Timothy A. Morris
Venous thromboembolism (VTE) can be entirely preventable or treatable. Yet, it is a persistent and prevalent cause of significant morbidity and mortality in the United States and is responsible for an estimated 50,000 deaths and 500,000 nonfatal episodes each year. Despite important diagnostic and therapeutic developments, medical science is only scratching the surface at understanding VTE, and clinical strategies have only partially impacted disability and death following VTE.
Venous thromboembolism, by definition, originates in systemic venous thrombosis, and is pathologically distinct from arterial thrombosis. Conditions that favor thrombosis fall into three categories (as predicted by Virchow over a century ago): (1) venous stasis, (2) injury to the venous intima, and (3) alterations in the coagulation–fibrinolytic system. The potential role of all three factors has been demonstrated in a variety of situations. Venous stasis occurs during bed rest and is associated with deep venous thrombosis (DVT); using intermittent compression stockings to restore venous flow reduces the risk for DVT. Injury to the venous wall is the most likely mechanism of the large number of lower extremity DVTs observed in proximity to sites of trauma and major orthopedic surgery. Coagulation abnormalities that occur alone or in concert with other conditions can promote clinical venous thrombosis. For example, there is an increased risk for VTE associated with mutations in factor V (factor VLieden) and in the untranslated region of the gene encoding prothrombin (although the procoagulant mechanism of the later mutation is still under investigation). Other less prevalent, but probably more potent, “thrombophilias” include deficiencies of antithrombin III, protein C, and protein S; aberrations in the thrombolytic system; and the presence of the doubly misnamed “lupus anticoagulant.” Clinical conditions that involve combinations of these fundamental risk factors are associated with a higher risk of thrombosis.
Many large studies have identified the major risk factors for DVT of the lower extremities. These include: (1) surgery involving general anesthesia for more than 30 minutes, (2) injury or surgery involving the lower extremities or pelvis, (3) congestive heart failure, (4) prolonged immobility from any cause, and (5) pregnancy, particularly during the postpartum period. Other conditions that increase risk are cancer, obesity, advancing age, varicose veins, a prior episode of DVT, use of estrogen-containing compounds, and dehydration. Predictably, these risk factors are cumulative.
The deep veins of the lower extremities are the dominant source of clinically significant pulmonary emboli—an important epidemiologic, diagnostic, and therapeutic consideration. Less commonly, thrombi can arise in superficial veins or in prostatic, uterine, renal, and other veins. They also can occur in the right cardiac chambers in patients with right ventricular failure. However, more than 95% of clinically significant pulmonary emboli arise from DVT in the lower extremities (whether or not such DVT is clinically detectable).
The events initiating venous thrombosis are not fully understood. The valves of the lower extremity veins, especially in the calves, are common sites for the initial event. The development of a small nidus leads to the elaboration of clot-potentiating materials that trigger prolongation of the thrombus with red blood cells (RBCs), fibrin and, to a lesser degree, platelets. Once formed, the thrombus grows by accumulating additional RBC, fibrin, and platelet “layers,” seen pathologically as the lines of Zahn.
Even as thrombosis is occurring, the process of resolution is beginning. The thrombi resolve by one or both of two mechanisms: fibrinolysis and organization. Fibrinolysis refers to actual dissolution of the thrombus by plasma enzymes. It is a relatively rapid process, proceeding over a period of hours to several days. If fibrinolysis is not totally successful, organization finishes the job of resolution. Reparative cells infiltrate the residual thrombus and replace the “thrombotic components” with connective tissue. The fibrotic residuum is then incorporated into the venous wall and re-endothelialized. Organization usually thickens the venous wall, which may provide loci for further thrombus formation. The thickening may also incorporate one or more venous valves, rendering them incompetent. Whatever the fate of a given thrombus, available data indicate that the sequence of resolution is complete within 7 to 10 days. By that time, the initial thrombus is gone or has been incorporated into the venous wall. In the latter case, the pathology is more accurately termed a venous scar than an old clot.
At any time during the process of resolution, pulmonary embolism (PE) can occur. It is important to recognize that embolism is not a new disorder; it is a serious complication of DVT. Because thrombi are most friable early in their development, embolic risk is highest during the first few days after thrombus formation. Thereafter, dissolution or organization sharply limits embolic risk (as long as no new thrombotic material has been laid down in the interval).
When emboli arise and lodge in one or more pulmonary arteries, hemodynamic and respiratory consequences occur. The hemodynamic consequences include a decrease in the available cross-sectional area of the pulmonary arterial system through both mechanical obstruction and release of vasoconstrictive thrombus metabolites directly into the vascular bed. The pulmonary vascular resistance rises, causing an increased pulmonary arterial pressure, and therefore an increased right ventricular workload. If these consequences are severe, the right ventricle may not tolerate the workload and the cardiac output will fall. Respiratory consequences include: (1) altered ventilation–perfusion relationships, which (combined with a fall in cardiac output and resulting lowered venous oxygen concentration) may lead to arterial hypoxemia, (2) development of one or more zones of alveolar dead space (zones that are ventilated but not perfused), (3) transient pneumoconstriction of these same zones, (4) hyperventilation (the reasons for which are debated), and (5) loss of surfactant in the underperfused zones. The first four events occur immediately; the fifth requires approximately 24 hours of total occlusion before alveolar surfactant is depleted. The two major consequences of surfactant depletion are atelectasis and an increase in permeability of the alveolar–capillary membrane, causing further problems with gas exchange.
Pulmonary infarction is a rare consequence of embolism; fewer than 10% of emboli lead to infarction. Therefore, embolism is by no means synonymous with infarction.
An important clinical question concerns the hemodynamic deterioration observed in some patients with PE in the first few days after embolization. Although PE can be immediately fatal, a large number of patients who eventually succumb, do so one or more days after presentation. It stands to reason that these “late fatalities” are caused by either progressively deteriorating myocardial fatigue (right ventricular infarction or other myocyte injury) or by increases in workload because of factors such as recurrent emboli, embolus propagation, or further release of vasoactive mediators from the embolus.
The phenomenon of embolic fragments entrapped in the right atrium and ventricle has been recognized in experimental embolism for some time; as cardiac echocardiography is more extensively utilized, this phenomenon has become more widely recognized in human disease as well. The clinical significance of these sessile cardiac thrombi has not been studied in a controlled fashion. However, it is likely that these thrombi may embolize; therefore, larger cardiac thrombi may warrant emergent surgical removal, especially in the presence of preexisting hemodynamic compromise.
Beyond these acute events, emboli (like venous thrombi) tend to resolve if prevented from propagating by anticoagulants. Precise data on the speed of resolution in humans are not available. The earliest reported time of total embolic resolution is about 2 days; most resolve substantially or completely within a few weeks. A very small number fail to dissolve, for unknown reasons, and form permanent vascular scars within the pulmonary arteries, causing chronic thromboembolic pulmonary hypertension (see Chapter 67).
Signs and symptoms of DVT are inconsistent and are essentially manifestations of its two consequences: inflammation of the venous wall and venous obstruction. The former may lead to local pain, tenderness (tenderness along the vessel wall is particularly suggestive), redness, and warmth; the latter may lead to edema in the leg zones drained by the vein(s) involved. Unfortunately, studies have demonstrated that fewer than half of patients with DVT have signs or symptoms at all and few have sufficient inflammation or edema to allow a clinical diagnosis to be made. Therefore, reliable and early detection requires that laboratory tests be used to supplement history and physical examination.
Three well-validated diagnostic procedures are generally available to diagnose and follow the course of DVT: compression ultrasound, impedance plethysmography, and contrast venography. Radiolabeled fibrinogen, a previously invaluable investigative tool for detecting thrombus presence and propagation, is no longer available. Other tests such as serologic markers of thrombosis/thrombolysis, magnetic resonance imaging (MRI) and, radiolabeled thrombus-specific agents are under development, but have not been completely validated for clinical use.
Compression ultrasonography involves the use of ultrasound visualization and Doppler analysis to distinguish between solid (thrombus) and fluid (blood) contents of the proximal deep veins of the leg. Failure to compress visualized veins suggests that at least part of the lumen is filled with solid material and is the only reliable criterion for DVT diagnosis. Findings such as “echogenic densities” or Doppler blood flow velocity measurements have not proved reliable in clinical studies and should not be used to make the diagnosis. The technique is not reliable in detecting thrombi limited to the calf or iliac veins. In addition, the vessel wall thickening from prior DVTs causes wall thickening in nearly half of the cases, which can cause non-compressibility on ultrasound. Even the most rigorously controlled clinical trials, using complex algorithms to compare old and new studies side by side, distinguished new thrombi from old scars only with great difficulty. In its current state, compression ultrasonography should not be used to diagnose recurrent DVT at the site of prior thrombosis.
Impedance plethysmography (IPG), which measures the rate of venous drainage from the leg, is positive when there is substantial obstruction to venous outflow at any point from the popliteal vein to the inferior vena cava. It is sensitive to above-the-knee thrombi, especially when unilaterally positive. The test has been well-validated in clinical studies and is a relatively inexpensive, standardized method to detect DVT. A great deal of work has been performed comparing the accuracy of IPG to that of compression ultrasound. As a group, these studies show compression ultrasound to be more accurate, although it is more expensive and highly operator-dependent. Unlike ultrasound, IPG has the benefit of returning to normal within weeks of an acute DVT, making it useful for diagnosing DVT recurrence. It should be noted that neither of these noninvasive tests reliably detects calf thrombi or asymptomatic proximal vein thrombi.
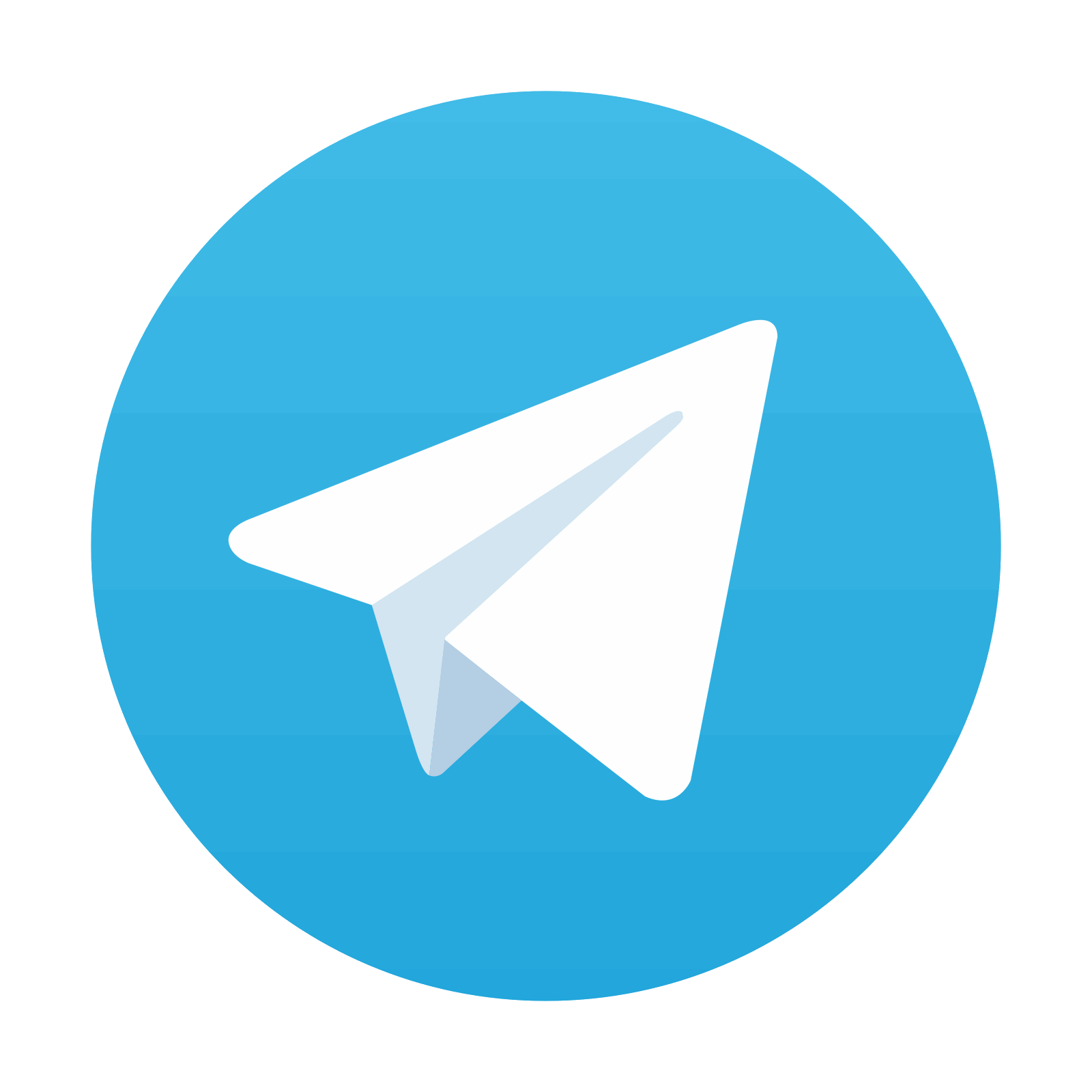
Stay updated, free articles. Join our Telegram channel

Full access? Get Clinical Tree
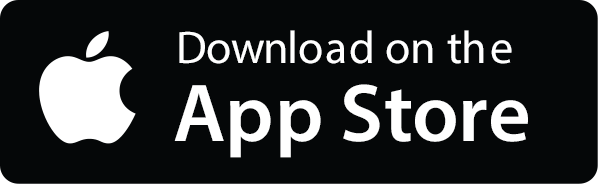
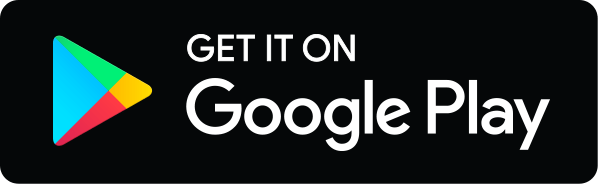