Fig. 2.1
Four afferent pathways that trigger the emetic reflex (green). These pathways converge on the nucleus tractus solitarius (NTS) in the lower hindbrain. The neural circuitry of the caudal hindbrain is sufficient to produce emesis [32–34]; potential forebrain pathways for nausea are shown for comparison to emetic pathways (based on Napadow et al. [96])
Consistent with the idea of convergence of emetic inputs, emetic treatments, including gastric irritants, provocative motion, and circulating toxins, produce activation of the NTS, as measured by c-Fos protein immunohistochemistry [22–27]. Immunohistochemistry for c-Fos is used by neuroscientists to anatomically localize neuronal activation in histological sections of brain tissue [28, 29]. Nonetheless, it must be recognized that most (if not all) physiological stress stimuli produce c-Fos expression in the NTS [30, 31]; therefore, the use of the c-Fos technique is limited in specificity. Other limitations of the c-Fos approach include a lack of temporal resolution (activation occurs as an aggregated response over tens of minutes) and not all cells show c-Fos expression when stimulated.
Where Is the Location of the Emetic Central Pattern Generator?
Often the locus of neurons that generate emesis is called the “vomiting center,” but we lack information on the precise location and phenotypes of these cells. On the other hand, it is clear that the caudal hindbrain contains this critical emetic circuitry; an isolated hindbrain, surgically transected from the forebrain, can produce fictive emetic episodes (an emetic pattern of neural and muscle responses) when stimulated in animal physiology experiments [32–34]. The neurons that produce emesis, the “final common pathway,” are likely distributed among many other types of hindbrain neurons; indeed, the caudal hindbrain contains a highly overlapping network of functional visceral organ control systems (e.g., respiration, cardiovascular control, etc.) [35]. In contrast to a “vomiting center,” the emetic neural network can be conceptualized as a central pattern generator (CPG). A CPG is a network of neural connections that produces rhythmic motor patterns. The initiating signal for the emetic CPG is likely from the NTS because this site integrates input from the four sensory inputs that produce emesis (see Fig. 2.1).
Components of the emetic CPG, which are downstream from the NTS, are controversial. Yates and colleagues have provided a succinct recent review [36] and the following is a summary of these components. A necessary requirement for retching and vomiting is patterned motor output to the abdominal and diaphragm muscles, which produces the internal pressure changes essential for emesis. To increase abdominal cavity pressure, it seems reasonable that inspiratory-related neurons in the rostral ventral respiratory group (rVRG) [37] play a key role in vomiting; surprisingly, rVRG neurons are inhibited during emesis [38, 39]. Retrograde neuroanatomical tracing from the diaphragm and abdominal muscles, using transneuronal transport of viruses, has revealed a region in the medial medullary reticular formation (MRF) that provides polysynaptic input to these muscles [40–45]. Indeed, individual MRF neurons supply input to both abdominal and diaphragm muscle groups [41]; and, lesions of the MRF abolish emesis [46]. Furthermore, neurons in the caudal ventral respiratory group (cVRG) are synchronously active with emesis; potentially both cVRG and MRF neurons supply input to the spinal pathways that produce emesis [36]. The intermediate link between these two areas and the NTS is suggested to be the lateral tegmental field (LTF), which receives input from the NTS [36].
What Are the Mechanics of Vomiting?
Motor outputs controlling the mechanics of a successful emetic episode are associated with three temporal phases (see Fig. 2.2). In Phase 1 – the prodrome, efferent vagal neurons, presumably from the dorsal motor nucleus (DMN), initiate a “giant retrograde contraction” that starts in the mid-intestine and functions to return luminal contents to the gastric compartment, which relaxes proximally [7, 47]. In Phase 2 – retching, spinal efferents produce abdominal, crural diaphragm (a medial muscle close to the esophagus), and costal diaphragm (lateral muscle) contractions that cause pressure increases in the abdominal cavity to position the gastric luminal contents under the distal esophagus for the next phase, expulsion or vomiting [48]. Finally, in Phase 3 – vomiting, similar in some aspects to Phase 2, spinal efferents produce abdominal and diaphragmatic muscle contractions but the crural diaphragm is not activated (see Fig. 2.2); consequently, gastric contents freely flow up and out the esophagus (expulsion) [49].


Fig. 2.2
The three phases of emesis, with movement of gastrointestinal contents (red arrows): (1) prodromal responses, a giant retrograde contraction from the middle of the small intestine; (2) retching; and (3) vomiting, expulsion of gastric contents. Up and down blue arrows indicate pressure changes caused by muscular contractions of the diaphragm and abdomen. Plus signs (black) signify electromyographic (EMG) responses from the crural and costal diaphragm and abdominal muscles (see reviews [61, 97])
Which Drug Therapies Control Emesis?
This section summarizes current antiemetic therapies; for an in-depth analysis, the reader is referred to Chap. 9 in this book and several extensive reviews [50–53]. Antiemetic drug targets are listed in Table 2.1, along with effects on known emetic sensory pathways (see Fig. 2.1); only established, and pathway-specific, emetic stimuli are included: (1) intragastric copper sulfate (CuSO4, a gastric irritant) or cisplatin (intravenous or intraperitoneal injection; an acute response) activates GI vagal afferents [54–56]; (2) systemic injections of nicotine or apomorphine stimulate the area postrema [57, 58], and exposure to provocative motion acts on the vestibular system to produce emesis [59].
Table 2.1
Antiemetic drugs
Target | Examples of stimulus effects | |
---|---|---|
Receptor antagonists | H1 | Motion [99] |
M(3/5?) | ||
5-HT3 | ||
NK1 | ||
Nicotine [106] | ||
Apomorphine [107] | ||
Morphine [102] | ||
Receptor agonists | CB1 | |
Nicotine [109] | ||
Apomorphine [110] | ||
Morphine [111] | ||
Motion [112] | ||
5-HT1A | CuSO4 [114] | |
Cisplatin [114] | ||
Nicotine [114] | ||
Motion [113] |
Current, clinically available, antiemetics target histamine type 1 (H1), muscarinic (M), neurokinin 1 (NK1), and serotonin type 3 (5-HT3) receptors [50–53]. NK1 receptor antagonists provide inhibitory control of emesis produced by a wide range of emetic stimuli, i.e., broad-spectrum antiemetic control; this supports the idea of convergence of vagal, area postrema, vestibular, and psychogenic sensory pathways to activate a final common pathway for emesis [60, 61]. In general, H1 and M receptor antagonists are less effective for controlling emesis in comparison to NK1 receptor antagonists. H1 and M antagonists have inhibitory effects on motion sickness and postoperative vomiting [16, 52], possibly due to direct effects on the vestibular nuclei or area postrema. In contrast, 5-HT3 receptor antagonists are not effective for inhibiting motion sickness [62, 63] but are routinely used to inhibit chemotherapy-induced and postoperative vomiting [64]. The location of 5-HT3 receptors responsible for antiemesis is unclear because these receptors are located on vagal afferent fibers in the GI tract and their terminal inputs in the NTS [65].
The need to control emesis produced by cytotoxic cancer chemotherapy has resulted in significant research efforts, ultimately leading to large clinical trials [66]. The fruits of this work led to the development of 5-HT3 and NK1 receptor antagonists (e.g., Zofran and Emend, respectively, and many similar agents). The physiology of chemotherapy-induced vomiting, particularly with the use of cisplatin and cyclophosphamide agents, has traditionally been divided into acute (up to 24 h) and delayed (greater than 24 h after injection) responses [67]; evidence indicates 5-HT3 receptor antagonists are effective for controlling the acute phase, whereas NK1 receptor antagonists are most effective in the delayed phase, albeit in combination with administration of dexamethasone [67]. To some extent, the successes achieved in the control of chemotherapy-induced vomiting, using 5-HT3 and NK1 receptor antagonists, have been applied to postoperative vomiting [52]. Postoperative vomiting is generated by dual activation of emesis with inhalational anesthesia and opioids, presumably at sites in the caudal hindbrain [68].
Why Do Some Animals Lack a Vomiting Reflex Circuit?
The presence of the emetic reflex is widespread among mammals. This response is present in several major lineages (see Fig. 2.3), including carnivora (e.g., cat, dog, ferret [86–91]), primates (e.g., human, monkey [82, 83]), Cetartiodactyla (e.g., pigs [84, 85]), and Eulipotyphla (e.g., shrews [69–71]). The reflex appears to be absent in Rodentia (e.g., laboratory rats and mice) and Lagomorpha (e.g., rabbits and hares) [12]. In Horn et al., we tested representative members of the three main groups of rodents (mouse-related, Ctenohystrica, and squirrel-related) and none were found to have emetic responses to apomorphine, CuSO4, or veratrine (a plant alkaloid) [34]. Using an isolated perfused brainstem preparation, we also showed that laboratory rats and mice lack any coordinated neural efferent activity that would indicate emesis, compared to musk shrew controls [34].
If vomiting is essential for survival, why do rodents lack this reflex? Current theory suggests that other behavioral responses of rodents have replaced emesis, for example, conditioned taste aversion (CTA) and pica (ingestion of clay) (see review [72]). CTA is a classically conditioned response, formed by association of a flavor with visceral sickness, which leads to avoidance of the flavor in the future [73]. In this regard, rodents might only nibble on a small amount of tainted food, not a lethal dose but adequate to produce a CTA. On the other hand, pica is believed to be a way to dilute the effects of a toxin entering the GI tract. Silicate clay (kaolin) can bind toxins [74, 75] and kaolin intake in rats is associated with emetic treatments, including cytotoxic chemotherapy, provocative motion, and apomorphine treatments [76–79]. The pica response can also be inhibited by treatment with antiemetics, such as NK1 and 5-HT3 receptor antagonists [80, 81].
Laboratory rats and mice are used for most neuroscience experiments, particularly because of the availability of powerful genetic, physiological, and anatomical techniques; however, an absent emetic reflex in these animals has led to the use of dogs, cats, primates, pigs, and shrews to study the neurobiology of emesis [10].
Conclusion
In summary, the emetic reflex is a “complex” patterned response of several neurological components, essentially engaging the movements of a large part of the torso. We still lack an understanding of why rodents (and lagomorphs) do not have this response, but an absent neurological circuit in the emetic CPG is suspected since the brainstem of these species appears to have no emetic-related motor output in response to emetic stimulation [34]. The four sensory pathways that trigger the emetic reflex are relatively known; however, there is sparse information on the coding of emetic signals by GI vagal afferents [92]. Furthermore, there is little insight into the nature of descending pathways from the forebrain that participate in cognitive and learned emetic responses. There is also limited information on the detailed components of the emetic CPG; existing data has focused on c-Fos measures, ablation techniques, and single cell electrophysiological recordings. We will need more precise and high-throughput tools, such as optogenetic control of specific cells and multi-electrode technology to determine the details of the CPG. Although research indicates good control of acute chemically-induced vomiting with current antiemetics, for example, chemotherapy and postoperative vomiting, we lack effective therapies for chronic vomiting (and nausea); this is particularly true in cases of gastroparesis, cyclic vomiting syndrome, and hyperemesis gravidarum (an extreme form of pregnancy-related nausea and vomiting) [93–95]. These issues require more research on the physiology of vomiting to develop novel and effective therapies.
References
1.
Fearing F. René Descartes. A study in the history of the theories of reflex action. Psychol Rev. 1929;36:375–88. doi:10.1037/h0074868.CrossRef
2.
Sherrington SCS. The integrative action of the nervous system. New Haven, CT, USA: Yale University Press; 1906.
3.
Horn CC. Why is the neurobiology of nausea and vomiting so important?. Appetite. 2008;50:430–4. S0195-6663(07)00365-0 [pii].doi:10.1016/j.appet.2007.09.015.
4.
Davis CJ. Emesis research: a concise history of the critical concepts and experiments. J R Nav Med Serv. 1997;83:31–41.PubMed
5.
Bischoff SC, Renzer C. Nausea and nutrition. Auton Neurosci Basic Clin. 2006;129:22–7. doi:10.1016/j.autneu.2006.07.011.CrossRef
6.
CDC. Estimates: findings | estimates of foodborne illness. CDC. 2011. http://www.cdc.gov/foodborneburden/2011-foodborne-estimates.html. Accessed 23 Dec 2015.
7.
8.
9.
10.
11.
Hu DL, Zhu G, Mori F, et al. Staphylococcal enterotoxin induces emesis through increasing serotonin release in intestine and it is down regulated by cannabinoid receptor 1. Cell Microbiol. 2007;9:2267–77. doi:10.1111/j.1462-5822.2007.00957.x.CrossRefPubMed
12.
Minami M, Endo T, Hirafuji M, et al. Pharmacological aspects of anticancer drug-induced emesis with emphasis on serotonin release and vagal nerve activity. Pharmacol Ther. 2003;99:149–65. doi:10.1016/S0163-7258(03)00057-3.CrossRefPubMed
13.
Lang PM, Grafe P. Chemosensitivity of unmyelinated axons in isolated human gastric vagus nerve. Auton Neurosci Basic Clin. 2007;136:100–4. doi:10.1016/j.autneu.2007.05.001.CrossRef
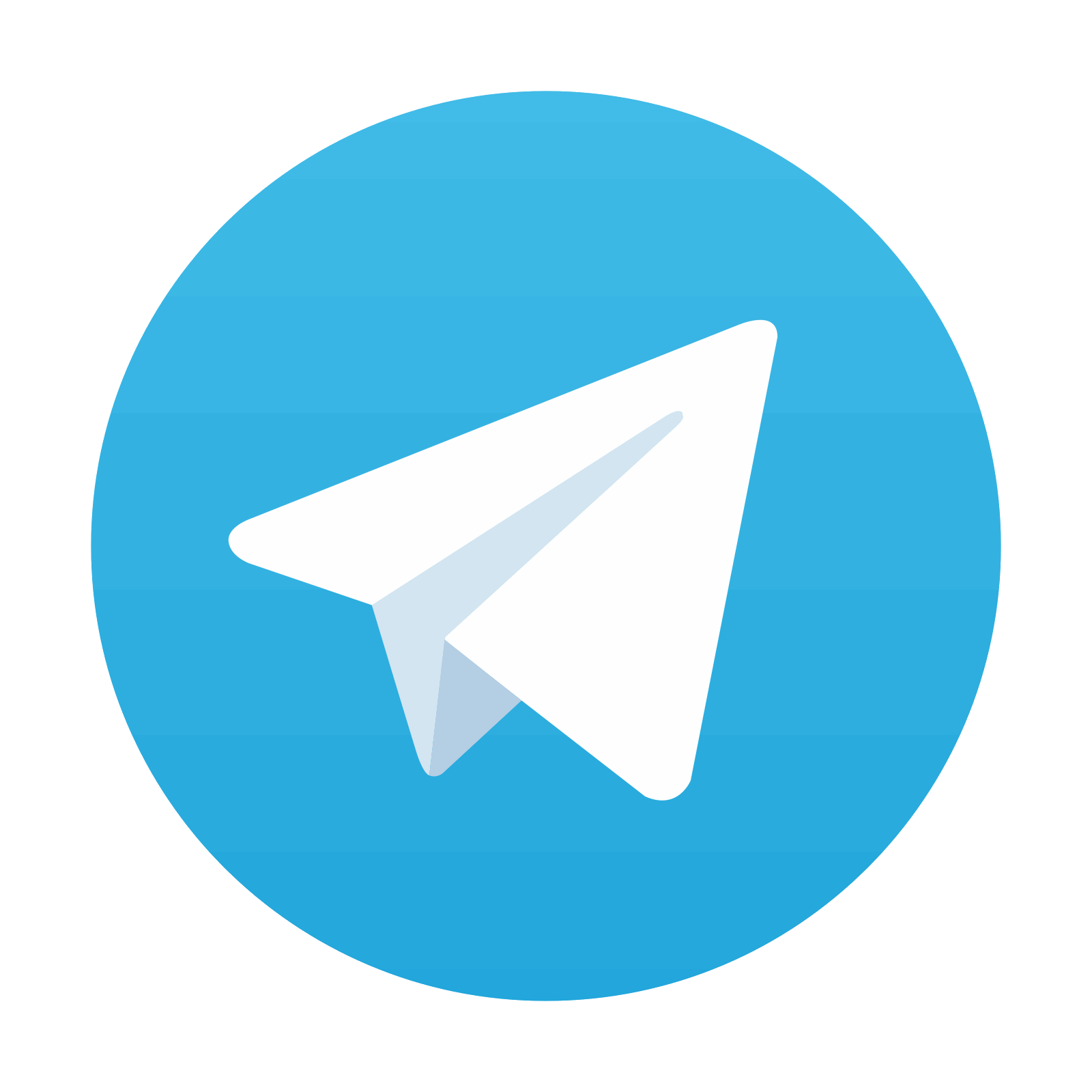
Stay updated, free articles. Join our Telegram channel

Full access? Get Clinical Tree
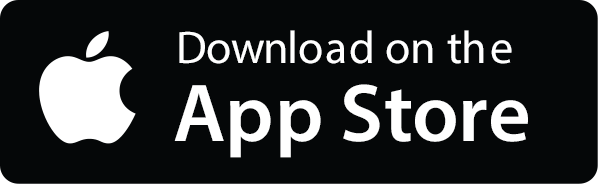
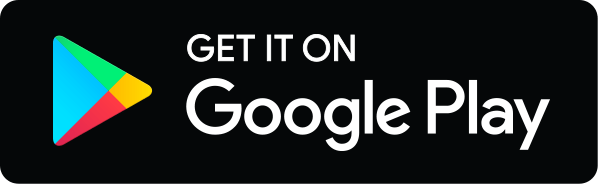