Fig. 22.1
Effects of RDN on BP (a) and changes in eGFR (b) over time in Symplicity HTN-1 with follow-up to 2 years (Reproduced from Symplicity HTN-1 Investigators [8], with permission)
Symplicity HTN-2 was designed as a randomized control study and results were reported in 2010 and 2012 [10, 11]. Enrolled resistant hypertensive patients with a baseline office systolic blood pressure >160 mmHg (>150 mmHg for patients with type 2 diabetes) were randomized to either undergo RDN (n = 52) or to continue their established conventional pharmacological treatment (n = 54). In line with the results of Symplicity HTN-1, renal denervation reduced office blood pressure –32 ± 23/12 ± 11 mmHg at 6 months [10], with sustained effect reported at 12-month follow-up (−28 ± 25/10 ± 11 mmHg) [11]. In contrast, no significant change was observed in the control group (1 ± 21/0 ± 10 in office blood pressure and 2 ± 13/7 ± 11 mmHg in home blood pressure) [10]. Mean estimated glomerular filtration ratio (eGFR) was unchanged in both groups at 6 months (0.2 ± 11 ml/min/1.73 m [2] in the RDN group and 0.9 ± 12 ml/min/1.73 m [2] in the control group) [10] (Fig. 22.2).


Fig. 22.2
BP reduction in Symplicity HTN-2 in the treatment and control group up at 3 and 6 months of follow-up (Reprinted from Esler et al. [10], Copyright (2010), with permission)
These trials had their origins in the three observations: (1) the renal sympathetic outflow is activated in patients with resistant hypertension; (2) overactivated renal sympathetic nerve results in higher blood pressure through the mechanisms of sodium and water retention, reducing renal blood flow, and activation of renin-angiotensin system; and (3) suppression of renal sympathetic nerve activity reduces blood pressure with sustained effects.
Symplicity HTN-3
The latest and the largest clinical trial of catheter-based renal denervation, Symplicity HTN-3, has been reported very recently [12]. This study was a rigorously designed randomized, blinded, sham-controlled trial. Patients in control group underwent renal angiogram, masked to mimic renal denervation. Patients’ enrollment criteria were essentially the same as previous Symplicity trials. Patients had to be treated with at least three antihypertensive medications at maximally tolerated dose, and one of which should be a diuretic. The regimen had to be unchanged for 2 weeks before the enrollment. Subsequently, patients needed to fulfill the blood pressure criterion which was systolic blood pressure ≥160 mmHg on a day of office visit. Automated 24-h ambulatory blood pressure monitoring was performed to confirm an average 24-h systolic blood pressure ≥135 mmHg to rule out white-coat hypertension. The renal denervation was performed by means of Medtronic Symplicity renal denervation catheter. The safety and efficacy endpoints were examined at 6-month follow-up. During the 6-month follow-up period, the regimen of antihypertensive medication was not allowed to change unless there was clinical necessity.
Among 535 uncontrolled hypertensive patients, 364 patients were blindly allocated to treatment group and underwent renal denervation across the USA. At 6 months after the procedure, despite a significant drop in office systolic blood pressure from the baseline in the treatment group, this was not statistically significant when compared to that of the sham procedure group (−14.1 ± 23.9 vs. –11.7 ± 25.9 mmHg, p = 0.26).
In contrast to Symplicity HTN-1 and HTN-2, the reduction of office blood pressure in the treatment group was less pronounced (−14.1 ± 23.9/−6.6 ± 11.9 in HTN-3 vs. −22/−11 mmHg in HTN-1 [7], and –32 ± 23/−12 ± 11 mmHg in HTN-2) [10]. Furthermore, there was a large effect in the sham control group; the drop in office systolic blood pressure was prominent compared to that of HTN-2 (−11.7 ± 25.3 vs. 1 ± 21 mmHg in HTN-2) [10]. Although the pretreatment blood pressure was similar, greater range of standard deviation in the treatment group of HTN-3 indicates wider variation in response. Interestingly, African-Americans had a less pronounced blood pressure effect in response to renal denervation, raising the question whether racial background may influence the response to the procedure. Of note, African-Americans have less effective blood pressure reduction with angiotensin-converting enzyme (ACE) inhibitors and beta-blockers [13].
Concerns have also been raised in regard to the operator experience in this US trial. The procedure of renal denervation in Symplicity HTN-3 was performed by 111 operators throughout the USA. Among them, 31 % (about 34 operators) had done only 1 procedure, and 85 operators had done <5 procedures. In addition, among 364 patients in the treatment arm, about half (181 patients) were treated by operators who had previously done <5 (mostly 1–2) procedures [12]. Although no significant difference was observed in outcomes between operators who performed <5 procedures and others, this may not eliminate the possible influence of the operators’ learning curve on relatively marginal reduction of blood pressure in the treatment group. From this point of view, ineffective renal denervation might be partly attributable to the neutral outcome of this study. However, the absence of tests to assess the quality of renal denervation makes it impossible to investigate this matter, unlike experimental renal denervation in animal studies.
The results raised some important issues that need to be resolved in future renal denervation studies. The procedure of catheter-based renal denervation is essentially different from traditional experimental denervation in animals, in which total renal denervation is accomplished by visually stripping and by painting phenol or xylocaine around the renal artery [5, 14, 15]. In contrast to animal experiments, a reliable test to confirm that renal denervation is successfully achieved is limited to the renal norepinephrine spillover and muscle sympathetic nerve activity (MSNA) in clinical studies [7, 16, 17], and the question remains what is accomplished by the catheter-based renal denervation.
While the evidence of the utility of renal denervation from experimental animal studies is very strong, it has to be considered together with outcomes of clinical research studies to inform future and better designed trials. In this regard, partial ineffectiveness of current clinical approaches to achieve successful renal denervation is a real possibility.
Irrespective of the results of Symplicity HTN 1–3, the therapeutic concept of renal denervation is likely to be most efficacious in conditions characterized by increased sympathetic drive, such as CKD, heart failure, and other cardiac-relating disorders. Below, we will discuss the potential roles of renal denervation in CKD from both a clinical and a basic science point of view.
Renal Denervation and Chronic Kidney Disease
CKD is one of the most important comorbidities of hypertension as CKD substantially contributes to the systemic fluid burden as well as sympathetic overactivity [18–21]. The mechanisms of renal sympathetic overactivity to elevate blood pressure include (i) urinary sodium retention and volume expansion, (ii) reduction in renal blood flow due to vasoconstriction, and (iii) activation of the renin-angiotensin system [1]. CKD therefore appears as a logical indication for renal denervation approaches [22]. Hering et al. reported the effects of catheter-based renal denervation on resistant hypertensive patients with moderate to severe CKD [23]. A significant drop in the office blood pressure (−34/−14, –25/−11, –32/−15, and –33/−19 mmHg at 1, 3, 6, and 12 months after RDN, respectively) was observed without deterioration in renal function and renal blood flow. These findings confirmed the safety and efficacy of catheter-based renal denervation in resistant hypertensive patients with CKD. Kiuchi et al. reported the beneficial effects of catheter-based renal denervation in 24 patients with CKD and refractory hypertension [24]. Using an irrigated cardiac ablation catheter, a significant improvement in eGFR (64.4 ± 23.9 to 85.4 ± 34.9 ml/min/1.73 m [2], at baseline and at 6 months after denervation, respectively), has been observed in addition to the reduction in the office blood pressure (186 ± 19/108 ± 13 to 135 ± 13/88 ± 7 mmHg, at baseline and at 6 months after denervation). Improvement in albuminuria was also reported after catheter-based renal denervation in resistant hypertensive patients [25].
In animal experiments, Campese et al. reported that renal afferent denervation (dorsal rhizotomy) improved the NE turnover rate in posterior and lateral hypothalamic nuclei of chronic renal failure rats [26] and prevented the progression of renal failure [27]. These results indicate that renal afferent nerves play an important role in the development of hypertension and the progression of renal disease in renal failure model. Therefore, it appears that successful catheter-based renal denervation has a potential not only to improve hypertension but also slow progression of CKD.
CKD and Hypertension as Major Cardiovascular Risk Factors
Since the introduction of the concept of cardiorenal syndrome, CKD has been recognized as a risk factor of CVD, rather than just a precursor of end-stage renal disease (ESRD). Go et al. reported the graded association observed between a reduced estimated GFR and the risk of death, cardiovascular events, and hospitalization in a large, community-based population [28].
As a clinical manifestation of CKD, proteinuria and albuminuria are not only reliable markers of progression of CKD but also stimulate progression of CKD [29]. In the RENAAL and LIFE studies, the improvement of albuminuria was also associated with the reduction in the incidence of CVD [30, 31]. Accordingly, improvement in proteinuria and albuminuria are potentially important in the therapy of CKD, not only from the viewpoint of CVD prevention, but also regression of CKD [32, 33].
Increased CVD risk in CKD is predominantly attributable to the development of atherosclerosis [34]. The frequent comorbidities of CKD listed in Table 22.1 (classic risks), such as hypertension, diabetes, and dyslipidemia, are also well known as risk factors for atherosclerosis. Because of those comorbidities, CKD had not been identified as an independent risk factor of CVD. Recently, however, albuminuria and hyperhomocysteinemia have been characterized as CKD-specific risk factors (nonclassic risks) and showed that they are independently associated with the development of atherosclerosis [35–37].
Table 22.1
Classic and nonclassic risk factors for
Classic risks | Nonclassic risk |
---|---|
Age | Albuminuria |
Gender: male | Hyperhomocysteinemia |
Hypertension | Lipoprotein, apoprotein |
Low HDL | Low production of nitric oxide |
Diabetes mellitus | Electrolyte abnormality |
Lack of exercise | Oxidative stress |
Menopausal | Inflammation |
Familial history of CVD | Somnipathy |
Left ventricular hypertrophy | Hemostatic/fibrinolytic abnormality |
Atherosclerosis also links CKD with hypertension. Through the development of atherosclerosis, CKD accelerates vascular remodeling which coincides with endothelial dysfunction. The vascular abnormalities increase systolic BP and pulse pressure, resulting in high cardiovascular mortality (Fig. 22.3). On the other hand, hypertension accelerates the progression of CKD. The risk of ESRD increases by 20–30 % with every 10 mmHg increase in systolic blood pressure [38]. In fact, a number of clinical studies suggest the strong relation between hypertension and CKD [18, 19].


Fig. 22.3
Schematic relationship between CKD and CVD mechanisms
As shown in Table 22.1, CKD and CVD have a lot of risk factors in common. Slowing progression of CKD therefore presents a means to reduce CVD morbidity and mortality. Indeed, the sub-analysis of HOPE study revealed that while angiotensin-converting enzyme (ACE) inhibitors reduced the events of CVD in patients with CKD to a similar extent to that in non-CKD patients [39, 40], the effect was more pronounced in more severe stages of CKD.
Improvement of albuminuria is potentially important to prevent the progression of CKD, as well as CVD, in the early stage. A recent study by Ott et al. showed that catheter-based renal denervation reduced micro- and macroalbuminuria in patients with treatment-resistant hypertension [25], demonstrating the crucial role of sympathetic hyperactivity in both hypertension and CKD.
Sympathetic Nervous System and CKD
Elevated sympathetic nervous activity is well known to play a key role in cardiovascular complications in humans [41]. Although sympathetic hyperactivity has been consistently demonstrated in ESRD, it is already present from the early stage of renal disease [20, 42]. Urinary albumin excretion was positively correlated with the elevated plasma norepinephrine level in a cross-sectional study of 495 subjects from the general population [43]. The elevated plasma norepinephrine level may be associated with future renal injury in normotensive subjects [20], and higher plasma norepinephrine is an established prognostic predictor in patients with chronic heart failure [44]. As a treatment of hypertension associated with CKD, both metoprolol and enalapril decreased microalbuminuria in patients with type I diabetes mellitus [45]. Regarding the underlying mechanisms, Rao et al. proposed adrenergic mediation of early glomerular permeability from the study of adrenergic polymorphism and genetic predisposition [46]. These findings suggest that sympathetic nervous activation precedes early glomerular permeability alterations [21]. Modulation of overactivity of renal nerves might therefore prevent progression of CKD. The question remains, however, which nerve pathway (afferent or efferent or both) plays the key role in sympathetic hyperactivity in CKD.
The Specific Role of Renal Sympathetic Nerves in Hypertension and CKD
The renal sympathetic nerves consist of efferent sympathetic fibers and afferent sensory fibers [47]. The renal efferent nerves supply all renal relevant structures including the renal vasculature, the tubules, and the juxtaglomerular apparatus with direct physiological control from the central sympathetic nervous system [47]. Accordingly, sympathetic activation of renal efferent nerves results in (i) urinary sodium and water retention via enhanced tubular sodium reabsorption, (ii) reduction in renal blood flow and GFR through neurally mediated vasoconstriction, and (iii) release of renin by stimulation of β1-adrenoceptors on the juxtaglomerular apparatus with concomitant engagement of the renin-angiotensin-aldosterone system [48–50].
The renal afferent sensory nerves are predominantly located in the renal pelvic wall [1, 51]. In contrast to renal efferent nerves, afferent nerves project to the ipsilateral dorsal root ganglia at T6–L2 level with the majority of the nerve cell bodies being at the T9–L1 level. Being processed in the paraventricular nuclei in the brain, stimuli of renal afferent nerves end up deteriorating baroreceptor sensitivity, vagal function, and endothelial function [52, 53]. Increase in renal afferent activity is also known to decrease renal efferent activity through the powerful negative feedback control of renal sympathetic activity via the activation of renorenal reflexes [51, 54, 55]. In contrast, increase in renal efferent activity increases renal afferent activity [56]. In experiments of rats, Kopp et al. showed that renal afferent denervation (efferent nerve remains intact) increased mean arterial pressure in rats [57], suggesting tonically active renal afferent nerves contributing to the maintenance of low efferent renal nerve activity. On the other hand, Campese et al. reported renal afferent denervation prevents the progression of hypertension in rats with chronic renal failure [26], indicating neurally mediated mechanisms of hypertension through renal afferent impulses from the kidneys of uremic rats.
In the context of neural control of renal function, as well as renal contribution to systemic sympathetic tone, neuromodulation therapy is theoretically beneficial in the treatment of hypertension with CKD. The overall goal of renal denervation is to maintain low renal efferent sympathetic nervous activity to achieve sustained reduction in blood pressure. In addition, renal afferent denervation contributes to maintain low systemic sympathetic activity in renal failure model. As summarized in Fig. 22.4, interaction of the kidney and brain involves multiple factors. Indeed, the etiology of hypertension tends to be multifactorial especially when accompanied by CKD. However, sympathetic overactivity is the fundamental hallmark of the disease. Given the abovementioned rationale of renal denervation, hypertensive patients with CKD would be expected to experience benefits from catheter-based renal denervation.


Fig. 22.4
Pathophysiological interactions between the brain and kidney increase total body sympathetic activity
Renal Denervation and Heart Failure and Electrophysiological Disorders
Among the various conditions of sympathetic activation, heart failure plays a crucial role in cardiovascular outcomes and is very common in CKD [44, 58]. Remarkably, sympathetic hyperactivation is known to occur in the early stage of asymptomatic heart failure with both preserved and reduced ejection fraction [59]. Given the role of renal denervation in sympathetic hyperactive condition, renal denervation may possibly improve the outcomes of patients with heart failure. Surgical renal denervation was shown to improve the LV function of heart failure Wistar rats induced by myocardial infarction [6]. Surgical renal denervation also restored natriuresis in response to atrial natriuretic peptide (ANP) in experimental ischemic heart failure dogs [60].
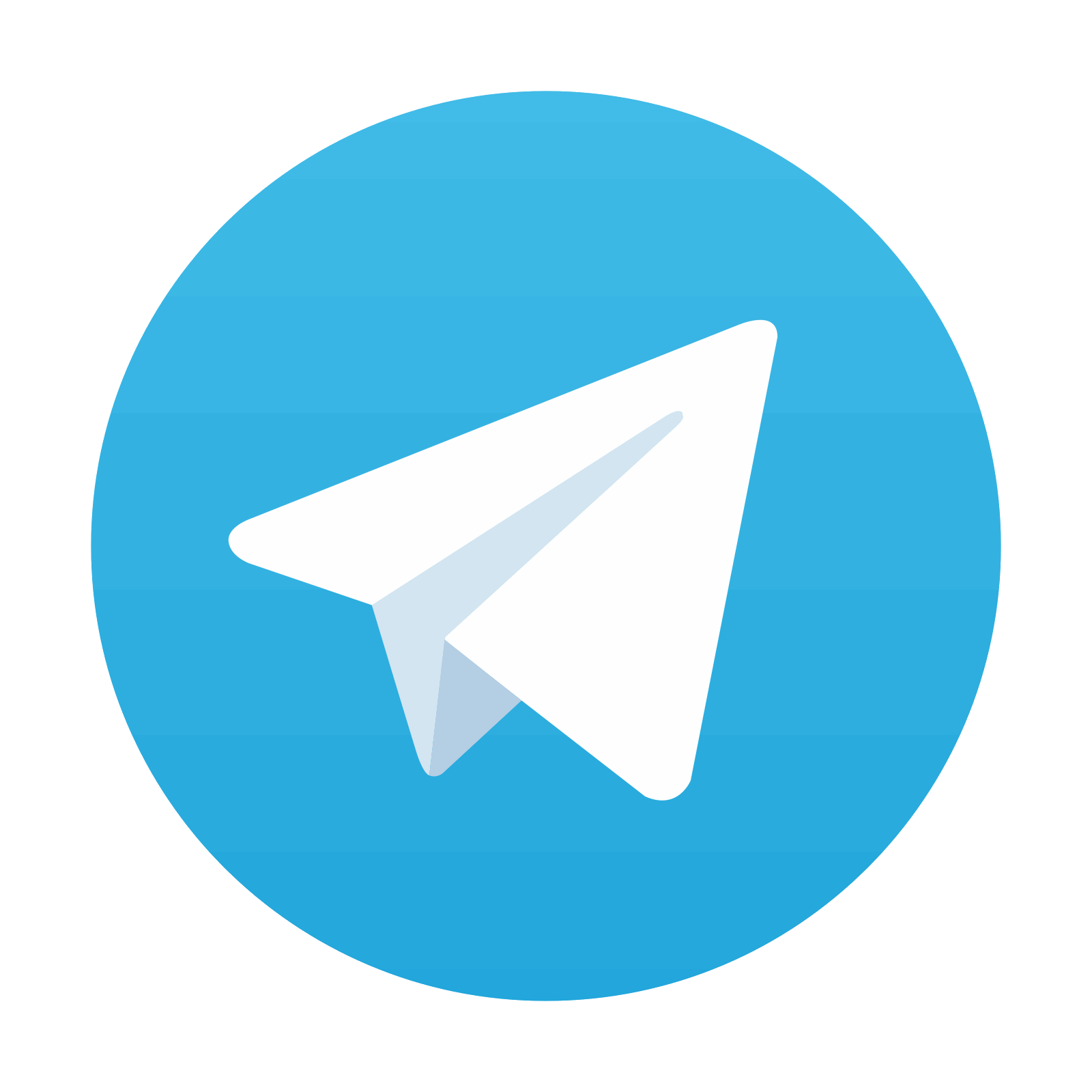
Stay updated, free articles. Join our Telegram channel

Full access? Get Clinical Tree
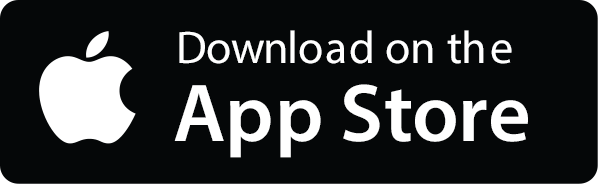
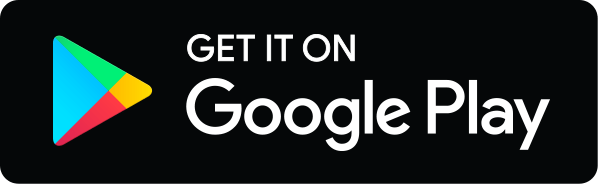