Gut microbes are capable of producing most neurotransmitters found in the human brain. Evidence is accumulating to support the view that gut microbes influence central neurochemistry and behavior. Irritable bowel syndrome is regarded as the prototypic disorder of the brain-gut-microbiota axis that can be responsive to probiotic therapy. Translational studies indicate that certain bacteria may have an impact on stress responses and cognitive functioning. Manipulating the gut microbiota with psychobiotics, prebiotics, or even antibiotics offers a novel approach to altering brain function and treating gut-brain axis disorders, such as depression and autism.
Key points
- •
Gut microbes can communicate with the brain through a variety of routes, including the vagus nerve, short-chain fatty acids (SCFAs), cytokines, and tryptophan.
- •
Psychobiotics are bacteria that when ingested in adequate amounts produce a positive mental health benefit.
- •
The brain-gut-microbiota axis represents a paradigm shift in neuroscience and provides a novel target for treating not only irritable bowel syndrome (IBS) but also conditions, such as depression, autism, and Parkinson disease.
Introduction
The human adult gut contains more than 1 kg of bacteria, essentially the same weight as the human brain. It is generally estimated that the gut is inhabited by 10 13 to 10 14 microorganisms, which is significantly more than the number of human cells in the body, and contains more than 100 times as many genes as in the genome. Amazingly, the genomic and biochemical complexity of the microbiota exceeds that of the brain. Studies of the brain-gut-microbiota axis have been described as a paradigm shift in neuroscience. Increasing evidence points to appropriate diversity in the gut microbiota that is essential not only for gut health but also for normal physiologic functioning in other organs, especially the brain. An altered gut microbiota in the form of dysbiosis at the extremes of life, both in the neonate and in the elderly, can have a profound impact on brain function. Such a dysbiosis might emerge for a variety of reasons, including the mode of birth delivery, diet, and antibiotic and other drug exposure. Given that the brain is dependent on gut microbes for essential metabolic products, it is not surprising that a dysbiosis can have serious negative consequences for brain function both from neurologic and mental health perspectives. Although much of the early data emerged from animal studies, mainly rodent based, there are now an increasing number of human studies translating the animal findings.
This review focuses on the routes of communication between the gut and brain, examines a prototypic disorder of the brain gut axis, explores the ways in which gut dysbiosis may evolve, and provides an up-to-date account of behavioral and neurologic pathologies associated with dysbiosis.
Introduction
The human adult gut contains more than 1 kg of bacteria, essentially the same weight as the human brain. It is generally estimated that the gut is inhabited by 10 13 to 10 14 microorganisms, which is significantly more than the number of human cells in the body, and contains more than 100 times as many genes as in the genome. Amazingly, the genomic and biochemical complexity of the microbiota exceeds that of the brain. Studies of the brain-gut-microbiota axis have been described as a paradigm shift in neuroscience. Increasing evidence points to appropriate diversity in the gut microbiota that is essential not only for gut health but also for normal physiologic functioning in other organs, especially the brain. An altered gut microbiota in the form of dysbiosis at the extremes of life, both in the neonate and in the elderly, can have a profound impact on brain function. Such a dysbiosis might emerge for a variety of reasons, including the mode of birth delivery, diet, and antibiotic and other drug exposure. Given that the brain is dependent on gut microbes for essential metabolic products, it is not surprising that a dysbiosis can have serious negative consequences for brain function both from neurologic and mental health perspectives. Although much of the early data emerged from animal studies, mainly rodent based, there are now an increasing number of human studies translating the animal findings.
This review focuses on the routes of communication between the gut and brain, examines a prototypic disorder of the brain gut axis, explores the ways in which gut dysbiosis may evolve, and provides an up-to-date account of behavioral and neurologic pathologies associated with dysbiosis.
Brain-gut-microbiota communication
The brain-gut-microbiota axis is a bidirectional communication system enabling gut microbes to communicate with the brain and the brain with the gut. Although brain-gut communication has been a subject of investigation for decades, an exploration of gut microbes within this context has only featured in recent years. The mechanisms of signal transmission are complex and not fully elucidated but include neural, endocrine, immune, and metabolic pathways. Preclinical studies have implicated the vagus nerve as a key route of neural communication between microbes of the gut and centrally mediated behavioral effects, as demonstrated by the elimination of central Lactobacillus rhamnosus effects after vagotomy and that humans who have underwent vagotomy at an early age have a decreased risk of certain neurologic disorders. The gut microbiota also regulates key central neurotransmitters, such as serotonin, by altering levels of precursors; for example, Bifidobacterium infantis has been shown to elevate plasma tryptophan levels and thus influence central serotonin (5HT) transmission. Intriguingly, synthesis and release of neurotransmitters from bacteria has been reported: Lactobacillus and Bifidobacterium spp can produce γ-aminobutyric acid (GABA); Escherichia , Bacillus , and Saccharomyces spp can produce noradrenaline; Candida, Streptococcus, Escherichia , and Enterococcus spp can produce serotonin; Bacillus can produce dopamine; and Lactobacillus can produce acetylcholine. These microbially synthesized neurotransmitters can cross the mucosal layer of the intestines, although it is highly unlikely that they directly influence brain function. Even if they enter the blood stream, which is by no means certain, they are incapable of crossing the blood-brain barrier (BBB). Their impact on brain function is likely to be indirect, acting on the enteric nervous system. SCFAs, which include butyrate, propionate, and acetate, are essential metabolic products of gut microbial activity and may exert central effects either through G-protein–coupled receptors, although such receptors are sparsely concentrated in the brain. It is more likely that they act as epigenetic modulators through histone deacetylases. SCFAs are also involved in energy balance and metabolism and can modulate adipose tissue, liver tissue, and skeletal muscle and function. Immune signaling from gut to brain mediated by cytokine molecules is another documented route of communication. Cytokines produced at the level of the gut can travel via the bloodstream to the brain. Under normal physiologic circumstances, it is unlikely that they cross the BBB, but increasing evidence indicates a capacity to signal across the BBB and to influence brain areas, such as the hypothalamus, where the BBB is deficient. It is through the latter mechanism the cytokines interleukin (IL)-1 and IL-6 activate the hypothalamic-pituitary-adrenal (HPA) axis, bringing about the release of cortisol. This is the most potent activator of the stress system.
The HPA axis, which provides the core regulation of the stress response, can have a significant impact on the brain-gut-microbiota axis. It is increasingly clear and probably of relevance in several pathologic conditions that psychological or physical stress can significantly dysregulate the HPA axis and subsequently the brain-gut-microbiota axis, for example, in IBS ( Fig. 1 ).
Multiple lines of approach have been used to interrogate the brain-gut-microbiota axis, especially in animal model systems; these include the use of germ-free animals, potential probiotic agents, antibiotics, animals exposed to pathogens and the use of stress to determine the effects of dysregulating the axis. The largest naturalistic study of a gut pathogen and the impact on the brain-gut axis was as a result of the Walkerton catastrophe. The contamination of the Walkerton (Walkerton, Ontario, Canada) water supply occurred in 2000 claimed 7 lives and left more than 2000 people ill. The E coli outbreak was caused by farm runoff contaminating the town’s water supply. Those infected had significant risk of developing postinfective IBS and many had comorbid depression/anxiety. To a greater extent than any prior study, this natural disaster provided clear cut support for the notion of postinfective IBS.
Brain-gut-microbiota axis and extremes of life
The intestinal microbiota of newborn infants is characterized by low diversity and a relative dominance of the phyla Proteobacteria and Actinobacteria in the early postnatal period, a time at which there is enormous brain development. With the passage of time, the microbiota becomes more diverse, with the emergence and dominance of Firmicutes and Bacteroidetes. Full-term, vaginally delivered babies born to healthy mothers who are breastfed and nonantibiotic treated have an optimal development of the neonatal microbiota. The characteristic intestinal microbiota observed in healthy full-term infants is disturbed in preterm infants, who are frequently delivered by caesarean section, receive antibiotics, and may have problems feeding. Furthermore, preterm infants possess a functionally immature gut with low levels of acidity in the stomach, due to insufficient gastric acid secretion and their requirement for more frequent feeding. These events lead to an increase in the prevalence of potentially pathogenic bacteria in the gastrointestinal (GI) tract and less microbial diversity than full term infants. The extent to which these features play a role in the development of cerebral palsy and subsequent autism are the subject of research and ongoing debate. What is clear is that complex brain maturation and the increasing sophistication of the gut microbiota are highly correlated. To date many assumptions are based on correlational data from which a causative impact cannot be conclusively concluded.
When the microbiota composition of elderly people in nursing homes are compared with those in the community, large-scale differences are detected. Those in nursing homes have a far less diverse microbiota and this has been attributed to a less varied diet. It is possible, however, that pathologic factors that lead to admission into nursing homes, such as deteriorating cognitive function and less physical activity, might play an important role in the decreased microbial richness and not the less diverse diet. Ongoing studies should clarify this issue, and there is a challenge for the food industry to produce diets for the elderly that help to sustain microbial diversity.
What is abundantly clear is that a dysregulated gut microbiota either in early childhood or in an aging population significantly increases the likelihood of brain dysfunction. The precise relationship between these observations is far from understood. Determining the mechanisms and pathways underlying microbiota-brain interactions may yield novel insights into individual variations and perhaps enable the development of new treatments for a range of neurodevelopmental and neurodegenerative disorders, ranging from autism to Parkinson disease.
Irritable bowel syndrome as prototype
IBS is the prototypic disorder of the brain-gut-microbiota axis, generally perceived as a having a biopsychosocial etiology and frequently comorbid with depression or anxiety. The most important single risk factors are female gender, younger age, and preceding GI infections. Recent studies suggest that trauma in childhood, especially sexual abuse, may be an important risk factor. The aspect of dysbiosis in IBS is important and is discussed elsewhere, but aspects of gut-to-brain communication are clearly altered. For example, elevated levels of plasma proinflammatory cytokines are found and there is an exaggerated pituitary-adrenal response to corticotropin-releasing hormone, together with augmented visceral pain responses. A recent study found that fasting serum levels of SCFAs did not differ between patients with IBS and controls. The postprandial levels of total SCFAs, acetic acid, propionic acid, and butyric acid were found, however, significantly lower in patients with IBS compared with healthy controls. An epigenetic model of IBS has been proposed, which is consistent with the potential epigenetic modulating effects of butyrate, the levels of which are altered substantially in the postprandial state.
Treatments of IBS that do not take into account this complex pathophysiology are likely to be of limited benefit ( Fig. 2 ).
Depression
IBS and depression are frequently comorbid and the latter is associated with the presence of biomarkers of inflammation, such as elevated IL-6, tumor necrosis factor (TNF)-α, and the acute-phase protein, C-reactive protein. Similar elevated biomarkers of inflammation have been seen in anxiety states and are known to occur as a result of stress. The site at which these proinflammatory molecules is produced in depression is not known and it has yet to be determined whether the elevation is core to the pathophysiology or merely epiphenomenal. There is evidence from rodent studies to indicate that stress alters the gut barrier function, allowing lipopolysaccharide (LPS) and other molecules to gain access to the bloodstream, stimulating Toll-like receptor 4 and other Toll-like receptors, resulting in the production of inflammatory cytokines. If this does occur in depression, which has yet to be definitively demonstrated, it would explain the proinflammatory phenotype observed.
Bercik and colleagues, using germ-free and specific pathogen-free mice, demonstrated that the early life stress of maternal separation alters the HPA axis and colonic cholinergic neural regulation in a microbiota-independent fashion. They showed, however, that the microbiota is required for the induction of anxiety-like behavior and behavioral despair. Colonization of adult germ-free maternally separated and control mice with the same microbiota produces distinct microbial profiles, which are associated with altered behavior in maternally separated mice but not in control mice. The results suggest that maternal separation–induced changes in host physiology lead to intestinal dysbiosis, which is a critical determinant of the abnormal behavior that characterizes this model of early-life stress. Prior studies in maternally separated rats demonstrated an altered behavioral phenotype when these animals reached maturity and also decreased diversity in the microbiota. Does this decreased diversity translate to patients with major depression?
In a recent study the fecal microbiota was sequenced ; 46 patients with depression and 30 healthy controls were recruited. High-throughput pyrosequencing showed that, according to the Shannon index, increased fecal bacterial α-diversity was found in those currently depressed but not in a group who had responded to treatment. Bacteroidetes, Proteobacteria, and Actinobacteria were increased, whereas Firmicutes was significantly reduced. Despite the profound interindividual variability, levels of several predominant genera were significantly different between the depressives and controls. Notably, the depressives had increased levels of Enterobacteriaceae and Alistipes but reduced levels of Faecalibacterium. The investigators conclude that further studies are necessary to elucidate the temporal and causal relationships between gut microbiota and depression and to evaluate the suitability of the microbiome as a biomarker. When rats are given a humanized microbiota from depressed patients as opposed to healthy controls, they develop a depressive phenotype from a behavioral and immune perspective.
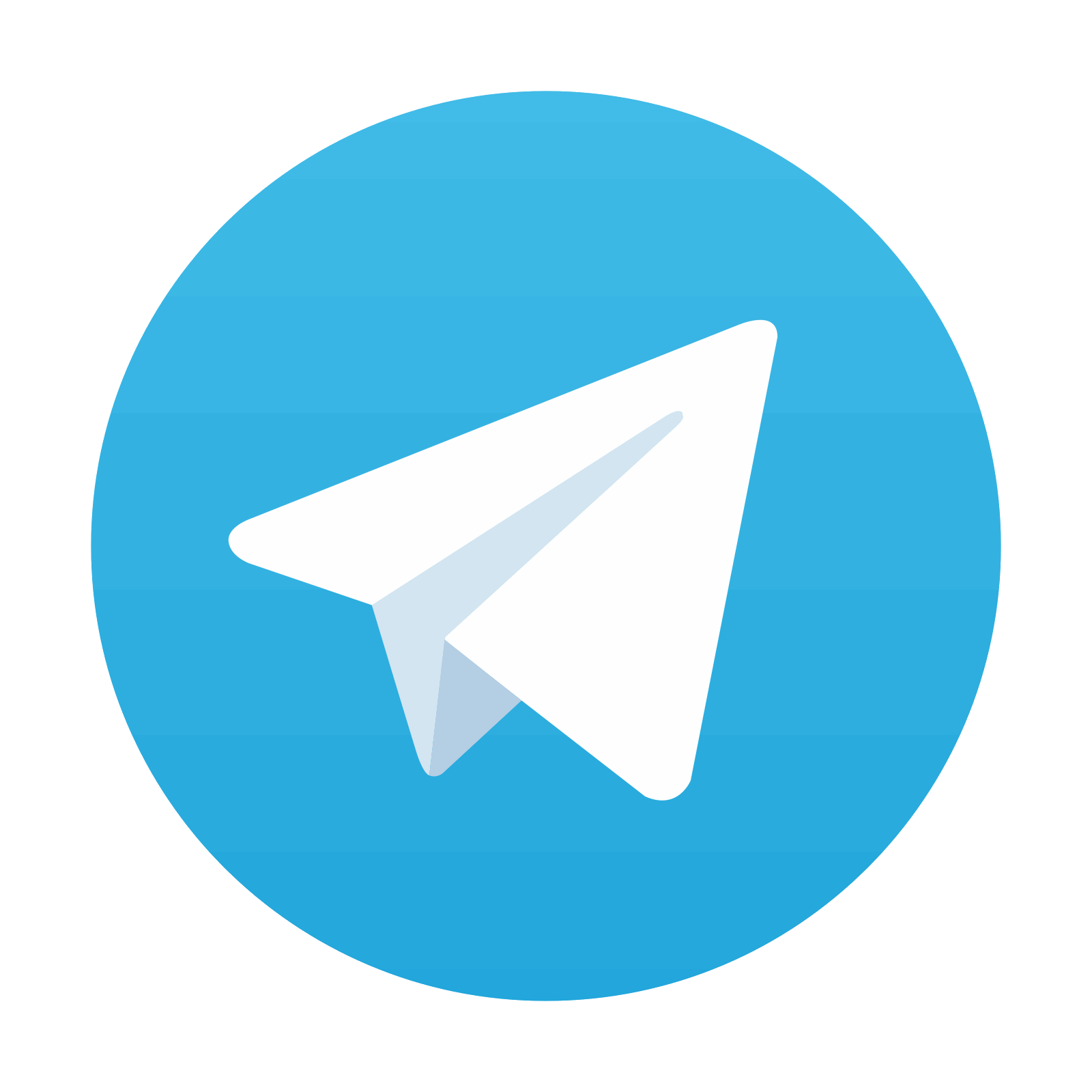
Stay updated, free articles. Join our Telegram channel

Full access? Get Clinical Tree
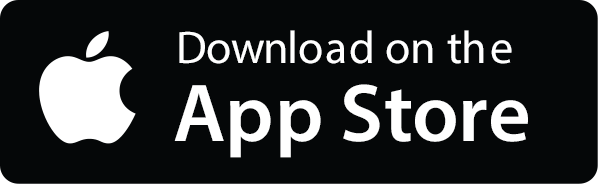
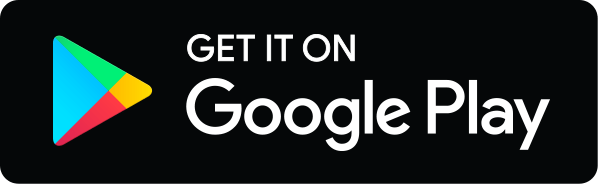