and humanized mice), it seems that cccDNA is lost through cell division due to dilution by unequal transmission to daughter cells and that cell turnover is required for an active elimination of hepatocytes harboring cccDNA [38, 39].
The adult liver is thought to be composed of approx. 5 × 1011 hepatocytes; it is interesting to see that in the early non-inflammatory phase of the infection, viral titers can be as high as 1011 virus copies/mL suggesting that the multiplicity of infection is much lower in vivo than in cell culture experiments, an observation that was confirmed after experimental inoculation of animals (chimpanzee, woodchucks or ducks) [40]. This again highlights the lack of virus propagation in cell culture, whose reason remains unclear but may be related to additional factors present in the liver microenvironment.
Other clinically meaningful questions concern the infectivity and replication capacity of the different HBV genotypes and the main circulating mutants (pre-core mutants, antiviral drug resistant mutants, and vaccine escape mutants), i.e., the possible role of HBV genetic variability. The evaluation of viral load in patients infected with these different strains reflects many other factors including duration of infection, the host immune responses, the number of remaining hepatocytes susceptible to infection in a damaged liver. Only few studies have looked at the true infectivity of these viral strains either in cultured hepatocytes (HepaRG cells or primary human hepatocytes) or in animal models (chimpanzees or liver-humanized mice) [41, 42].
The study of the viral fitness of escape mutants or viral genotypes has also been hampered by the lack of easy in vitro or in vivo system to study competition between viral species and remains challenging compared to other virus models where the dynamic of infection is much higher (i.e., HCV or HIV).
Assessment of cccDNA in View of New HBV Cure Strategies
Methodological Issue s
Clearance of cccDNA will be the major treatment endpoint for a HBV cure, either using silencing or degradation strategies [43]. Thus, it will be critical to develop new sensitive and robust methodologies to assess in a quantitative manner (1) the number of infected cells harboring episomal viral DNA versus hepatocytes harboring only integrated viral sequences (witness of a past infection of the parental cells or of cccDNA cure in a non-cytolytic manner), (2) the number of cells expressing viral antigens, and (3) the quantitative determination of the epigenetic status of cccDNA in infected cells. These new technologies may require the establishment of in situ hybridization/PCR methods to detect and visualize cccDNA in hepatocytes, as well as methods to analyze chromatin-bound cccDNA in cultured or fresh liver samples. However, the access to human liver biopsies is getting more difficult due to ethical considerations and implementation of noninvasive methods of liver fibrosis assessment. Thus, the development of fine needle aspiration technologies linked to highly sensitive metho ds to analyze HBV in a few hepatocytes with single cell PCR methods is warranted.
Anti-cccDNA Strategies: Experimental Issues
Physical elimination of cccDNA harboring cells can occur by specific cytotoxic T cell responses, as demonstrated by resolutio n of acute infections in the chimpanzee [44]. Nevertheless, this immune response is impaired in chronically infected patients [45, 46], hence putting little immune pressure on infected hepatocytes.
When viral eradication is not achievable, lowering of liver cccDNA levels and/or inactivation of cccDNA directed transcription to prevent viral replication and remission of liver disease could be a realistic endpoint. Identification of cccDNA-free woodchuck hepatocytes containing traces of the infection in form of viral integrations indicated that cccDNA clearance may occur without killing the infected cells. This could be achieved mainly by two mechanisms: (1) “dilution effect”: since cccDNA is not replicated along with the host genome, cccDNA-free cells could arise through multiple rounds of cell division and unequal partitioning of cccDNA molecules into daughter cells [47, 48]. Notably, studies in the duck model showed that antiviral therapy with polymerase inhibitors induced a greater cccDNA reduction in animals displaying higher hepatocyte proliferation rates [49]. Inducing hepatocyte death and division would not be an easily controllable phenomenon in view of clinical application. Moreover, it has been demonstrated that very low levels of cccDNA can persist indefinitely in few liver cells even after the resolution of an acute infection [50]; (2) “targeted cccDNA degradation”: the recent discovery that IFN-α and lymphotoxin-β are capable of inducing a partial non-cytolytic degradation of the cccDNA pool through cytidine deamination in vitro invokes the possibility to cure HBV infected cells via pharmacological activation or triggering of host antiviral pathway [51]. It remains debated whether this APOBEC3A/B mediated degradation of viral DNA occurred on a single stranded DNA or on true cccDNA [52]. Similarly, DNA cleavage enzymes, including homing endonucleases or meganucleases, zinc-finger nucleases (ZFNs), TAL effector nucleases (TALENs), and CRISPR-associated system 9 (Cas9) proteins [53], specifically targeting the cccDNA are currently being engineered. These enzymes are clearly working in vitro [53, 54], but need further demonstration of their efficacy in vivo.
Interfering with cccDNA-associated chromatin proteins might be another exciting approach to achieve HBsAg loss. Indeed, the acetylation and/or methylation status of the histones bound to cccDNA affect its transcriptional activity and, consequently, HBV replicative capacity [55]. In cell culture and in humanized mice, IFN-α administration induces cccDNA-bound histone hypoacetylation, as well as active recruitment of transcriptional co-repressors on the viral minichromosome [55]. This may represent a molecular mechanism whereby IFN-α mediates epigenetic repression of cccDNA transcriptional activity, which may assist in the discovery of novel therapeutics.
The Role of the Liver Microenvironment in HBV Infection and Immune Pathogenesis
Another important issue that affect in vitro and in vivo models of HBV infection is the role of the liver microenvironment during the early phase of infection to control the outcome of infection, i.e., resolution versus chronicity, as well as its role in the pathogenesis of chronic infection [56, 57]. This type of questions is difficult to address both in tissue culture and with liver samples from infected patients or animals. Experiments performed in tissue culture can identify the potential role of individual cell types of the microenvironment, but the reconstitution of the liver lobule architecture with all the immune cells residing in the liver remains a challenge. Studies performed with liver samples from infected patients or animals can benefit from improved cell sorting technologies to isolate liver cells and perform functional studies. These studies remain limited by the difficulty to study sequential events in the same patient, while in animal models the impact of human/animal chimera in the liver or the host specificity is difficult to handle.
When HBV enters the liver, it is confronted with many different cell types. Indeed, the liver is a complex and structured organ that contains hepatocytes (parenchymal cells), non-parenchymal cells such as liver sinusoidal endothelial cells (LSEC), stellate cells and numerous resident immune cells, including Kupffer cells (KC), dendritic cells (DCs), NK/NKT, CD4+ T cells, CD8+ T cells, regulatory T cells (Treg), B cells [56, 58] These cells are organized according to a very particular and unique architecture. The importance of the liver microenvironment is often underestimated, and one should be cautious with in vitro experiments using hepatocytes that may behave differently when studied outside this microenvironm ent. Similar consideration can be done for anti-HBV immunity study (Fig. 8.2).
For example, we have already discussed that most of the detailed knowledge of intrahepatic HBV-specific T cell function derived from studies performed in mice with a normal liver environment, However, IL-10 [59], TGF-β [60], and arginase [61] are elevated in chronic HBV infections and such inhibitory cytokines impair T and NK functions. In addition, the composition of immune cells in pathological and normal livers is also altered in patients [62]. Thus, the function of immune cells in normal or pathological liver environment can differ.
The ability of cytokines to inhibit HBV replication in infected hepatocytes can also be influenced by the pathological liver microenvironment. IFN-alpha and IFN-gamma inhibit HBV replication in cell culture and in vivo models, but again most of the experiments performed to measure the ability of cytokines to inhibit HBV replication have been performed in experimental systems devoid of chronic inflammatory events [55, 63]. However, intrahepatic levels of SOCS3, a negative regulator of cytokine signaling and a predictor of poor IFN-alpha therapy response in HCV patients [64] are increased in patients and woodchucks with chronic hepadnavirus infections [65, 66].
Age and HBV Infection
The development of chronic hepatitis B (CHB) is inextricably linked to the patient’s age at the time of infection. HBV is thought to exploit the immaturity of the neonatal immune system to establish a persistent infection, reflected in the 90 % of neonates who develop chronicity following perinatal transmission. On the contrary, acute hepatitis B infection in adults is almost invariably associated with control of HBV infection through the induction of an efficient HBV-specific T and B cell response [1]. HBV infection in infants or young children rarely causes acute hepatitis and results in the asymptomatic disease phase characterized by high levels of HBV replication and a low incidence of liver inflammation defined as immune tolerant [67]. To explain this dichotomy, data from experimental animal models (i.e., HBV transgenic animals) have described the presence of immunological defects which impair HBV-specific T- and B-cell priming in neonatal animals [68–70] that could cause HBV persistence. However, it is important again to consider that since HBV does not infect murine hepatocytes, these animal studies can only partially imitate the events occurring during natural vertical HBV infection.
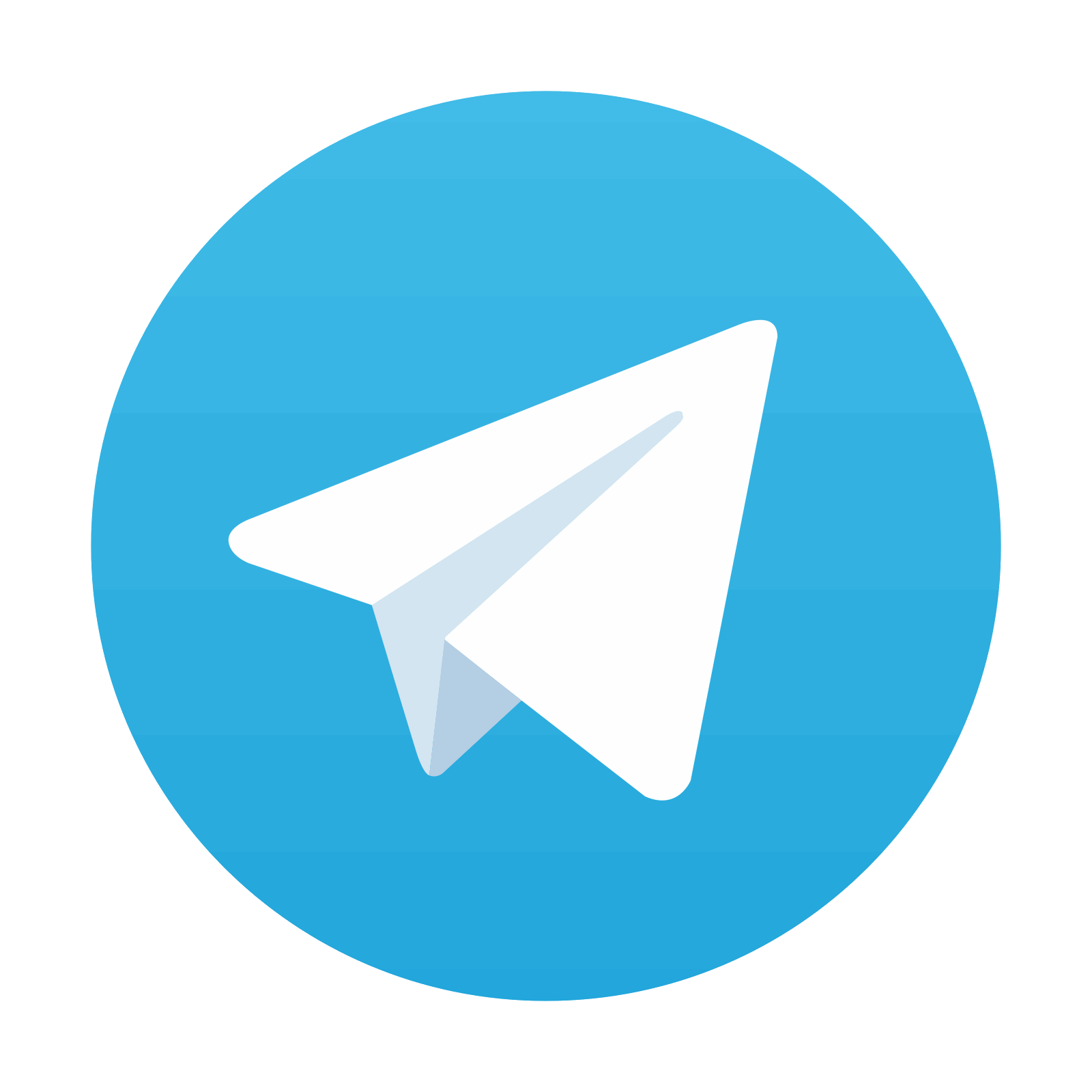
Stay updated, free articles. Join our Telegram channel

Full access? Get Clinical Tree
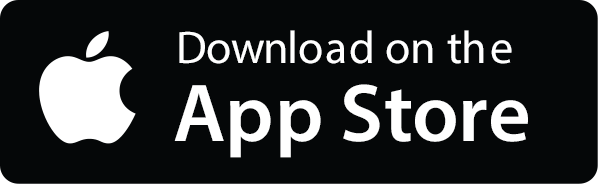
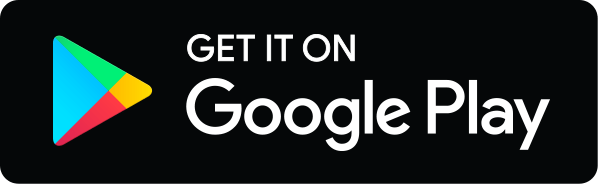