Jonathan Cohen1 and David A. Greenwald2,3 1 New York University Grossman School of Medicine, New York, NY, USA 2 Mount Sinai Hospital, New York, NY, USA 3 Icahn School of Medicine at Mount Sinai, New York, NY, USA Gastrointestinal endoscopy has grown increasingly more complex as the field has evolved over the past several decades, now requiring the practitioner to become proficient at many techniques. To perform high‐quality care, endoscopists often have had to devote time to learn new techniques as well as take care to continually maintain existing skills. As the technology and applications have progressed, so too have the methods by which individuals have learned to perform these procedures. In this chapter, we will trace the evolution in training from the self‐taught pioneers of the early days to the advent of formal proctored tutelage that remains the mainstay of training in this field. The chapter will also relate the emergence of numerous innovative learning tools that have already served to further transform training in gastrointestinal endoscopy. In particular, we will describe the development of simulator‐based instruction from the creation of realistic models to their validation and growing importance in endoscopic training. Lastly, we will address a number of novel principles of education in endoscopy that have paralleled the growing availability of these new teaching tools. Self‐training for gastrointestinal procedures was the mode by which many of the early endoscopists progressed, largely because devices and equipment became available for which there was no “expert” instruction. In general, this method is not appropriate any longer for training in standard procedures (i.e., colonoscopy, upper endoscopy) where sufficient proctoring is readily available. However, as newer techniques are introduced (i.e., endoscopic suturing, endoscopic mucosal resection (EMR), endoscopic sub‐mucosal dissection (ESD), stent placement, transluminal surgery), the question of how to satisfactorily teach these new skills becomes relevant [1]. In fact, “short courses” have been developed to review the cognitive and technical aspects associated with such procedures. American Society for Gastrointestinal Endoscopy (ASGE) guidelines concerning such “short courses” exist, and suggest them as a possible way for experienced endoscopists to acquire new skills, but reject such methods for initial training for “standard” endoscopic techniques such as colonoscopy, upper endoscopy, endoscopic retrograde cholangiopancreatography (ERCP), and endoscopic ultrasound (EUS) [2]. The need to impart the wisdom from the growing expertise with endoscopes was readily apparent to the pioneer generation of flexible fiberoptic endoscopy. As early as 1962, the then recently renamed ASGE conducted a symposium entitled “Teaching Methods in Gastrointestinal Endoscopy” in New York City [3]. Two years later, the ASGE formed a committee to examine the requirements for training endoscopists; the conclusions established training as a priority and created a framework that guided formal endoscopy training for many years to follow. Three items were required: (1) full training in medicine or surgery, (2) special training specifically in gastrointestinal (GI) endoscopy under the supervision of an appropriately skilled teacher, and (3) performance of an adequate number of procedures. Soon to follow was the first annual postgraduate training course. These efforts at a national level have been complemented by a proliferation of local and regional efforts to promote training with local courses and lectures aimed to supplement the one‐on‐one supervised instruction of trainees in the endoscopy laboratory as well as keep practicing endoscopists up on all of the latest techniques and advances. In 1973, Jim Eddy, Jerry Waye, Hiromi Shinya, Sid Winawer, Paul Sherlock, Henry Colcher, David Zimmon, and Richard McCray met at the Yale Club in New York to discuss how they might disseminate their knowledge and excitement about colonoscopy and polypectomy to practicing gastroenterologists. The result was the formation of the New York Society for Gastrointestinal Endoscopy (NYSGE) and shortly thereafter, an annual endoscopy course initially designated, “A Day in the Colon.” In this case, a regional society was founded for the sole purpose of promoting training. The evolving role of societies in training is the subject of Chapter 38 in this book. However, it is important to recognize that from the national to the local level, the endoscopic societies have provided the dedication, organization, and resources to innovate and advance the field of training. Supervised performance of actual endoscopies remains the predominant mode of endoscopy education today. Such apprenticeship‐type relationships between mentor and mentee have evolved greatly from the autocratic and unidirectional flow of information characteristic of similar learning environments dating back to the Middle Ages (Figure 1.1). Recognition and adoption of key concepts such as the benefits of learning in a reduced stress environment, the need for constructive feedback and interactive dialog, and the importance of gradually increasing autonomy of the trainee as skills progress are among the concepts that would make current trainee learning environments quite foreign to medical apprentices of earlier eras. In the United States today, most instruction in the techniques of gastrointestinal endoscopy is accomplished in the setting of formalized training programs of 3 years duration, with additional training available for selected “advanced” procedures such as ERCP and EUS. Proctored teaching of endoscopic techniques within such highly structured environments has been the “traditional” training method in gastrointestinal endoscopy. Endoscopic skills are developed concurrently with the immersion of the trainee in a complete curriculum that encompasses the range of normal and abnormal functioning of the digestive system, GI anatomy, and pathology. Trainees learn the indications for endoscopy, diagnostic and therapeutic capabilities of endoscopy, technical endoscopic skills, and application of therapeutic endoscopic intervention all in the context of intensive active supervised participation in consultative gastroenterology, for both outpatients and hospitalized individuals. While many physical aspects of endoscope manipulation and even lesion recognition can be taught to individuals not versed in the science and art of caring for patients with gastrointestinal complaints and disorders, to date, patients and practitioners alike have recognized the value and requirement that endoscopy be performed by individuals trained in such a comprehensive fashion, something that in this day can only be achieved in formal gastroenterology and surgical training programs. For this reason, this remains a first principle of published ASGE training guidelines [4, 5]. Figure 1.1 An example of a typical apprenticeship contract in colonial America, circa 1750. Within these training programs, didactic information about endoscopy is included in the curriculum to an extent, but much of the actual endoscopic training remains directly imparted from instructor to student in the course of the performance of actual procedures on actual patients. Such hands‐on supervision allows for increasing independence on the part of the trainee, as the teacher constantly assesses both technical and cognitive progress [6, 7]. In this process, the endoscopy teacher must give the trainee sufficient time to develop skills while protecting the patient’s safety at all times, and must be able to give appropriate feedback [8, 9]. This process is both time and labor intensive. Additionally, sufficient case volume is necessary to allow for development of necessary skills through repetitive deliberate practice, and enough variation in pathology needs to be present to allow the development of cognitive skills to go along with advances in technical expertise [10, 11]. Mere possession of clinical judgment and endoscopic proficiency do not guarantee that an individual is qualified to be a good endoscopic teacher. The importance of having instructors who know how to teach and the constraints that limit the time such mentors have to devote to teaching can pose significant challenges for this “traditional method” of endoscopy training—challenges which some of the newer complementary teaching tools discussed later in this chapter were developed to address. While many of the chapters in this book refer to the importance and characteristics of good mentors, very little investigation has yet been conducted to understand how to best train the trainers to teach endoscopy. This topic is addressed in detail in Chapter 4. Guidelines for training in gastrointestinal endoscopy have been published and widely disseminated [4, 5]. Skill sets that trainees must acquire to successfully perform endoscopic procedures have been outlined [12–16] and include the following: Since the early establishment that training in endoscopy was a high‐priority activity among academic endoscopy centers and GI societies, a great deal of effort has been devoted to assess the efficacy of training, determine learning curves for various procedures, and explore new methods for imparting proficiency. A number of important guidelines on the subject have incorporated much of this data and expert opinion on the subject [5]. One large review delved into the data for each procedure in great detail [16]. Specific chapters in this volume address training as it relates to quality in endoscopy and to specific standards for each of the major endoscopic procedures that are performed. Apart from the specific recommendations about learning particular procedures, a number of themes have emerged throughout all current guidelines, which reflect the evolution of the concepts of optimal training in endoscopy. Key principles include the following: Simulators have been proposed as a way to facilitate endoscopic training from the time of the earliest development of the field. In fact, Rudolf Schindler described using a model stomach for practice in orientation [17]. Many of the items in the “skill sets” listed above, and particularly those that involve dexterity, hand–eye coordination, and recognition of normal anatomy and abnormal pathology, can be addressed through the use of various endoscopic simulators. The initial attempts to complement endoscope training with simulators utilized static models. Such “phantoms” were intended to teach basic hand–eye coordination, the use of the endoscope dials, and even the recognition of basic pathology. In the 1970s, as upper endoscopy and colonoscopy were becoming established as important modalities, other models were developed. These included the Heinkel hemispheric anatomical model [18] and the upper GI plastic dummy introduced by Classen [19]. In the early 1970s, homemade demonstration models of the colon, featuring a mobile transverse colon and the ability to demonstrate an “N” or alpha loop, were devised (Figure 1.2). In 1972, a colonoscopy model fashioned from the spiral metal‐reinforced tubing of a hair dryer was introduced, with the ability to demonstrate corkscrewing movements (Figure 1.3). This early simulator featured the ability for the colonoscope to “become stuck” and then to be “straightened out.” Christopher Williams’ St. Marks/KeyMed colonoscopy model of 1975, shown in Figure 1.4, had an improved feeling of realism and was made commercially available. Twisting movements were required to negotiate the lumen, and endoscopists found it challenging [20]. Figure 1.2 Roller demonstration model (1971): Homemade model showing alpha loop and mobile transverse colon. (Courtesy: Dr. Christopher Williams.) Figure 1.3 Hair dryer tube model (1972). (Courtesy: Dr. Christopher Williams.) Simultaneously, hand–eye coordination models were developed. These included an electronic targeting model (Figure 1.5), which had a photocell at the center, tested two‐handed coordination, and allowed for “scoring” of results. An endoscopic version of the popular game “Pong” was even developed in 1977 (Figure 1.6), allowing for reinforcement of left/right coordination maneuvers in an enjoyable and motivating “game.” Figure 1.4 St Mark’s/KeyMed model (1975): Commercially available with semirealistic feel. (Courtesy: Dr. Christopher Williams.) Figure 1.5 Electronic targeting model (1975): Tested hand–eye coordination. (Courtesy: Dr. Christopher Williams.) The Imperial College/St Mark’s College Simulator was introduced in 1980 (Figure 1.7) and allowed for insertion of a limited amount of the shaft of an endoscope into the computer model, with real‐time video feedback. This model demonstrated that such devices were feasible, although the particular model was limited by the fragility of the microswitches. Improvements were made over the ensuing 5 years, and by 1985, an updated and substantially more robust version of that simulator existed. The MK2 simulator allowed full “shaft” insertion, a sensation of resistance during looping, and audio tracks to simulate patient “complaints” (Figure 1.8). The computer allowed for a database and record keeping. Still, the simulator was felt to be crude and somewhat unrealistic. Figure 1.6 Endoscopic Pong Game (1977): Tested hand–eye coordination. (Courtesy: Dr. Christopher Williams.) In 1992, Leung and Chung developed a static model and described its use in teaching ERCP [21]. Unfortunately, the utility of each of these models has been limited by their inability to truly simulate realistic conditions. To date, while these static learning devices can be useful in instruction and learning of appropriate manipulation of the endoscope within the bowel lumen, they offer little in the way of simulated pathology. The lack of motility, the “feel” of actual compliant tissue, and the inability to practice therapeutic maneuvers have largely limited the use of static models to introductory training. Perhaps, the most comprehensive application of static models in endoscopic teaching was described by Lucero et al. in 1995 [22]. This group designed a psychomotor training program called SimPrac‐EDF y VEE (simulator for the practice of fiberoptic digestive endoscopy and electronic video endoscopy). Moreover, they described a series of courses in which they included static models and superimposed painted pictures to recreate frequently seen endoscopic abnormalities. These courses featured didactic lessons, slides, tapes, and supervised hands‐on training on models. In addition to the Lucero model, those of Classen and Heinkel were also used. A specific Billroth II model was designed to demonstrate the unique features of this altered anatomy. Participants were offered sessions with increasingly challenging manual and cognitive tasks; faculty at the course assessed objective skills of the participants [22]. In Lucero’s courses, 8–25 individuals were included in each particular workshop, and trainees had a mean duration of hands‐on practice of 28 hours. In all, 422 trainees in over 22 such courses were described, and the authors noted that 95% of trainees demonstrated an “acceptable level of skill” by the end of the training [22]. However, the authors failed to describe other details of the possible benefits of such training. Such courses would appear to be difficult logistically to conduct and hugely labor intensive. Perhaps, the most important contribution of this work was the concept of integrating various hands‐on training tools into a comprehensive training program that combined didactic lesions, cognitive training, and specific hands‐on exercise geared to develop particular skill sets. Lucero’s use of a patterned lesson plan integrated into multimodality workshops using expert faculty, a blend of manual training and cognitive skills, and immediate feedback and evaluation served as a model for subsequent efforts using more realistic and sophisticated simulators. As such, it remains an important example for future endeavors in endoscopic training. Figure 1.7 Imperial College/St Mark’s simulator (1980): Limited shaft insertion, but feasibility of simulator demonstrated. (Courtesy: Dr. Christopher Williams.) Figure 1.8 Imperial College/St Mark’s simulator MK2 (1985): Full shaft insertion and audible “complaints.” (Courtesy: Dr. Christopher Williams.) Since that time, static models evolved in two distinct directions. Two ERCP static trainers were designed that allow for complex therapeutic procedure performance and team training between endoscopist and assistant. These are described in detail in Chapter 8 (Figure 1.9a,b). Figure 1.9 (a) Novel ERCP endotrainer introduced by Joseph Leung, MD that allows for simulated sphincterotomy and fluorosocpy equivalent. (b) Rome ERCP trainer designed by Dr. Guido Costamagna and colleagues allows for cannulation and endotherapy of bile and pancreatic ducts and ability to interchange papillae of different orientation and cannulation difficulty (Photo courtesy of Cook Medical, Winston‐Salem, NC). A further advance in endoscopic simulation was developed by Grund et al. at the University of Tübingen in Germany [24]. In this “Interphant” or “Phantom” model, artificial electrically conductive tissue called Artitex is used to fashion abnormalities such as polyps and strictures and incorporate this into static models. These “pathologies” are in place of the painted‐on abnormalities used in some of the pure static models mentioned above. Grund’s “Artitex” abnormalities are sewn directly into a three‐dimensional latex anatomical model (Figure 1.10a,b). While these models generally lack a realistic representation of bowel wall compliance and motility, the integrated pathology appears realistic and allows practice in electrosurgical techniques. In order to simulate the resistance to endoscope passage in an actual procedure, this colon model uses a semiflexible series of coils. In addition, to allow for a still wider possibility of simulated techniques, Grund’s model can incorporate real animal tissue into the existing framework. For example, using a chicken heart, they can fashion an ampulla of Vater replete with separate pancreatic and biliary orifices and insert this into their upper endoscopy simulator (Figure 1.10b). The advantage of using this type of system is that several “polyp‐laden” colons and “chicken‐heart papillae” can be prepared in advance and quickly inserted into the chassis of the model during a training session, after the initially prepared material has been depleted. The Tübingen simulators made possible the teaching of polypectomy and provided an excellent means of teaching therapeutic procedures such as argon plasma coagulation and simple therapeutic ERCP. In particular, the orientation of the man‐made papilla more closely resembled that of humans than the porcine papilla found in the Erlangen models described below. Pancreatic cannulation and endotherapy was possible, in contrast to the porcine tissue models in which the pancreatic orifice was not readily accessible. However, procedures that required submucosal injection were still not feasible. Figure 1.10 (a) Artificial tissue colonoscopy “Phantom” simulator, U. of Tübingen. (b) Combined artificial tissue “Phantom” upper GI simulator with integrated chicken heart tissue papilla for ERCP simulation. While this model represented a technological advance over prior static models and added many new capabilities, there remained several limitations that hindered its more widespread use in training. The main drawbacks were that the pathology remained hand‐prepared and that the models were not mass‐produced. Therefore, the “Phantoms” have not been readily available and have required the presence of the Tübingen team if the device was to be used at a training course. The trade‐off for increased realism and the ability to start practicing therapeutic manipulations were significant increases in the logistical and cost obstacles to widespread use. Furthermore, models combining the real tissue abnormalities of the Tübingen model with the more accessible ex vivo animal tissue simulators described below now exist. In 1996, Hochberger and Neuman created an innovative simulator using pig organs obtained from a slaughterhouse and fastened to a plastic platform [25, 26]. In order to create a model that would allow training and practice in therapeutic techniques, Hochberger then created a highly realistic simulation of pulsatile arterial bleeding (Figure 1.11a,b). This was accomplished by inserting tubes through the stomach, and sewing real arteries attached to a roller pump capable of pulsatile perfusion with a cherry‐colored saline solution. Following this, Hochberger developed representations of other pathologies for this model, including polyps, varices, and strictures [27, 28]. Currently, there are two basic model types based on these principles. The original Erlanger model features pig organs inserted into a dummy mannequin. This model has been used in the simulation of various laparoscopic surgical procedures [29]. Hochberger then created the compact‐EASIE model, a smaller portable, lightweight version using a tabletop platform. There are now several commercially available versions of this type of simulator in which only the organs needed for endoscopy simulation are secured to the platform plastic tray (Figure 1.11a,b). For example, only the esophagus to the duodenal bulb may be needed for a specific training session, but the model has the flexibility to allow the liver and hepatobiliary tree for an ERCP simulation involving fluoroscopy. Multiple therapeutic procedures may be demonstrated, taught, and evaluated on both of these animal tissue‐based simulators. With the advent of portable, compact tabletop ex vivo models that can easily be shipped to a location along with pre‐prepared frozen organ packages, some of the obstacles to simulator availability have diminished. Figure 1.11 (a) Compact‐EASIE porcine model hemostasis simulator. (b) Close‐up view of porcine stomach with arteries sutured in attached to catheters for hook‐up to tubing connecting vessels to pump. The trainee puts together a band ligation device for varices treatment simulation. Just as in the Lucero et al. experience described above, training sessions using the Erlanger or EASIE models are labor intensive, with high faculty‐to‐trainee ratios. Moreover, the advance work to organize and staff workshops using this technology is extensive. Since the compact EASIE is relatively portable, its use may allow easier access to animal simulator training at local sites, but this is counterbalanced by the need to have the required expertise to prepare the animal tissues and “load” the models. For this training technique to be applied widely, many expert teachers will still need to be trained; the feasibility of 1 day “train‐the‐trainer” courses has now been demonstrated [38]. On the other hand, like the static models before it, the EASIE model allows trainees to perform multiple repetitions of the same technique. The use of real tissue and the capability of performing advanced therapeutic procedures make this an attractive simulator for practitioners hoping to learn new techniques and for advanced fellows to practice and improve their skills, as well as to acquire new abilities [39]. By devising a way to allow realistic simulation of diagnostic and therapeutic techniques using a model that is portable, Hochberger set in motion a rapid expansion in the use of hands‐on training for GI fellows in the United States and Europe. Workshops at courses such as the annual NYSGE course and at national and regional endoscopy meetings proliferated. Around 2000, under the leadership of Christopher Gostout, the ASGE launched a major initiative to create opportunities for ex vivo model training in a central freestanding location. This effort culminated in the creation of the Integrated Technology and Training (ITT) center of the ASGE, and the development of a standardized first year fellows’ course curriculum, integrating a day of intensive hands‐on work in the ex vivo laboratory with interactive didactic lectures. The ongoing ASGE and industry support for these courses has led to over 300 fellows each year since 2005 attending the ITT workshop in the summer of their first year of fellowship. Through this effort, ex vivo hands‐on training to some degree has become part of the standard endoscopy training for US GI fellows. Efforts to extend such opportunities for advanced endoscopy trainees and to individuals in practice have expanded considerably since the mid 2000s and are addressed in Chapters 37 and 38 of this book. Just as important as his role in the development and popularization of the ex vivo model, Hochberger made several other key contributions to the evolution of endoscopy training. Expanding on the course concept of Lucero, he embedded the simulator work into rigorous training programs, which deconstructed instruction according to specific targeted skill sets. Regardless of the endoscopic procedure being taught, each learning station was assigned separate specific learning objectives. Manual hand–eye skills were taught using specially designed coordination exercises. Communication skills between endoscopist and assistant were emphasized. Assessment of skills was broken down by specific procedure steps to ensure that all details received specific attention and instruction. The structure of the workshops he designed in collaboration with his German colleagues and his colleagues from the NYSGE incorporated expert demonstration of proper technique, repetitive practice with sufficient endoscope time per trainee per skill station to do so, self‐assessment, and instructor feedback. These components have remained the essential backbone of all subsequent ex vivo model‐based training. In addition, Hochberger first promoted the concept of “team training,” focusing on the importance of coordinating training among the endoscopist and assisting staff. Although clearly advantageous for the above reasons, live animal courses also present some substantial drawbacks. Among these are that animals are very expensive to maintain and there are significant ethical considerations in using animals for training. These ethical considerations are magnified by the fact that ex vivo alternatives now exist for teaching most techniques and do not require sacrificing any animals solely for this purpose. In contrast to the multiple uses possible on other simulator types, once certain procedures, such as sphincterotomy, are performed, it is difficult or impossible for others to practice the same techniques on the same animal. For these and other reasons, training on live animals, while potentially more realistic than on inanimate simulators, appears now to be on the wane in the evolution of endoscopic training techniques. It appears likely that live animal courses will be limited to advanced procedures such as sphincter of Oddi manometry, for which no comparable inanimate model exists, and advanced training in ESD and NOTES®. For the latter techniques, many of the skill sets would still be best taught in inanimate tissue models, saving the live animal work for later training in which real physiological conditions and the potential for complication management is required. Live animal endoscopy laboratories remain well suited for clinical investigation. Finally, testing of new accessories and development of new techniques on live animals will likely continue, but much of the groundwork for these tests will have been already completed on inanimate simulators. Parallel to the introduction and adoption of ex vivo animal tissue models has been the development of increasingly sophisticated computer simulators. The technology has evolved to incorporate two main features, the ability to vary the pathology encountered and refinements of forced feedback or “haptics” to improve the realism of the earlier static models. A number of investigators have pioneered efforts to produce computer models, which can allow a realistic experience of handling the endoscope, and are also able to incorporate broad exposure to pathologic images [20, 43–53]. Because so many diverse images can be stored, computer simulation offers the best opportunity to expose trainees to a wide range of pathology. Computer‐based learning can take place either independently or as part of larger training courses, and progressive tutorials of increasing difficulty can be constructed. Unlimited repetition and drilling in specific infrequently encountered procedures is possible. Moreover, progress during training can be recorded and opportunities for feedback exist. Computer simulators typically utilize a “real” endoscope passed into a dummy mannequin. Tactile feedback capability, generated by sensors on the endoscope tip, is a key feature. The experience is enhanced by incorporation of real video images. Moreover, insufflation, suction, and bowel wall motility can be reproduced. An ASGE technology assessment statement on simulators describes in detail the innovative technological developments in this field [43]. The images on the display can be derived from interactive video stored on the computer or external storage devices, computer‐generated images, or a combination of both. The AccuTouch® endoscopy simulator (Immersion Medical) system (Figure 1.12) (http://www.immersion.com/products/medical/endoscopy.html) allows training in a number of procedures, including flexible sigmoidoscopy and colonoscopy, as well as bronchoscopy. It is possible to practice mucosal biopsy on this model. The simulator provides direct performance feedback to the trainee. A number of validation studies have been conducted using this simulator [53–56], which will be covered in detail in Chapters 5 and 6 of this book. The GI Mentor II (Simbionix) (Figure 1.13) (http://www.simbionix.com/GI_Mentor.html) offers several diagnostic and therapeutic modules [49]. Upper and lower endoscopy and ERCP are all performed on the same mannequin using a special endoscope for each procedure type. An accessory channel allows the endoscopist to perform a variety of therapeutic techniques, including biopsy, polypectomy, sclerotherapy, and electrocoagulation to control active bleeding, ERCP cannulation, and sphincterotomy. This simulator also includes some manual dexterity training exercises ideal for beginners to develop skills controlling the endoscope dials and using torque. The logical descendent of the Lucero model and progressive training program, the simulator incorporates a series of cases of varying pathology and technical difficulty. Instructors may delineate specific training programs. Trainees can get immediate feedback during and after completing each simulated procedure. In fact, the computer will even generate an expression of pain for overinsufflation or excessive looping of the instrument. Performance is recorded, including numbers and types of errors made. The instructor can review the progress of each trainee and the written procedure reports to determine whether abnormalities were correctly detected and identified; feedback messages may be sent back to the trainee. Figure 1.12 Immersion AccuTouch® colonoscopy simulator. Figure 1.13 GI Mentor II (Simbionix) colonoscopy virtual reality simulator. The GI Mentor II computer simulator has been incorporated into a number of European endoscopy courses, most notably in Scandinavia [57–59]. Respondents to questionnaires have expressed great satisfaction with the limited experience on the GI Mentor II simulator. As with the AccuTouch® simulator, a number of objective validation studies have been carried out for the GI Mentor II, and these data are presented in detail elsewhere in this book in Chapters 5 and 6. If computer simulators are to have a role in credentialing in addition to training, they must be able to distinguish between a novice and an accomplished endoscopist. A study from the Mayo Clinic demonstrated that performance parameters on the simulator vary according to real colonoscopy experience [56]. To date, however, no investigator has shown that a particular performance level measured on a computer or any other simulator is predictive of competent performance on subsequent real endoscopy. The Olympus colonoscopy simulator was a colonoscopy‐specific simulator based on advanced mathematical models developed in the late 2000s. Among its features, this model attempted to better simulate more difficult colonoscope passage [60, 61]. While this model was discontinued and is not commercially available, the rationale and performance characteristics to which it aspired remain critical to current simulator development and needs. For, it is enhanced realism and simulated procedure challenge that will be required to enhance simulator‐based training beyond the novice stage and to achieve reliable skills assessment on a simulator. At present, computer simulators appear to have much to offer trainees in terms of showing diverse pathology and teaching beginners hand–eye coordination and endoscope handling. Unique aspects of this type of training are simulation of contractions, feedback on comfort, opportunity for self‐instruction without constant expert supervision, quantification of skills, and offsite skills assessment by instructors. Current available models appear less useful for more experienced endoscopists, although capabilities are expanding rapidly. At present, the therapeutic modules for the GI Mentor II simulator are best suited for introductory orientation only to polypectomy, hemostasis, and ERCP. Computerized technology offers the potential to incorporate didactic lessons, specific questions for the endoscopist concerning accessory setup and generator settings, and opportunities for self‐assessment quizzes to complement the hands‐on technical experience. However, to date, such potential advances have not yet been incorporated into the existing simulators. The major obstacle to expanded use of these simulators remains the cost and logistics of making them accessible to trainees. At costs ranging from $50,000 to 70,000, most individual departments cannot afford to purchase computer simulators. What will be the next steps in the evolution of endoscopy simulators? A number of scenarios can be envisioned. Some potential developments envisioned in 2010 are described in Table 1.1, which interestingly shows ideas for future applications of simulators that were proposed in the first edition of this chapter, and now updated with remarks in red showing the progress or in some cases, lack of progress, in these areas over the past decade. Regardless of exactly how this field evolves in the coming years, it is fairly certain that simulators will play an increasing role in teaching and training in gastrointestinal endoscopy. On November 19 and 20, 2017, the ASGE hosted an EndoVators Summit, partially supported by grant from the National Institute of Diabetes and Digestive and Kidney Diseases of the National Institutes of Health at the ASGE Institute for Training and Technology in Downers Grove, Illinois. The purpose of the summit was to define the role and value of simulators in the future of endoscopic training and to reach consensus regarding priority areas for simulation‐related education and research and simulator development. Over 70 thought leaders in simulation research, simulator development, and endoscopic education and training and key decision makers from industry gathered to review the current state of endoscopic simulation and the role it could play in endoscopic training. A White Paper published in 2019 [62] summarized the day’s events and the findings. Table 1.1 Anticipated expanded applications of endoscopy simulation in 2010 and current status in 2020. The goal was to provide a working roadmap for the developers of simulators and for the investigators whose goal is it to define the optimal use of endoscopy‐related simulation. Moreover, the attendees emphasized the importance of assessing the impact of simulation on educational outcomes and health care quality, and the need to provide clear guidance to educators who seek to enhance integration of simulation into training and practice. While simulators are likely to play an increasingly important role in endoscopic training and retraining, key gaps were identified that must be addressed for simulation to have an even greater impact on endoscopic training. These include The past two decades have been characterized by rapid expansion of the training modalities at our disposal and the general acceptance of their use. Much work remains to clarify the optimal way to integrate these tools into standard training, training in advanced procedures, and in the uncharted waters of maintaining skills. A second major area for progress is in the area of creation and validation of simulator‐based skills assessments that predict performance level and competency on actual procedures. Simulator investigators have long realized that a key milestone would be the development of reliable simulator‐based assessments of competency. A third area that will need to be addressed in coming years is the further integration of some of these new teaching modalities into local programs. For example, the ideal follow‐up of the national first year’s fellows hands‐on training experience at the ITT would be follow‐up hands‐on workshops at various intervals run and funded locally with support of local physicians and industry. Funding and logistic issues need to be addressed, but adoption will first require increased acknowledgment by local program directors of the importance of such activities. For example, a combination didactic and hands‐on training course for advanced and interventional endoscopy held regionally may be an alternative to a centralized approach. To date, such workshops at professional society national and regional meetings (such as at ASGE, ACG, and NYSGE meetings) have emerged, but have not yet been scaled to reach the vast majority of GI trainees. Further expansion of hands‐on ex vivo simulator training at the local level will also require a considerable effort to train a broader group of trainers on how best to utilize these simulators to teach endoscopy [38] (see also Chapter 4). Finally, what about the introduction of new endoscopic technology and techniques? This would be another area of real promise for an expanded role of simulators and specific training program development. On the part of industry, this begins with the use of models to test early devices and procedures prior to more costly animal studies. Next is the growing recognition that innovative techniques require specific training programs to ensure both proper execution of the new procedures and acceptance of the innovation by practitioners. Busy clinicians will not adopt new skills unless an efficient and preferably validated training program is available to ensure that they can develop the proficiency to safely and effectively do the procedure. In contrast to the past when efforts to determine the best ways to train for techniques often came long after the procedure was adopted, in the future, there will be increasing pressure to address training upfront. One hopes that with this additional attention to training in parallel with technology development will come increasing avenues of support for simulator‐based training in general. This may be of particular importance as a partial solution to the problem of creating more opportunities for practicing endoscopists who desire to and will need to learn new techniques. The innovators will need to support those they hope will adopt the innovation; simulators are likely to facilitate this growing interdependence. It is clear that since 2000, there has been an increased focus on how endoscopic training should occur and on achieving benchmarks for assessing progress before further training should continue. Progress in simulation has been steady but remains slow, yet sentiment for engaging both trainees and experienced endoscopists for simulator training is evidenced by summits, White Papers, and continued innovation. Moreover, there continue to be progress in e‐learning, accelerated by the expanded use of video recording, and easier access to high‐quality material with distribution by broadband internet and advertisement and amplification on social media platforms. So where is endoscopic teaching likely to go? While the future is always hard to predict, certain things seem to be on the horizon. In general, one can predict increased training done by sharing of educational videos on platforms that are accessible worldwide. (see Table 1.2 for a summary of likely features of endoscopic training by 2030). These changes to video and remote learning were already moving into place, but the COVID‐19 pandemic of 2020 and beyond may have accelerated this development. Important driving factors here include: Table 1.2 A look at endoscopy training in 2030. Figure 1.14 Remote teaching of flexible endoscopy from New York to Kyabirwa, Uganda by Jerome D. Waye, MD, Professor Emeritus Icahn School of Medicine at Mount Sinai.
1
Training in Endoscopy: A Historical Background
Introduction
Standard training in endoscopy: then and now
What must be learned?
Standards and end points of current endoscopic training
Emergence of complementary teaching modalities
Why use simulators?
Endoscopy simulators, including ex vivo artificial tissue, animal tissue, and virtual reality computer‐based models, provide a unique method for endoscopic teaching. These devices allow for teaching which is free from the possibility of patient discomfort or injury. This factor alone confers several benefits to the learning process. First, the stress of the learning environment is reduced for the trainee and the trainer alike. There is more time for questions and feedback than available when an actual patient is involved. The issue of reduced trainee endoscope time due to critical clinical exigencies is eliminated, and there is ample opportunity for repetition. In fact, the sequence of demonstration of proper technique, repetitive practice of skills with expert feedback, and assessment of skill are all possible in this environment. Creative teaching exercises such as demonstrating common errors and what constitute poor technique are also uniquely possible using such alternative means of instruction to the traditional proctored human endoscopy setting for instruction (Video 1.1). In this way, simulators can confer excellent opportunities for “standard” techniques to be practiced by trainees and allow for new procedures to be taught to experienced clinicians [1]. To the extent that certain models might be used independently by trainees without real‐time instructor feedback, and to the extent that simulator work might hasten the time in which trainees can perform unsupervised procedures on their own, simulators also have the potential to address the time constraints facing endoscopy instructors with substantial nonteaching clinical responsibilities of their own to fulfill. However, as we will relate below, much of the actual effective learning using endoscopy simulators does require fairly labor‐intensive expert instruction, and to date, the potential for freeing up time spent mentoring trainees has not yet been realized.
Evolution and types of endoscopy simulators
Static models
In contrast, Dr. Christopher Thompson teamed up with MIT engineers to develop a static box model to allow for repetitive practice on five specific tasks designed to mimic component skill sets integral to performing colonoscopy [23]. In a departure from the paradigm to design sophisticated simulators to allow a trainee to practice complete procedures, the Thompson Endoscopic Skills Trainer (TEST) (Endosim, LLC. Boston, MA) is an example of using simulation to deconstruct complex procedures into core maneuvers (Video 1.2).
Ex vivo artificial tissue models: the “Phantom” Tübingen models
Ex vivo animal tissue simulators: Erlanger and EASIE models
While the most common application of the Hochberger model has been for hemostasis training, this group has conducted a number of training courses using the EASIE model in other areas, including EMR, stricture management, vital staining, polypectomy, and ERCP [28, 30–32]. A wide range of techniques can be demonstrated, including basic biliary cannulation, plastic stent insertion, choledochoscopy, laser lithotripsy, and placement of bilateral hilar metal stents (Videos 1.3 and 1.4). EASIE training has been shown to significantly improve technical skill in endoscopic hemostasis in gastroenterology trainees as compared to clinical endoscopy training alone [33]. As detailed in multiple chapters in this book, further adaptations of the ex vivo simulator have extended the use of this modality for training in colonoscopy, balloon‐assisted small bowel endoscopy, improved simulation of bile duct and pancreatic duct manipulation, EUS with FNA, and even NOTES® [34–37].
Live animal courses
Both research and training courses have employed endoscopy performed on live anesthetized pigs and dogs [39–42]. Using live animals provides the best possible tactile “feel” of real tissue and endoscope movements with conditions most closely resembling those that occur during human endoscopy. Specifically, this includes the presence of luminal fluid, motility, and the ability to cause real bleeding and perforation (Video 1.5). Such courses have been conducted to teach therapeutic techniques, most notably ERCP and EUS [41]. At present, live animal courses are the only means of nonhuman simulation of sphincter of Oddi manometry [42].
Computer simulation
Two commercially available computer simulators exist for EGD, colonoscopy, bronchoscopy, EUS, and ERCP, and a colonoscopy‐specific simulator was also developed but not commercially available (Video 1.6).
The future of simulators in endoscopy training
Ongoing evolution of endoscopic training
Several challenges exist as endoscopic training continues to evolve. To date, the vast capacity to incorporate the trove of stored video and photographic content covering endoscopically encountered pathology and how to manage it has been greatly under‐utilized. The DAVE Project [64] was launched in 2004 and represented a significant advance in allowing for free and easy access to view much of this kind of material (Video 1.7). Converted in 2013 to Apple podcasts, the DAVE Project stored annotated videos on an enormous range of common and uncommon findings, as well as demonstrations of typical and unusual procedures. Similar contact is now found in newer online platforms, including Video GIE (https://www.videogie.org). However, there is still very limited incorporation of this material into web‐based interactive learning opportunities. By taking advantage of broadband transmission and web‐based learning, cognitive training might undergo as great a transformation in the coming years as ex vivo models have provided for technical skills development.
A peek into the future of endoscopic training to 2030
The COVID‐19 pandemic has led to sharply increased interest and then sudden acceptance of video platforms that turn out to be easily accessible worldwide. Learning in remote parts of the world has been made simple and affordable. Rather than bringing equipment and personnel to remote parts of the world, technology allows for the teachers to both teach and supervise from afar. Indeed, despite being retired from active clinical practice, Dr. Jerome Waye, a true pioneer in endoscopy and colonoscopy and in its education, is again pioneering by leading this new wave of remote teaching and proctoring. In 2020, he was able to bring “video‐mentoring” to Uganda and other parts of the world (Figure 1.14) (Video 1.8). [See insert for a first‐hand description of this project by Dr. Waye.]
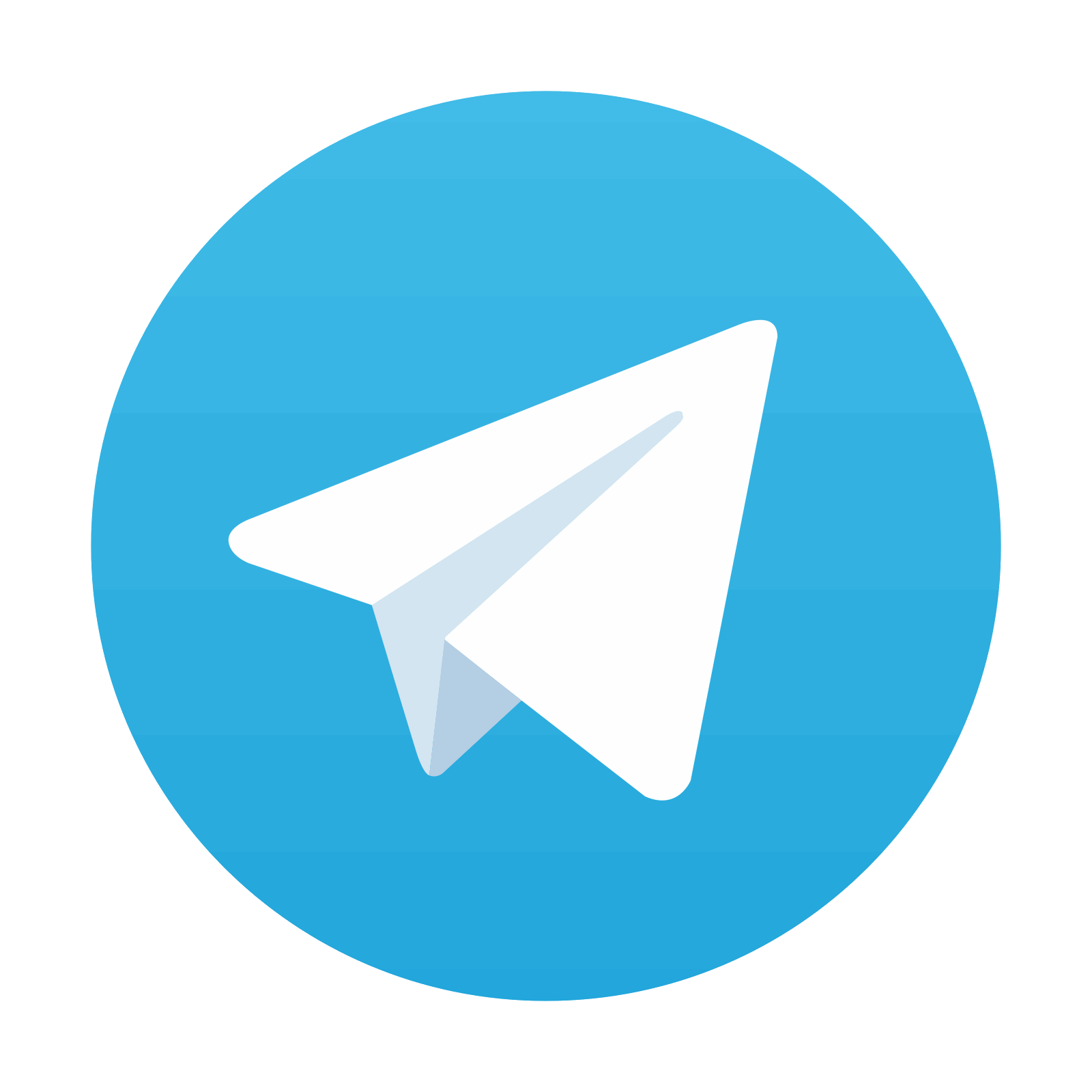
Stay updated, free articles. Join our Telegram channel

Full access? Get Clinical Tree
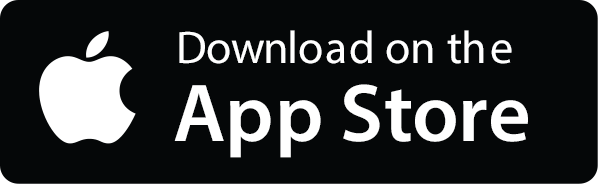
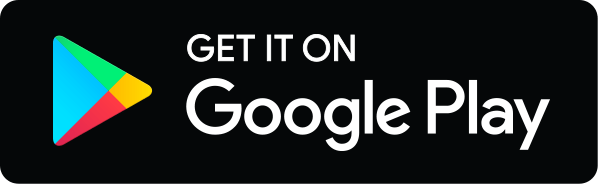