Fig. 7.1
Schematic diagram of oxalate utilisation by O. formigenes. (1) Oxalate2− enters the cell via an oxalate:formate antiporter (OxlT). (2) Oxalate2− is activated by the transfer of CoA from a formyl-CoA donor in a reaction catalysed by a formyl-CoA transferase (Frc). (3) Oxalyl-CoA is decarboxylated by an oxalyl-CoA decarboxylase (Oxc)
Lactobacillus spp. and Bifidobacterium spp.
Perhaps the candidates with the greatest potential for the treatment of kidney stone disease are the oxalate-degrading Lactobacillus and Bifidobacterium spp. [2]. These genera have a long history of use as probiotics and many species have been granted ‘Generally Regarded as Safe’ (or ‘GRAS’) status, which facilitates their use commercially. They appear to degrade oxalate through a similar mechanism to O. formigenes, possessing both oxc and frc genes. However, the mechanism of oxalate uptake for these genera has not yet been elucidated. It is interesting to note that Lactobacillus and Bifidobacterium spp can grow on a range of alternate energy sources in addition to oxalate and are, therefore, called “generalist oxalotrophs”. This allows them to survive in the gut even when oxalate levels are low.
The majority of genetic research thus far has focussed on Lactobacillus acidophilus and Lactobacillus gasseri. In both species the frc and oxc gene homologues are situated adjacent to one another on the genome, unlike the arrangement in O. formigenes where the genes are located separately [8, 26, 55]. Importantly, expression of both genes requires mildly acidic (pH 5.5) growth conditions, similar to those present in the lower GIT. It is possible that one or both of the gene products function during the bacterial response to acid stress as the consumption of H+ ions during oxalate degradation can help to buffer the internal pH of the cell. This is seen in experiments using L. acidophilus, where inactivation of the frc gene resulted in increased susceptibility toward oxalic acid [8].
Bifidobacterium animalis subsp. lactis is the only member of the bifidobacteria for which a functional characterisation of the genes involved in oxalate degradation has been carried out. Screening of a B. animalis subsp. lactis genomic library using a probe prepared from the oxc gene from O. formigenes allowed the identification of a oxc homologue [15]. Later studies identified a putative frc homologue as well as a conserved hypothetical protein, which may function as an oxalate transporter [54]. As for the lactobacilli, acidic conditions were required for expression of the frc and oxc genes, although in this case a lower pH was required (pH 4.5). Recently published genome sequences have revealed that putative frc and oxc genes are present in several additional Bifidobacterium spp., including B. dentium, B. gallicum, B. pseudocatenulatum and B. pseudolongum, however, functional characterisation of the genes is lacking in these species.
In Vitro and Animal Models for Oxalate Utilization by GIT Bacteria
The in vitro oxalate degradation capacity of the various Lactobacillus and Bifidobacterium spp has been shown to be both species and strain specific. Mogna et al. [36], demonstrated that the best oxalate-degraders (68 %) in the Lactobacillus group came from L. paracasei, L. gasseri or L. acidophilus while Turroni et al. [55] isolated a range of novel Lactobacillus spp (including L. acidophilus and L. gasseri) that could degrade more than 50 % of the oxalate present. In the Bifidobacterium group, Federici et al. [15] reported that the highest level of oxalate degradation was with Bifidobacterium lactis DSM 10140 at 61 %, while Bifidobacterium longum MB 282 and Bifidobacterium adolescentis MB 238 showed 35 % and 57 % degradation respectively. In general, all the Bifidobacterium strains tested had lower degradation activity than the lactobacilli, possibly due to intrinsic oxalate toxicity toward the former genus.
In the search for efficient oxalate degraders, laboratories have also screened novel isolates from the guts of various animals. Murphy et al. [38] isolated Bifidobacterium and Lactobacillus strains from cats and dogs, and demonstrated in vitro oxalate degradation in 61 % of the Lactobacillus isolates. In contrast, the Bifidobacterium spp. showed very little degradation activity for all species tested. Two strains of L. murinus and two of L. animalis were tested in a rat model, but only the L. animalis strains gave significant reduction in urinary oxalate levels. Other workers have screened probiotic strains for both oxalate-degradation activity and also their ability to modulate inflammation in the human GIT, and have found strains of L. plantarum, L. acidophilus, B. breve and B. longum to be promising candidates for this purpose in in vitro testing [17].
Gut Colonisation and In Vivo Bacterial Oxalate Utilization Studies in Humans
A number of human in vivo studies have been undertaken to examine the effect of oral administration of lactic acid bacteria probiotics on dietary hyperoxaluria, plasma oxalate concentration and urinary oxalate excretion. However, most of these studies are limited in various ways, with low numbers of participants and varying test procedures making evaluation of their comparative effectiveness difficult.
The earliest studies suggested that lactic acid bacteria, such as Lactobacillus and Bifidobacterium in particular, reduced urinary oxalate excretion by 40–50 % in a study concerning six calcium-stone forming participants [11]. The reduction persisted after the treatment ceased. This was followed by a study with participants with enteric hyperoxaluria, where the Oxadrop (VSL Pharmaceuticals) probiotic preparation was used. This was comprised of a mixture of four lactic acid bacterium species (L. acidophilus, L. brevis, S. thermophilus and B. infantis). One packet of Oxadrop per day reduced urinary oxalate excretion by 19 %, and this increased to 24 % when two packets per day were administered [28]. This study had 20 participants, 10 receiving Oxadrop daily and 10 in the placebo group.
However, more recent studies, using randomized double-blind groups of stone-formers with idiopathic hyperoxaluria, were unable to show a reduction in urinary oxalate excretion, despite using the same Oxadrop probiotic preparation at the same concentration of 4–9 × 1011 cfu. The sample size in each case was also a total of 20 participants, 10 receiving Oxadrop daily and 10 in the placebo group [18]. Lieske et al. [29] conducted a double-blind study to determine the effect of Oxadrop and the synbiotic AKSB preparation containing fructooligosaccharides, Enterococcus faecium and Saccharomyces cerevisiae (Agri-King Synbiotic, Fulton, USA) on 40 participants with mild hyperoxaluria receiving an oxalate controlled diet. They concluded that while the restricted diet reduced urinary oxalate, the probiotics and synbiotics did not. However, it is not clear what the basis of the choice of the organisms in the AKSB preparation was, nor how they might influence oxalate metabolism. Recently, Siener et al. [49] placed 20 healthy participants on an oxalate-rich diet for 6 weeks, and administered Oxadrop for 5 weeks. Urinary oxalate excretion and plasma oxalate concentrations increased with the oxalate-rich diet, and were not reduced by the ingestion of probiotics in the treated group.
A different probiotic preparation, VSL#3 (Sigma Tau Pharmaceuticals, USA) was used in two studies. This formulation contained eight different Lactobacillus, Streptococcus and Bifidobacterium strains, none of them the same as Oxadrop. In the first of these studies, a significant reduction in urinary oxalate excretion was observed in subjects with an oxalate-rich diet after probiotic administration, but this occurred only in those four subjects with a very high level of oxalate absorption from the gut [39]. In the second study, 11 healthy participants were given a high oxalate diet of 176 mg oxalate per day, and either one dose or two doses of VSL#3 daily. The results showed that either dosage of probiotics reduced urinary oxalate and increased oxalate absorption. However, the absorption was only monitored 6 h after ingestion, and not after a 24-h period as in other studies [4]. Some laboratories isolate and test their own probiotic mixtures. Ferraz et al. [16] treated 16 stone-forming patients with hyperoxaluria while on a controlled oxalate-rich diet with a mixture of L. casei and B. breve, but had variable results in the lowering of urinary oxalate.
Liebman and Al-Wahsh [27], in reviewing the effects of probiotics on dietary oxalate absorption, concluded that the variation in the results between laboratories was due to differences in protocols, variations in diet and perhaps the choice and administration of the specific probiotics. However, there is evidence that probiotics can, in certain cases, contribute to a reduction in oxalate after a high oxalate diet [39] or in cases of enteric hyperoxaluria [28]. There is, therefore, a need for more rigorous studies on the use of oxalate-degrading lactic acid bacteria for the treatment of patients at risk of developing kidney stones. Effective bacterial strains and administration protocols have still to be developed and proven for future therapeutic applications.
In particular, it will be important to consider the issues around the benefits of chronic administration of the oxalate degrading probiotics as opposed to undertaking permanent recolonisation of the guts of patients suffering from enteric hyperoxaluria. There is currently limited information correlating the lack of a particular oxalate-degrading bacterial population and the development of kidney stone disease due to the variations in normal base-line microbial numbers [23]. The many factors affecting microbial colonisation, including diet and host-microbial interactions, also require further investigation [41, 56].
Novel Recombinant Approaches (Oxalate Decarboxylase from B. subtilis and Expression in L. plantarum)
A more recent alternative approach to enhancing microbial oxalate degradation in the gut is to introduce higher levels of an oxalate degrading enzyme, such as oxalate decarboxylase, either delivered in the form of in vitro purified recombinant enzyme or expressed in vivo in a naturally occurring recombinant LAB species which was previously unable to degrade oxalate [47]. Oxalate decarboxylase (OxDC) (EC4.1.1.2) has been found in several fungi [51] as well as certain bacteria, such as Bacillus subtilis [52], and catalyses the conversion of oxalate to carbon dioxide and formate [22]. The B. subtilis OxDC enzyme is encoded by the oxdC gene (formerly known as yvrk) and was induced under acidic conditions (pH 5.0) but not by the presence of oxalate. The purified B. subtilis OxDC activity was catalytically dependent on low concentrations of O2 [42]. However, it retained up to 76 % of its activity when tested in vitro under anaerobic conditions at pH 5.0 [52]. In addition, since the human gut demonstrates an intraluminal oxygen gradient [3], there is the potential availability of catalytic concentrations of oxygen sufficient to drive the enzyme reaction in vivo. These features make the OxDC enzyme an appealing candidate for possible heterologous expression and use in the treatment of hyperoxaluria.
Use of Free Oxalate Decarboxylase for In Vivo Oxalate Degradation
The first work reported in this field aimed at introducing oxalate decarboxylase into the gut through the direct ingestion of in vitro purified recombinant protein. The perceived advantage of this approach was that it aimed at providing a specific dose of a stable and active oxalate degrading enzyme throughout the gut [19]. The B. subtilis OxDC enzyme was expressed in E. coli and purified [22]. The processed protein crystals were cross-linked with gluteraldehyde (OxDC-CLECR) to provide an active preparation for administration in in vivo trials using mice suffering from hyperoxaluria due to an Agxt gene (alanine-glyoxylate aminotransferase) mutation [19]. It was found that a daily dose of 200 mg of the preparation, given for 16 days, reduced the faecal oxalate concentration of the mice by 72 %, and the urinary oxalate by 44 %, relative to a control group receiving a placebo (n = 7 per group). When the experiment was repeated in the presence of ethylene glycol (EG), which induced nephrocalcinosis and kidney failure, it was found that 80 mg per day of the OxDC-CLECR preparation given to the test group (n = 11) for 32 days was sufficient to cause a urinary oxalate reduction of 40 %, prevented the EG-induced kidney pathology, and there was 100 % animal survival.
Cowley et al. [13] developed an improved recombinant mutant form of the B. subtilis OxDC enzyme by replacing the cysteine residue at position 383 with a serine to prevent auto-aggregation of the protein without loss of enzyme function in vitro. This compound was named OC4 (Oxazyme). Its oral toxicity in rats and dogs was evaluated by oral gavage over a 14 day period at 50 times the anticipated clinical dose, and no adverse toxic or adverse effects were observed during this period or in a subsequent 7 day recovery period. The oxalate degrading ability of Oxazyme was also tested in vitro in simulated gastric and intestinal environments [37]. The oxalate decarboxylase enzyme completely degraded oxalate derived from potassium oxalate under pH conditions equivalent to those found in the stomach and the proximal colon. It also significantly reduced the oxalate concentration of homogenised spinach (a food with a high oxalate content) under both conditions. Oxazyme was subsequently used in a limited non-randomised human clinical trial at the Mayo Clinic (ClinicalTrials.gov Identifier: NCT01127087; Principal Investigator Dr John Lieske, MD). All participants showed no adverse effects, and there was some indication of a statistically significant reduction in urinary oxalate. However, the number of participants completing the trial was very small, and the need for a more robust, extensive study is indicated.
Expression of Oxalate Decarboxylase in a Suitable Heterologous Probiotic Host Bacterium
Oxalate decarboxylase could also be delivered to the gut as a recombinant enzyme expressed in vivo in a suitable LAB species with the aim of enhancing oxalate degradation in the human gut and the prevention of hyperoxaluria. One of the major criteria that need to be considered when selecting a suitable heterologous host probiotic strain for human use is that the bacterium should effectively colonise the mucosal surface of the gut. Studies on Lactobacillus plantarum adhesion to the gut extracellular matrix (ECM) showed that four of the 16 indigenous Lb. plantarum strains tested showed significant ability to bind to both the fibronectin and mucin ECM components [58]. This organism, therefore, shows potential as a delivery vehicle for the OxDC enzyme as well as the potential capacity for long-term colonisation of the gut.
Cloning of oxdC from B. subtilis and Heterologous Expression in Lb. plantarum
Kolandaswamy et al. [24] examined the over-expression of the B. subtilis oxdC gene in Lb. plantarum NC8, a GRAS bacterium which does not normally express oxalate decarboxylase. The gene was cloned onto a shuttle vector and its expression was regulated from an inducible promoter by sakacin-P using the pSIP high-level expression system [50] thus producing an active recombinant OxDC protein. However, the expression of this enzyme in Lb. plantarum was intracellular and it was generated at very low levels from the inducible promoter to which the gene was fused.
With a view to improving these shortcomings and developing a recombinant strain which might degrade oxalate extracellularly and more efficiently and under human gut conditions, Sasikumar et al. [47] investigated the use of signal peptides to drive the secretion of the recombinant enzyme. Lb. plantarum WCFS1, a human saliva isolate, was used to express the B. subtilis oxdC gene cloned downstream of the same sakacin-P (inducible) promoter used by Kolandaswamy et al. [24] but this time fused to homologous peptide sequences Lp_0373 or Lp_3050, which had previously been shown to enhance secretion in this host [34, 35]. They found that a functional OxdC protein was secreted efficiently from cells carrying these constructs and could degrade up to 50 % of the oxalate in the growth medium.
Anbazhagan et al. [6] further developed the system by introducing a constitutive promoter, which required no induction, ahead of the signal sequences and the cloned oxdC gene. The Lb. plantarum L-lactate dehydrogenase promoter was successfully used to overexpress functional, extracellular oxalate decarboxylase, suggesting that, in this form, the organism expressing the recombinant protein might be useful for degrading dietary oxalate in the gut.
In Vivo Evaluation of Recombinant Lb. plantarum Expressing OxDC
In a recent study, Sasikumar et al. [45, 46] compared the in vitro and in vivo oxalate degrading ability of Lb. plantarum WCFS1, expressing OxDC constitutively either intracellularly or extracellularly in vitro, in male wistar albino rats. All rats (n = 30) received 5 % potassium oxalate in their normal diets but the test groups (n = 5 per group) were, in addition, fed either of the two forms of the recombinant Lb. plantarum daily from day 14 for an additional 2 weeks. It was found that the rats receiving the recombinant bacterium showed a significant reduction in urinary oxalate as well as calcium, creatinine and uric acid as compared to the control group, and that the excreted form of the enzyme achieved this more efficiently. Examination of the kidney homogenates of the various groups also showed significantly reduced oxalate levels in the test group, and there was no microscopic histological sign of calcium oxalate crystallisation when compared to the rats which had not ingested the recombinant bacteria. The authors conclude that daily oral administration of a biologically contained bacterium expressing the enzyme in a secreted form may be a useful therapy for the treatment of calcium oxalate kidney stone disease.
< div class='tao-gold-member'>
Only gold members can continue reading. Log In or Register a > to continue
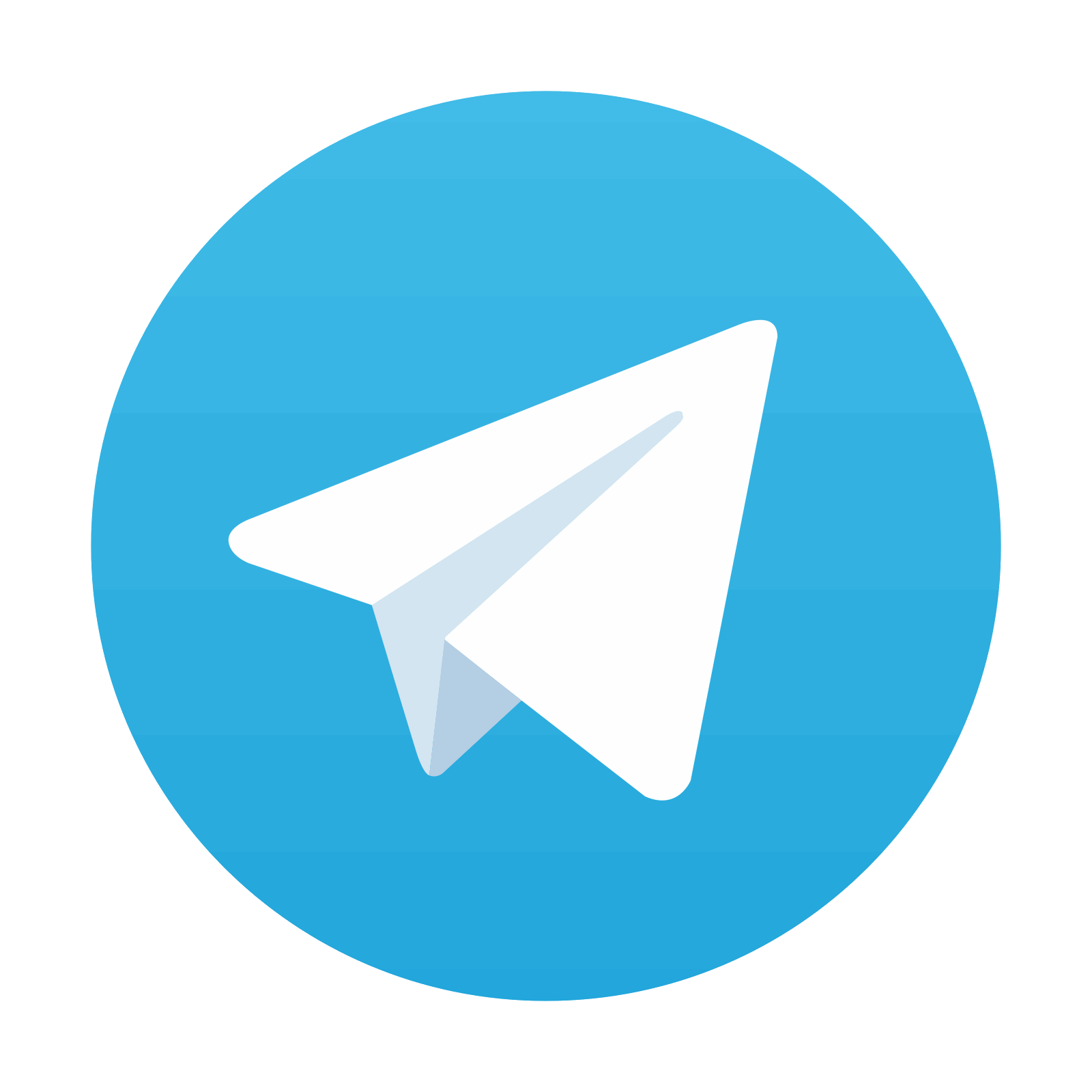
Stay updated, free articles. Join our Telegram channel

Full access? Get Clinical Tree
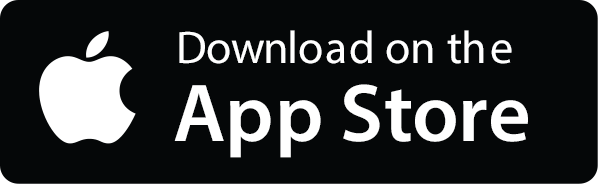
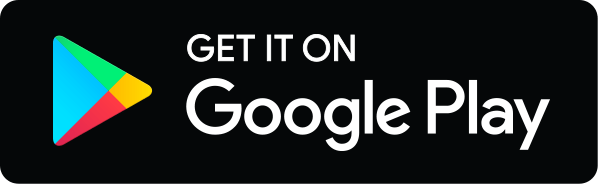