Keywords
Physiologic methodAnion gapBase excess methodPhysicochemical methodpCO2 SIDAcid–base disorders are extremely common in critically ill patients. They carry increased morbidity and mortality. Therefore, accurate assessment and appropriate therapeutic intervention are necessary to prevent these associated complications.
- 1.
Physiologic (traditional or bicarbonate-based) method
- 2.
Base excess method
- 3.
Physicochemical (Quantitative) method
This chapter assesses and discusses both merits and drawbacks of each one of these methods in detail.
Physiologic Method
![$$ \left[{\mathrm{H}}^{+}\right]=\frac{\mathrm{K}\times \left[{CO}_2\right]}{\ \left[{HCO_3}^{-}\right]} $$](https://i0.wp.com/abdominalkey.com/wp-content/uploads/2020/10/480755_1_En_3_Chapter_TeX_Equ1.png?w=960)
![$$ pH= pKa\kern0.5em +\log \frac{\left[{HCO_3}^{-}\right]}{\left[{\mathrm{H}}_2{CO}_3\right]} $$](https://i0.wp.com/abdominalkey.com/wp-content/uploads/2020/10/480755_1_En_3_Chapter_TeX_Equ2.png?w=960)
The supply of H+ and HCO3 − is unlimited as long as the availability of pCO2 is normal. Initially CO2 combines with water to form H2CO3, which dissociates (ionizes) into H+ and HCO3 − (as discussed in Chap. 2). At pH of 7.40, most of the dissolved CO2 is maintained as HCO3 −, as shown below:

This reaction is catalyzed by carbonic anhydrase (CA) and is extremely important in biologic fluids because of formation of not only HCO3 − but also [H+] and thus plays an important role in acid–base physiology.
Normal compensatory responses to primary acid–base disorders
Acid–base disorder | Compensatory response |
---|---|
Metabolic acidosis | pCO2 = HCO3 × 1.5 + 8 ± 2 or for each mEq/L decrease in HCO3 −, pCO2 decreases by 1.2 mmHg |
Metabolic alkalosis | For each mEq/L increase in HCO3 −, pCO2 increases by 0.7 mmHg |
Respiratory acidosis | |
Acute | For each mmHg increase in pCO2, HCO3 − increases by 0.1 mEq/L |
Chronic | For each mmHg increase in pCO2, HCO3 − increases by 0.4 mEq/L |
Respiratory alkalosis | |
Acute | For each mmHg decrease in pCO2, HCO3 − decreases by 0.2 mEq/L |
Chronic | For each mmHg decrease in pCO2, HCO3 − decreases by 0.4 mEq/L |
Anion Gap
The introduction of anion gap (AG) has largely improved the interpretation of acid–base disorders by the physiologic approach. The AG elucidates, in particular, the cause of metabolic acidosis. Full discussion of AG is presented in Chap. 4. It signifies the presence of unexplained anions, such as the presence of organic acids. Normal AG varies between 3 and 11 mEq/L. Patients with metabolic acidosis may have normal, high, or low AG. Generally AG is influenced by the levels of albumin; therefore, the AG should be corrected for hypo- or hyperalbuminemia. Since critically ill patients have hypoalbuminemia, uncorrected AG may lead to misdiagnosis.
Another concept that is useful in the evaluation of physiologic acid–base disorder is ⧍AG/⧍HCO3 −. When applied appropriately, the derived ratio from this formula will unmask the presence of another acid–base disorder that is superimposed on the primary acid–base disturbance.
In summary, the physiologic method follows [H+], pH, HCO3 −/CO2 buffer system, AG, and ⧍AG/⧍HCO3 − with secondary physiologic response to explain both primary metabolic and respiratory as well as mixed acid–base disorders. However, this method has been criticized by many investigators. Some of the critic in terms of merits and drawbacks is presented below.
Merits
- 1.
It is widely used because it is simple and easy to follow at bedside
- 2.
The AG is easy to calculate, and the corrected AG is useful in elucidating the cause of metabolic acidosis
- 3.
It is easy to evaluate the mixed acid–base disorder and its etiology
Drawbacks
- 1.
The method is descriptive rather than mechanistic in nature
- 2.
No linear relationship exists between pH and [H+] throughout the pH scale (see Table 1.1). For example, the decrease in pH from 7.40 to 7.20 is associated with linear increase in [H+] from 40 to 63 nmol/L, whereas a drop from 7.00 to 6.80 is associated with nonlinear increase from 100 to 159 nmol/L. This suggests that changes in pH are not consistent with changes in [H+]
- 3.
The Henderson–Hasselbalch equation suggests only HCO3 −/CO2 buffer system and not the other buffer systems, although this equation considers HCO3 −/CO2 buffer is in equilibrium with other buffer systems (isohydric principle).
- 4.
The Henderson–Hasselbalch equation does not include all concentrations of anions and cations in the evaluation of acid–base balance
- 5.
Although pCO2 and HCO3 − are interdependent, the physiologic method assumes HCO3 − behaves like an independent variable, and its concentration determines the metabolic component of acid–base disorder
- 6.
HCO3 − is not always a representative of metabolic acidosis, because concentration is high not only in metabolic alkalosis but also high in chronic respiratory acidosis. Similarly [HCO3 −] is low both in metabolic acidosis and respiratory alkalosis. Thus, HCO3 − alone is not the only determinant of metabolic acid–base disorder because its concentration is affected both by pCO2 (a respiratory component) and pH or [H+] (a metabolic component)
- 7.
Uncorrected AG may lead to incorrect analysis of metabolic acidosis
Base Excess Method
It is well established that pCO2 is the determinant of respiratory acid–base disorder. Since HCO3 − is dependent on pCO2, several investigators tried to develop an independent marker to identify metabolic (nonrespiratory) acid–base disorder independent of pCO2. Some of the terms that were proposed to express the metabolic component of the acid–base disorder were (1) buffer base, (2) standard HCO3 −, and (3) base excess. We will discuss briefly these three terms.
Historically, the concept of base excess originated from the work of Singer and Hastings in 1948 [4], who introduced the term whole-blood buffer base as a measure of metabolic acid–base disturbance independent of pCO2 and HCO3 −. They defined buffer base as the sum of fixed cations (or strong bases) minus the sum of fixed anions (strong acids). A fixed ion is an ion that is unable to bind or give off an H+. The important fixed cations in plasma are Na+ and K+ except H+. The important fixed anion in plasma is Cl−, and nonfixed anions are HCO3 −, albumin, hemoglobin, and phosphate. These nonfixed anions are considered weak acids or buffer acids, as they bind H+. Based on the above information, buffer base is simply defined as the sum of plasma HCO3 − and nonfixed buffer ions such as albumin, hemoglobin, and phosphate.

Although measurement of buffer base was not easy in 1948, Singer and Hastings constructed a nomogram from which they derived the value of buffer base. Singer and Hastings did not recognize that buffer base, as mentioned above, does not change with respiratory disturbance. However, in the whole body or in vivo, the buffer base does not remain constant. Also, buffer base changes with changes in albumin and hemoglobin concentrations.
In subsequent years, other investigators, particularly, Siggaard-Andersen, Engel as well as Astrup [5–8], introduced the term base excess as a measure to quantify the metabolic (nonrespiratory) component of an acid–base disorder. To determine the base excess, Siggaard-Andersen and colleagues conducted in vitro experiments that equilibrated blood at pCO2 of 40 mmHg and at 37 °C to avoid any respiratory interference. They then quantified the amount of either strong acid (HCl) or strong base (NaOH) required to return 1 L of whole blood to a pH of 7.40. This quantity of acid or base is called the base excess, which is negative when NaOH was used (acidosis) or positive when HCl was used (alkalosis). Base excess is, thus, defined as the amount of base or acid required to titrate in vitro 1 L of whole blood to a pH of 7.40 at pCO2 of 40 mmHg and a temperature of 37 °C. These equilibration studies were performed with whole blood with the hemoglobin concentration of approximately 14–15 g/dL. As hemoglobin is the most important nonbicarbonate buffer, changes in hemoglobin will influence not only buffer base but also base excess. Since blood was equilibrated with pCO2, base excess is not influenced by changes with respiration or pCO2.
In vitro evaluation of base excess may not be applicable to in vivo or whole organism, since CO2 equilibration in vivo occurs not only in blood (intravascular) but in interstitial compartment (extravascular) as well. Also, hemoglobin concentration is not the same in blood and interstitium because of different water content in both compartments. Interstitium has more water content than intravascular compartment, and thus hemoglobin concentration is much lower in the interstitial compartment. This will cause changes in base excess. To overcome this, Siggaard-Andersen modified the original equation of base excess and introduced the term standard base excess as a measure of metabolic component of an acid–base disorder. This standard base excess was derived from equilibration with hemoglobin concentration of 5 g/dL, which is the concentration in the interstitium. Initially, a nomogram was constructed to read the standard base excess value, but currently blood gas analyzers automatically measure standard base excess as base excess.
Base excess (mEq/L) is usually expressed as positive (+) or negative (−). Positive base excess means an excess of base or lack of acid, and a negative base excess indicates excess of acid or lack of base. Negative base excess is also called base deficit, which is expressed numerically as 5 or −5. Also, base deficit is expressed as base excess −5. Thus, base deficit and base excess are used interchangeably. Normal base excess values range from −3 to +3. Therefore, base excess of −5 indicates metabolic acidosis, whereas +5 indicates metabolic alkalosis.
Base excess values are frequently used in critically ill patients to analyze acid–base disturbance [9]. For example, the pH indicates either acidosis or alkalosis, pCO2 indicates the respiratory component, and base excess implies the metabolic component. Thus, the intensivists and anesthesiologists will have a quick analysis of an acid–base disturbance at bedside to make therapeutic decisions.
Base excess seems to have a prognostic value in critically ill patients [9]. Studies in trauma, burn, and other ill patients have shown that negative base excess (−6 mEq/L) carried high morbidity and mortality. However, no consistent correlation was found between base excess and other metabolic parameters such as lactate, HCO3 −, or uncorrected or corrected AG.
Similar to physiologic approach, base excess method has some merits and drawbacks.
Merits
- 1.
Easy to apply at the bedside
- 2.
As mentioned above, base excess is a prognostic indicator in trauma and burn patients
- 3.
Base excess can be used to calculate the amount of NaHCO3 needed to correct the base deficit to improve pH, as follows:
Patient’s weight (kg)
HCO3 − space (usually 30–40% of body weight)
Base deficit (e.g., −6 mEq/L)
Example: Weight = 70 kg
HCO3 − space = 40%
Base deficit = 6
Amount of NaHCO3 needed = 70 × 0.4 × 6 = 168 mEq
Drawbacks
- 1.
Base excess measurements are variable from machine to machine. Not only intermachine variability but also intramachine variability is rather common. This variability may lead to incorrect diagnosis and raises the question of clinical validity of base excess. A variability in base excess of approximately 3.7 mEq/L has been reported when measured with different modern blood gas analyzers
- 2.
Base excess can be altered by (1) dilution of plasma, (2) changes in Cl−, and (3) changes in albumin and unmeasured anions
- 3.
Base excess is calculated from the arterial blood sample and may not be useful with venous blood samples
- 4.
The negative (e.g., −4) or positive (e.g., +4) base excess does not necessarily mean that metabolic acidosis or alkalosis is present. For example, in chronic respiratory acidosis, the base excess is positive due to compensatory increase in [HCO3 −] in the absence of metabolic alkalosis and negative in chronic respiratory alkalosis due to a decrease in [HCO3 −] in the absence of metabolic acidosis. In acute respiratory acidosis, the base excess is normal, but pCO2 is high
- 5.
In mixed acid–base disorders, base excess may lead to erroneous diagnosis. For example, the base excess in a patient with chronic respiratory acidosis is positive. If this patient develops acute metabolic acidosis (lactic acidosis due to sepsis), the base excess decreases without much change in pCO2, suggesting that the patient has acute respiratory acidosis rather than metabolic acidosis that is superimposed on chronic respiratory acidosis. Thus, the real diagnosis of acid–base disturbance is missed. The above example also suggests that base excess cannot determine the cause of metabolic acidosis
Analysis of an Acid–Base Disorder Using Physiologic and Base Excess Methods
Case | Normal |
---|---|
pH = 7.32 | pH = 7.40 |
pCO2 = 80 mmHg | pCO2 = 40 mmHg |
HCO3 − = 40 mEq/L | HCO3 − = 24 mEq/L |
Standard HCO3 − = 32 mEq/L | Standard HCO3 − = 23 mEq/L |
Base excess = 11 mEq/L | Base excess = −3 to +3 mEq/L |
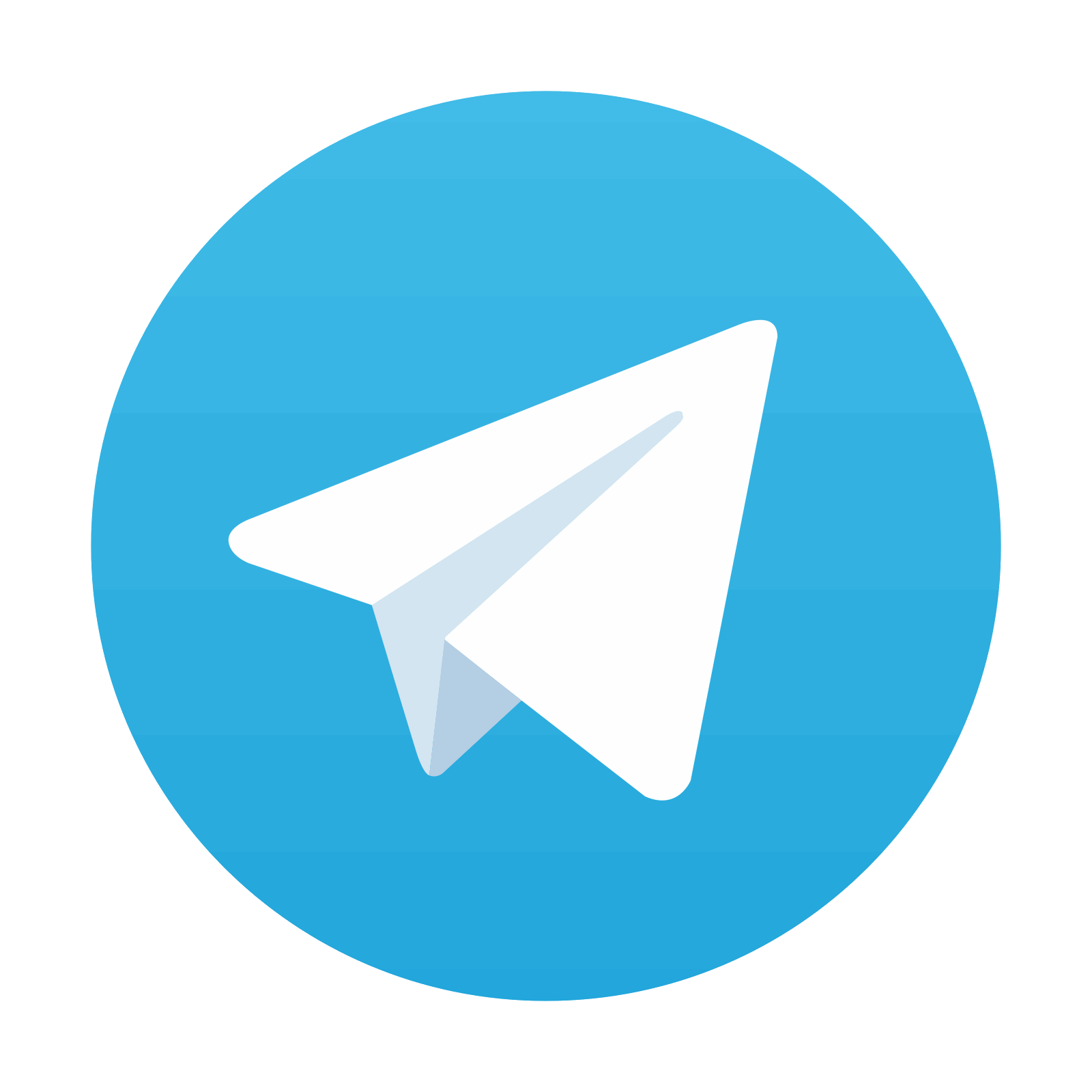
Stay updated, free articles. Join our Telegram channel

Full access? Get Clinical Tree
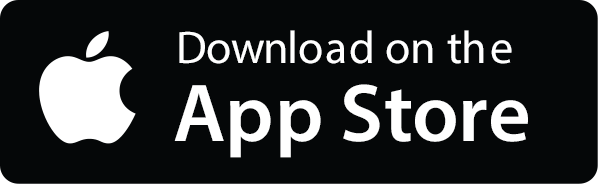
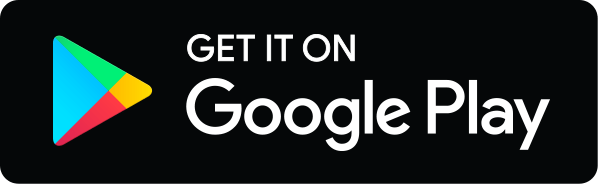