The Patient with an Acid-Base Disorder
William D. Kaehny
I. Acid-base disorders are the chest pains of the body fluids. They are important signs of disorders that have deranged physiology. Occasionally, acid—base disorders disrupt homeostasis sufficiently to move the arterial pH into a dangerous range (less than 7.10 or greater than 7.60). Depending on the overall status of the patient and the response of the cardiovascular system, the pH level may require direct attention. After the clinician detects the presence of an acid-base disorder from clinical and laboratory clues, he proceeds logically through a progression of steps to optimal management of the patient.
A. Step 1. Measure pH. This identifies acidemia or alkalemia. The change in bicarbonate and partial pressure of CO2 (Pco2) indicates whether the primary process is metabolic or respiratory.
B. Step 2. Check the compensatory or secondary response of the Pco2 or
to see if the disorder is simple or mixed.

C. Step 3. Calculate the serum anion gap (AG) to screen for an increase in organic anions such as lactate. Add any increase in AG (ΔAG) that is potential
to the serum total carbon dioxide content (tCO2) to screen for a hidden metabolic alkalosis.

D. Step 4. Determine the cause of the acid-base disorder from the clinical setting and laboratory tests.
E. Step 5. Treat the underlying disorder, unless the pH is dangerous either acutely or chronically (such as acidosis affecting bone).
II. WHEN TO SUSPECT ACID-BASE DISORDERS
A. Clinical. The underlying cause of the acid-base disorder is most frequently responsible for a patient’s signs and symptoms. Certain clinical settings and findings should alert the clinician to the likelihood of an acid—base disor der. Coma, seizures, congestive heart failure, shock, vomiting, diarrhea, and renal failure generate changes in the Pco2 or
levels. Marked changes in the pH occasionally may cause direct clinical manifestations. Severe alkalemia causes an irritability of heart and skeletal muscle. Severe acidemia causes a depression of heart pump function and vascular tone. Although central nervous system dysfunction appears frequently with acid—base disorders, changes in pH do not appear responsible. Rather, altered plasma osmolality and Pco2 appear to be the causative agents.

B. Laboratory. A thoughtful measurement of serum electrolytes in patients with abnormal losses or gains of body fluids is good practice. An abnormal
serum tCO2 is definite evidence of an acid—base disorder; an abnormal serum AG is very suggestive; an abnormal serum potassium is suspicious.
serum tCO2 is definite evidence of an acid—base disorder; an abnormal serum AG is very suggestive; an abnormal serum potassium is suspicious.
1. Serum tCO2. The
in blood can be estimated reasonably by measuring the tCO2 in venous serum. The serum tCO2 is 1 to 3 mmol/L greater than the arterial
because it is from venous blood, which has more
, and it includes dissolved CO2 and trivial amounts of other substances. Normal sea level serum tCO2 levels average 26 to 27 mmol/L. A value below 24 or above 30 likely marks a clinical acid—base disorder. An acid—base disorder of the mixed type may exist with a normal serum tCO2.



2. The serum AG is calculated from the venous serum sodium, chloride, and tCO2:

The units are in mEq/L, because this calculation estimates the charge difference between the so-called unmeasured anions (serum total anions represented by Cl– and
) and unmeasured cations (total cations represented by Na+). The average normal value is 9 ± 3 mEq/L, but varies in different laboratories. Albumin contributes most to the AG. A fall in serum albumin of 1 g/dL from a normal of 4.4 decreases the AG by 2.5 mEq/L. Importantly, the corrected serum AG includes this calculation.

a. Metabolic Acidosis due to an organic acid such as lactic or acetoacetic acid is marked by an increased AG. An increase in the AG of 8 mEq/L to 17 or higher usually indicates the presence of organic acidosis, although at times the exact anion may not be identified. The anion of the organic acid replaces the
lost in the buffering of the hydrogen ion (H+) part of the acid and therefore increases the unmeasured anions. Importantly, a normal or slightly elevated AG does not rule out the presence of organic metabolic acidosis, such as diabetic ketoacidosis, because a patient with good renal perfusion and ample urine flow may excrete the ketoanions at a rate sufficient to keep the serum AG from rising markedly.

b. Metabolic Alkalosis. At times, a metabolic acidosis that increases the AG and lowers the
may coincide with a process that generates a metabolic alkalosis. For example, vomiting that generates a high
may be caused by diabetic ketoacidosis, which lowers the
. In this case, the serum tCO2 (and arterial
) may be low or normal despite the elevating action of the metabolic alkalosis. The clue to the presence of such hidden metabolic alkalosis is derived in a Holmesian manner by adding the measured serum tCO2 and the ΔAG (measured AG — 9). If this sum is greater than 30 mEq/L, a hidden metabolic alkalosis is likely present. The ΔAG is a marker of “lost” or potential
, that which was titrated by the H+ of an organic acid. Pure metabolic alkalosis may directly increase the AG by up to 5 mEq/L due to effects on the albumin concentration and charge.





3. Serum Potassium. Potassium metabolism is linked to acid-base metabolism at the levels of cell shifts, renal tubular functions, and gastrointestinal transport. Therefore, an abnormal serum potassium concentration alerts the clinician to the likelihood that an acid—base disorder is also present.
III. IDENTIFYING THE MAJOR ACID-BASE DISORDERS.
When the clinician suspects that an acid—base disorder might be present and that patient management might be adjusted, a set of acid—base variables should be obtained: pH, Pco2, and
.

A. Chemistry and Physiology of Acid-Base. Cellular, tissue, and organ systems apparently function best at an extracellular fluid (ECF) pH of approximately 7.40. Intracellular fluid (ICF) pH is heterogeneous within the cell, depending on organelles and metabolic activity, but averages approximately 7.00. ECF buffer molecules bind or release H+ to keep pH close to 7.40 in the face of gain or loss of acids or bases.
Current clinical acid—base chemistry is based on the Brønsted—Lowry theory which designates acids as proton donors and bases as proton acceptors. The three key elements are the hydrogen ion activity (pH), carbonic acid (the acid), and bicarbonate (the base). The Pco2 represents the acid in the modified Henderson—Hasselbalch equation. Base excess, used by some, is another concept derived from these elements in an attempt to explain whether alterations in these elements are due to metabolic or respiratory disorders.
Another approach that appears useful in investigative, analytic settings uses the Stewart equations. These calculate the pH from three, so-called independent, variables: Pco2, the strong ion difference, and total weak acid (mainly protein).
1. Blood pH is the mathematical expression of the intensity of acidity or H+ activity. H+ concentration usually is expressed in nmol per L. H+ concentration is 100 nmol/L at pH 7.00 and 40 nmol/L at pH 7.40. The pH is measured at body temperature with a glass, flow-through electrode.
2. The partial pressure of carbon dioxide in blood, Pco2, represents the acid component in blood. The respiratory system determines the level at which the Pco2 is set. Pco2 is measured in whole blood with a pH electrode that detects the change caused by the diffusion of CO2 from the sample into a buffer solution.
3.
is the metabolic component of the acid—base equation, serving as the base in the buffer pair.
concentration is controlled by the buffering state, metabolic processes, and the kidneys.
concentration is calculated from the pH and Pco2 using the Henderson—Hasselbalch equation. The fact that it is calculated makes it no less reliable a value than the serum tCO2.



4. The acid-base equation allows the determination of the state of ECF acid—base balance, the presence of an acid—base disorder, the nature of the disorder, and the presence of a simple or mixed disorder:

Therefore, the pH level depends on the ratio or mathematical relationship between the
and the Pco2. An acid—base disorder is generated by an alteration from normal of either of these two factors. The resultant change in pH results in chemical shifts in the buffers, which mitigate the change in pH somewhat. A physiologic compensatory response occurs in the respiratory system for a metabolic disorder and
in the kidneys for a respiratory disorder. A new steady state ensues, with the new pH set by the new values of the
concentration and Pco2.

in the kidneys for a respiratory disorder. A new steady state ensues, with the new pH set by the new values of the

B. Measurement of Acid-Base Variables. The determination of the acidbase state is usually based on an analysis of arterial blood, although arterialized venous blood analysis is equally valid. After warming the extremity, blood is drawn without air mixing from an artery or from a forearm vein without tourniquet. Although experimental studies show that ICF pH and mixed venous acid—base measurements correlate better with organ function, arterial blood measurements are more easily available and provide a read ily interpretable view of the metabolic state of organs and their function. Keep in mind that tissue hypoperfusion, as in cardiopulmonary arrest or profound shock, makes tissue acidosis worse than that reflected by arterial blood acid—base values.
C. Identification of a Major Acid-Base Disorder. The basis of this approach is to determine the direction (up or down) in which the measured values differ from the arbitrary normal values for pH (7.40), Pco2 (40 mmHg), and
(24 mmol/L). First, determine whether acidemia (pH down) or alkalemia (pH up) is present. Then determine whether the primary generat ing change was in the
or in the Pco2 (Table 4-1). The compensating factor should change in the same direction as the generating factor to yield a simple acid—base disorder.


1. Example of a Simple Disorder. Arterial blood analysis revealed the fol lowing values: pH 7.55,
18 mmol/L, and Pco2 21 mmHg.

a. Step 1. The pH is up. Therefore, alkalemia is present and must be due to an increased
(as in metabolic alkalosis) or to a decreased Pco2 (as in respiratory alkalosis).

c. Step 3. Because the Pco2 is low, it can account for the increased pH; this is respiratory alkalosis.
d. Step 4. The
change is in the same direction as that of the Pco2; this is consistent with compensation and a simple respiratory alkalosis.

2. Example of a Mixed Acid-Base Disorder. Sampling of arterial blood yielded the following: pH 7.55,
30 mmol/L, and Pco2 35 mmHg.

Table 4-1. Simple Acid-Base Disorders | |||||||||||||||||||||||||
---|---|---|---|---|---|---|---|---|---|---|---|---|---|---|---|---|---|---|---|---|---|---|---|---|---|
|
a. Step 1. The pH is up. Therefore, alkalemia is present.
c. Step 3. The Pco2 is low and it, too, can account for an increased pH.
IV. JUDGING WHETHER AN ACID-BASE DISORDER IS SIMPLE OR MIXED.
When an underlying process generates an acid-base disorder by perturbing one member of the
Pco2 buffer pair (remember that Pco2 represents H2CO3), the other partner is adjusted to compensate by the physiologic response of the body and changes in the same direction as the primary partner in order to reduce the magnitude of the change in pH. The time-honored term for this physiologic response is compensation. However, the physiologic response mechanisms may be activated by stimuli other than pH and actually may contribute to the maintenance of the abnormal pH. Therefore, some have termed these responses maladaptive because they are not always truly compensatory. For example, a low Pco2 in response to metabolic acidosis actually causes the kidneys to reduce
reabsorption. Importantly, compensation does not restore the pH exactly to normal, because that would shut off the stimulus for the compensatory mechanism.


A. Steps in Judging Whether an Acid-Base Disorder Is Simple. After the major disorder is identified, determine whether the compensation for the primary event is appropriate.
1. Check Directions of Changes from Normal of
and Pco2. The acid—base buffer pair changes from normal in the same direction in all simple acid—base disorders. If they change in opposite directions, the disorder must be mixed.

2. Compare the Magnitude of the Compensation of the Pco2 or
with the Primary Change in the
or Pco2. In metabolic disorders, the primary change occurs in the
, with the compensation occurring in the Pco2. The opposite is true in the respiratory disorders. Table 4-2 contains guidelines or rules that can be used to judge whether compensation is appropriate. The respiratory disorders have two stages of compensation: acute, when only tissue buffering slightly changes the
, and chronic (after 24 hours), when the kidneys cause major changes in the
concentration. If the measured change in the compensating factor does not approximate the change predicted, a mixed disorder is likely. Two methods of predicting compensation appear in Table 4-2
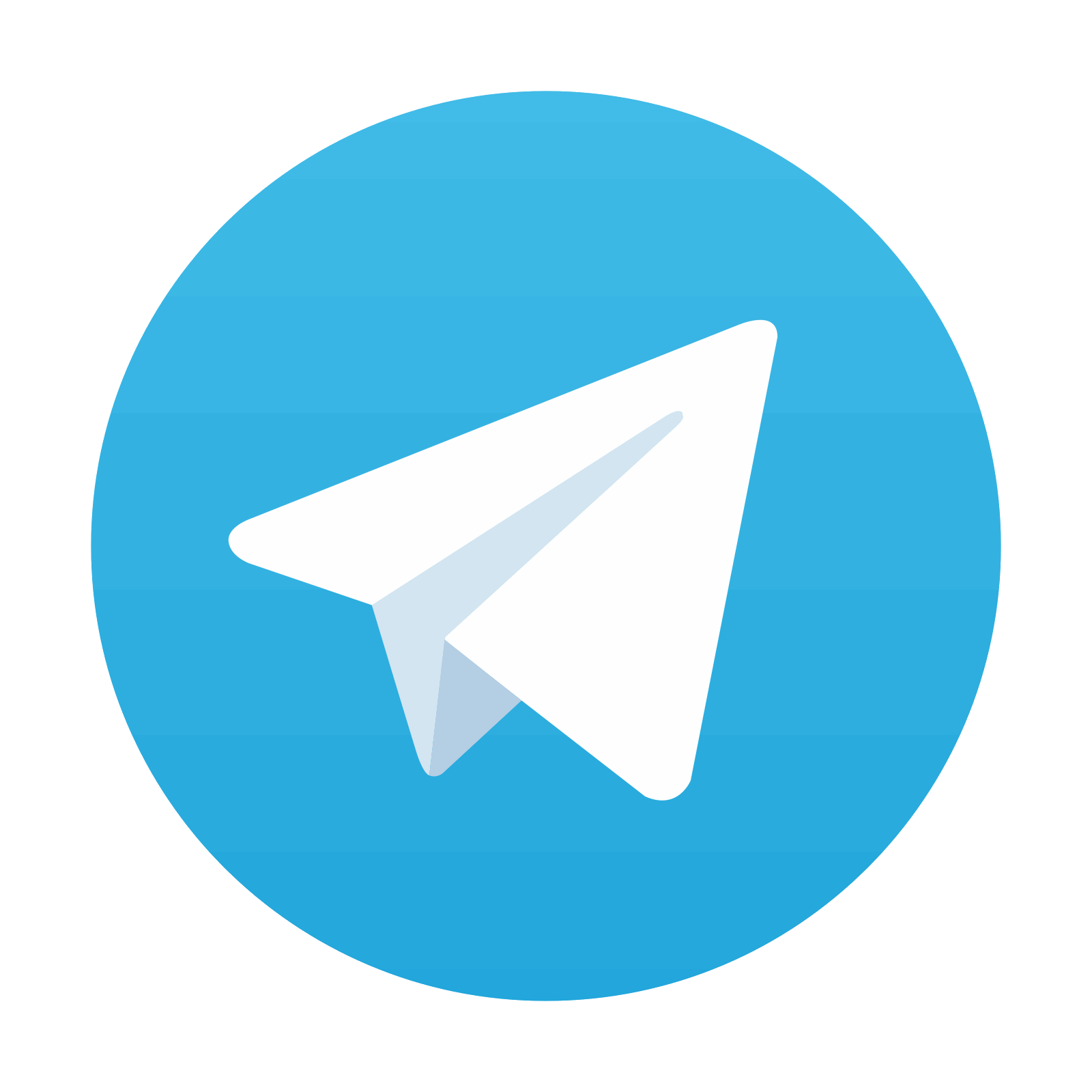





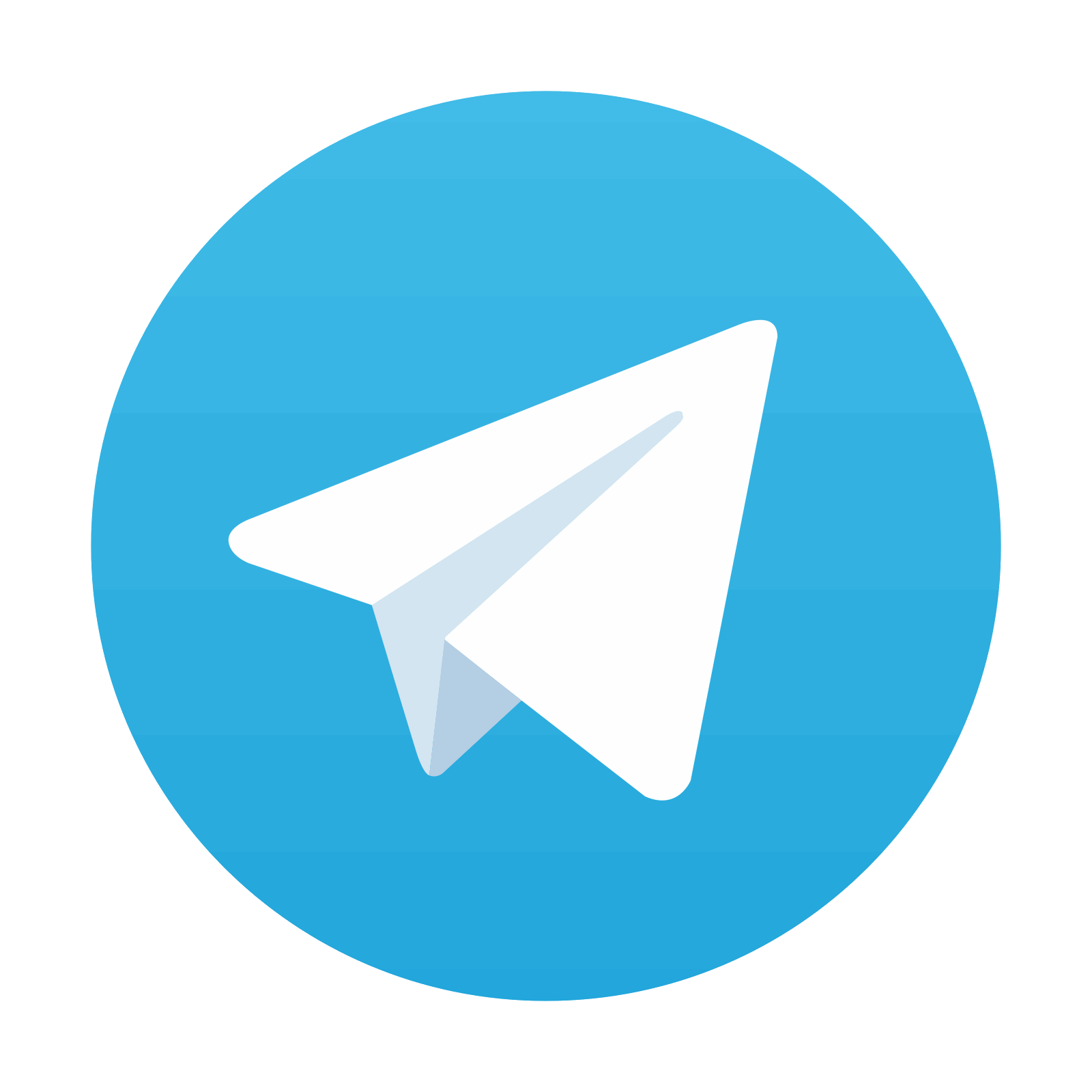
Stay updated, free articles. Join our Telegram channel

Full access? Get Clinical Tree
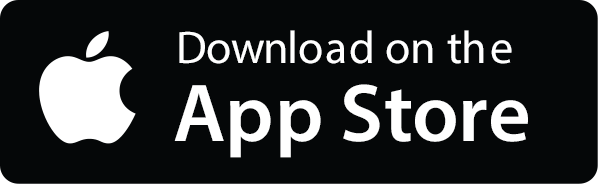
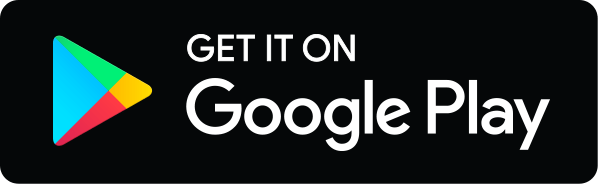