Fig. 2.1
The anatomy of the intestinal immune system. The basic organizational structure of the intestinal immune system. The follicular-associated epithelium (FAE) contains M (microfold) cells which transport antigen into the underlying subepithelial dome of Peyer’s patches. Antigen is processed and presented by DCs to naïve B and T cells. A similar process can occur in smaller lymphoid follicles, or DCs can exit the lamina propria and travel to associate mesenteric lymph nodes. Activated B and T cells are targeted back to the lamina propria where they exert their respective effector functions
The Innate Immune System and IBD
The immune system can be divided into two main branches: innate and adaptive. The innate immune system functions based on germ line-encoded recognition of microbial (and other) signals. The primary strength of the innate immune system is the rapid nature of its responses. Innate immune responses are fast because the receptor repertoire is predetermined, with specificity honed over the course of evolution. The components of the innate immune system range from pattern recognition receptors expressed by many nonimmune cell types to innate immune cell types including granulocytes, professional phagocytic cells (such as macrophages, dendritic cells, and neutrophils), and innate lymphocyte populations, all of which play essential antimicrobial and antineoplastic functions. The following section will review the innate immune system and its relevance to maintaining intestinal homeostasis or contributing to IBD pathogenesis.
Pattern Recognition Receptors
Pattern recognition receptors (PRRs) are germ line-encoded receptors that recognize an array of pathogen-associated molecular patterns (PAMPs). There are many families of PRRs. Two of the most well-characterized, membrane-bound Toll-like receptors (TLRs) and cytoplasmic NOD-like receptors (NLRs), will be briefly reviewed here because of their contribution to IBD pathogenesis. TLRs are a family of integral membrane proteins that are situated on the plasma membrane or on intracellular endosomes. TLRs recognize a broad array of PAMPs including bacterial lipoprotein, peptidoglycans, lipopolysaccharides, nucleic acids, and other microbial components. TLR activation results in nuclear factor kappa-light-chain enhancer of activated B-cell (NF-κB)-dependent expression of pro-inflammatory cytokines. TLRs are expressed throughout the intestine by epithelial cells and immune cells and play a central role in microbial surveillance. There are a number of lines of evidence that suggest that TLR signaling is altered in IBD. Polymorphisms that impair TLR signaling are associated with increased risk of IBD in humans [23–25]. Additionally, dysregulated TLR expression, in particular upregulation of TLR2 and TLR4, is observed in patients with IBD [26–28]. Finally, mice deficient in the TLR adaptor molecule MyD88 have a more severe disease phenotype when subjected to various models of intestinal inflammation [29].
NLRs recognize bacterial peptidoglycans that are present in the cytoplasm. NLRs signal through the CARD-containing serine-threonine kinase RIP2, which in turn activates TAK1 kinase and NEMO to cause MAPK activation and NF-κB-dependent expression of pro-inflammatory cytokines [30]. NLRs are of particular interest to the study of IBD pathogenesis because of the early observation that mutations in the NOD2 signaling pathway are significantly associated with CD, but in some cases protective from ulcerative colitis (UC) [31]. There are many theories as to why mutations in the NOD2 signaling pathway may predispose to CD. For example, NOD2 signaling inhibits cells from further TLR2 or TLR4 activation, a form of tolerance that may be lost in patients with NOD2 mutations [32, 33]. Additionally, it has been shown that mutations in an attenuator of NOD-driven cytokine responses, ATG16L1, are also associated with CD. It is not clear at this time why NOD2 mutations may be protective from UC, but this observation supports the notion that NOD2 signaling is integral to promoting immune homeostasis in the intestine.
Granulocytes: Neutrophils, Eosinophils, Mast Cells, and Basophils
Granulocytes are a group of innate immune cells that are characterized by the presence of granules in their cytoplasm. They include neutrophils, eosinophils, mast cells, and basophils. While granulocytes originate from a common myeloid precursor, they have distinct properties in their mature form. Neutrophils (PMN) are the most abundant form of granulocyte and make up the majority of peripheral circulating immune cells in humans. Unlike the other granulocytes, PMNs are primarily phagocytes (meaning they actively seek out and engulf particles, bacteria, or dead or dying cells). As a result, they play a central role in early antibacterial immunity. Eosinophils, mast cells, and basophils fall into the category of “type 2” granulocytes in that they are classically thought of as effector cells that mediate antiparasite immune responses (or allergic inflammation) downstream of T-helper (TH) 2 cell differentiation. Both eosinophils and basophils are predominantly found circulating in the blood, while mature mast cells are predominantly a tissue-resident population. Eosinophils and basophils hone to sites of tissue inflammation where they, along with mast cells, exert effector functions including release of granules containing cytokines, chemokines, enzymes, and growth factors. In addition, it has more recently been appreciated that some granulocytes, most notably basophils, can circulate from sites of tissue inflammation to draining lymph nodes where they can influence the development of adaptive immune responses [34].
There is substantial evidence to support the notion that PMNs play a central role in the effector stage of IBD pathogenesis. Locally, PMNs infiltrate inflamed colonic tissue in UC as evidenced on pathology, and by measurements of fecal calprotectin (a stable by-product of neutrophilic inflammation that is tested clinically parallels intestinal inflammation and predicts relapse) [35]. It has also been shown that interfering with PMN recruitment to sites of tissue inflammation, through the use of CXCR2 antagonists, chemokine analogs, anti-CXCR2 monoclonal antibodies, or CXCR2 knockout mice, is associated with reduced colonic inflammation in animal models [36]. Additional evidence supporting a role for neutrophil dysfunction in IBD pathogenesis is the defective acute inflammatory response observed in CD patients. Impaired neutrophilic infiltration, IL-8 production, and vascular flow have all been shown in CD [37]. Furthermore, treatment of patients with GM-CSF, a growth factor that supports neutrophil development and function, has been studied as a potential therapy in CD patients [38, 39].
Macrophages
Macrophages are a phagocytic cell type that play an important role in clearance of bacteria and infected or dead cell remnants. Macrophages also circulate to associated lymphoid tissues where they help to initiate adaptive immune responses. Macrophages develop from embryonic precursors or bone marrow-derived monocytes that can be recruited to sites of tissue inflammation; however, in the steady state, mature macrophages are primarily resident in tissues such as those of the GI tract and associated lymphoid structures. So termed “tissue-resident macrophages” are highly plastic and take on unique functional characteristics specific to their resident tissue. For example, intestinal resident macrophages promote tolerance to foreign substances, food-derived proteins, and commensal bacteria [40, 41]. This unique function may be in part due to their relative hypo-responsiveness to TLR ligands and diminished ability to prime adaptive immune responses [42].
During times of infection and inflammation, however, macrophages are activated through PRRs and become effective modulators of the innate and adaptive immune systems. Activated macrophages express membrane-bound PRRs specific for opsonized particles and pathogens, complement, and bacterial proteins. Recognition of pathogens via these receptors leads to phagocytosis and intracellular degradation as well as the secretion cytokines. Activated macrophages produce large amounts of TNF-α and IL-12, two cytokines responsible for the recruitment and activation of pathogenic effector T cells. The cytokine TGF is also produced by activated macrophages and is a potent chemoattractant leading to the recruitment of monocytes and neutrophils from the blood to the site of inflammation [43]. While initially pro-inflammatory, these newly recruited monocytes can develop into an anergic state (potentially as a result TGF and IL-10 signaling) that results in profound downregulation of pro-inflammatory cytokine release resumption of mucosal homeostasis [44, 45].
The role of macrophages in IBD pathogenesis is on ongoing field of study. It is known that in IBD there is a large influx of macrophages into the intestinal mucosa, and these macrophages display a distinct phenotype and distribution as compared to tissue-resident macrophages in the steady state. For example, in UC pro-inflammatory macrophages are found throughout the epithelium and underlying lamina propria, while macrophage infiltrates observed in CD are extensive and include the underlying mesenteric muscular layer and fat tissue [42]. These infiltrating macrophages have increased expression of the CD33, the IgG receptor CD64, and the chemokine receptor CX3CR1, but express low levels of HLA-DR [46]. The infiltrating macrophages observed in IBD are likely pathogenic as they exhibit NF-κB-dependent expression of pro-inflammatory cytokines including TNF-α, IL-6, IL-8, IL-12, IL-23, IL-1β, and IFNγ [47–49]. Consistently, the efficacy of anti-TNF-α therapy in IBD is well established [50, 51]. Furthermore, promising anti-IL-12 studies such as the use of the anti-IL-12/23p40 antibody ustekinumab have shown efficacy in Crohn disease, supporting a role for these cytokines in IBD pathogenesis [52, 53]. Finally, the ability of macrophages to enter an anergic state may be impaired in IBD [54]. Additional studies may further elucidate the role of macrophages in IBD pathogenesis.
Dendritic Cells
The dendritic cell (DC) is considered to be one of the most effective of the professional antigen-presenting cells (APCs), and intestinal DCs play an important role in determining and directing the inflammatory response to an antigen. DCs express a wide array of PRRs that detect environmental signals and modulate their expression of cytokines that promote specific lymphocyte responses [55]. DCs can encounter antigen at sites of antigen transport across the epithelium via M cells or via direct luminal sampling [56]. Upon detecting antigen, DCs activate and migrate to T-cell areas in lymphoid structures where they display major histocompatibility complex (MHC)-peptide complexes and co-stimulatory or inhibitory signals to naïve T cells. DCs and other innate cells further dictate T-effector cell polarization using membrane-bound or secreted cytokines [57]. Under homeostatic conditions, intestinal DCs express low levels of co-stimulatory molecules and cytokines and preferentially promote the differentiation of Tregs. However, under conditions of pathogen invasion or inflammation, DCs promote T-cell polarization into one of the many known inflammatory T-helper cell subtypes (TH1, TH2, TH17). Through this critical role, DCs play an important role in modulating adaptive immune responses to maintain intestinal immune homeostasis (Fig. 2.2).


Fig. 2.2
The major molecular receptor-ligand pairs involved in antigen-specific T-cell stimulation. The initial interaction between the APC and the naïve T cell occurs between antigen bound to major histocompatibility complex (MHC) class II and the T-cell receptor (TCR) (signal 1). A co-stimulatory signal (signal 2) is required for T-cell activation. T cells become anergic when presented with signal 1 in the absence of signal 2. Many inhibitory co-stimulatory molecules have also been identified such as the cytotoxic T-lymphocyte-associated antigen 4 (CTLA4). Finally, cytokines (which can act in a paracrine or autocrine manner) act as third signal to help determine the T-helper cell subtype (TH1, TH2, TH17, Treg) and provide for optimal activation
Recent studies have attempted to correlate murine DC subsets with those found in the intestines of humans. Through these efforts it has been found that human CD103+Sirpα– DCs express markers of cross-presentation (the ability process and present antigen via MHC class I molecules to CD8 T cells) and are most similar to mouse intestinal CD103+CD11b– DCs that are protective in models of experimental colitis [58, 59]. Furthermore, human DCs that expressed both CD103 and Sirpα are most similar to murine CD103+CD11b+ DCs as they seem to have a prominent role in inducing Treg responses [59]. Human CD103+ DC subsets induced TH17 cells, while CD103– Sirpα+ DCs induce TH1 cells [59]. Finally, gut-derived DCs can cause homing of lymphocytes primed in the MLN back to the intestine by producing retinoic acid which upregulates α4β7 and CCR9 on lymphocytes [60].
While the majority of our knowledge of DC function in health and disease stems from studies in mice, there have been some intriguing observations made in humans that may help us understand how this key cell type could contribute to the pathogenesis of IBD. A paradigm has emerged in experimental models where DCs promote regulatory or inflammatory responses depending on the stage of inflammation. Early in the inflammatory process, intestinal DCs seem to protect the intestinal mucosa as their depletion leads to more profound inflammatory states [58, 61]. In settings of more long-standing immune dysregulation, however, such as the constitutive absence of TGFβ signaling, DCs fail to assume a regulatory phenotype and play an active role in promoting pathologic T-cell responses [62, 63]. In light of these observations, one can envision a scenario where DCs are primarily pathogenic in human IBD. Consistent with this hypothesis, intestinal DCs from patients with CD or UC have higher baseline expression of TLR2 and TLR4 and higher expression of the activation marker CD40 [64]. Furthermore, colonic DCs from CD patients express higher levels of IL-12 and IL-6 in the steady state, suggesting that DCs from patients with IBD are constitutively active [64]. Together, these observations provide some insights into how intestinal DCs may contribute to IBD pathogenesis.
Innate Lymphoid Cells and Other Innate Lymphocytes
Innate lymphoid cells (ILCs), which lack a recombined antigen receptor, have been found to play important roles in mouse models of infection, inflammation, and tissue repair and be dysregulated in multiple human disease states [65]. ILCs are organized into three groups, ILC1, 2, and 3, depending on the transcription factor required for their development and their functional profile. ILC1s (which include natural killer cells) are characterized by T-bet expression, are responsive to IL-12, and produce IFNγ. ILC2s are characterized by GATA3 expression; are responsive to IL-25, IL-33, and TSLP; and produce IL-4, IL-5, IL-9, and IL-13 and amphiregulin. ILC3s (which include lymphoid tissue inducer cells) are characterized by RORγt expression, are responsive to IL-1β and IL-23, and produce IL-17 and/or IL-22 [65].
NK cells, a prototypical member of the ILC1 family, are a type of cytotoxic lymphocyte that kill target cells via cytoplasmic granule toxins or through engagement of death receptors that cause caspase-dependent apoptosis [66]. NK cells are also an early source of IFNγ and can promote differentiation of TH1 cells [67]. CD16+ NK cells have been found to be increased in the colonic lamina propria of IBD patients, and it was observed that these NK cells were reduced after azathioprine therapy [68]. As a result, NK cells may promote intestinal inflammation in some forms of IBD.
ILC3s may also be of particular relevance to human IBD, but data indicate that ILC3s can play protective or pro-inflammatory roles depending on the experimental or clinical context. On one hand, ILC3s have been shown to mediate intestinal repair in some animal models of acute injury [69–72], and their numbers are reduced (and ILC1 numbers increased) in intestinal tissue samples of some patients with IBD [73–76]. Furthermore, ILC3s can directly induce cell death of activated commensal bacteria-specific T cells, and MHCII on colonic ILC3s is reduced in pediatric IBD patients [77]. On the other hand, ILC3s seem to have a pro-inflammatory phenotype in other animal models [62, 78–80], and there are reports of both increased frequency and IL-22 production by ILC3s in IBD patients [81–83]. Part of the confusion regarding the exact role of ILC3s in IBD may stem from the heterogeneity of this group, as well as the potential for plasticity among ILCs [84, 85]. Nevertheless, further investigation of ILC3s in the context of IBD is warranted.
γδ T cells are a small subset of T lymphocytes which express a T-cell receptor (TCR) composed of the γ- and δ glycoproteins. Unlike conventional αβ T cells, γδ T cells do not require the thymus for development [86], do not recognize antigen in association with MHC class I or II [87], and do not participate in adaptive immune responses [88]. Like ILC3s, γδ T cells play protective or pro-inflammatory in IBD roles depending on the experimental or clinical context. For example, γδ T cells are recruited to inflamed tissue in CD and UC where they produce IFNγ [89, 90]. However, in two models of intestinal inflammation, γδ T cells appear to be protective [91–94], while in other models γδ T cells can take on a pathologic role [95, 96].
Invariant natural killer T cells (iNKT cells) share cell-surface proteins with conventional T cells and NK cells. iNKT cells mature in the thymus, recognize lipid antigen presented via the “MHC-like” complex CD1d, and secrete large amounts of pro-inflammatory cytokines to kill infected and neoplastic cells. Quantitative analysis has shown that iNKT cells are reduced in inflamed colonic tissue from patients with UC [97]. However, iNKT cells isolated from patients with UC produce large amounts of IL-13 and have cytotoxic activity against colonic epithelial cells [98]. As such, the exact role of iNKT cells in IBD pathogenesis is unclear.
Together, innate lymphocytes are a heterogeneous population of cells with diverse functions in health and disease. While they are almost certainly involved in IBD pathogenesis, their role seems to change with different experimental and clinical contexts. As such, more experimentation is necessary to fully understand the role of these cells in IBD pathogenesis.
The Adaptive Immune System and IBD
The adaptive immune system is made up of two subsets of lymphocytes, B and T cells. Both B and T lymphocytes develop in the bone marrow, but T lymphocytes undergo a necessary maturation process in the thymus. The primary strength of the adaptive immune system stems from the specificity of responses. In addition to mediating immunity to pathogens, the adaptive immune system provides a necessary regulatory role in controlling immune responses to self and foreign antigens. The following section will review the adaptive immune system, its relevance to maintaining intestinal homeostasis, and how it may contribute to IBD pathogenesis.
B Cells and IBD
As mentioned previously, B cells primarily develop in the bone marrow; however, B cells can also develop via extramedullary hematopoiesis. Early in embryonic development, pluripotent hematopoietic stem cells migrate to the liver where mature B cells develop and migrate to the intestine. There are several checkpoints in B-cell development directed at ensuring normal recombination of the heavy and light immunoglobulin loci and blocking cells with autoreactive B-cell receptors (BCR) from making it to maturity. The GALT and commensal microbiota of the intestine play an important role in B-cell development and tolerance. Immature transitional B cells transit through the GALT where autoreactive cells are removed from the developing repertoire [99]. When an autoreactive BCR is detected, it causes clonal deletion, anergy, or editing of the BCR depending on the strength of the autoreactivity. Clonal deletion is the fate of approximately 85% of the newly formed immature B cells. Only cells that reach maturity are available to respond to antigen presented by follicular DCs and other APCs in the germinal center. Antigen recognition can result in activation and proliferation, but not class switching, hypermutation, or memory formation, all of which require T-cell help [100].
Class switching is a biological mechanism that changes the class of immunoglobulin produced by the B cell. Class switching occurs in the germinal center through interactions with TH cells. Naïve mature B cells produce both IgM and IgD, which are the first two heavy chain segments in the immunoglobulin locus. The class switched molecule contains the same variable domains as generated during recombination, but possesses distinct heavy chain constant domains. After activation, B cells present antigen to cognate T cells which in turn express cell-surface co-activation molecules and cytokines. This coupling enables class switching, hypermutation, and the formation of memory cells or high-affinity antibody-producing cells which secrete IgG, IgA, or IgE molecules [101].
One of the primary roles of B cells in mucosal immunity is the production of secretory IgA, and to a lesser extent secretory IgM. IgA is a dimeric molecule in its secreted form and it plays an important role in mucosal defense. The joining or “J” chain of polymeric IgA spontaneously interacts with the polymeric Ig receptor expressed on the basolateral surface of epithelial cells facilitating exportation of the IgA to the gastrointestinal lumen [102]. In this manner, a vast quantity of IgA is secreted across the epithelium into the intestinal lumen (approximately 3 grams per day, and three-quarters of the total secreted daily immunoglobulin) where it plays an important role in regulating commensal and pathogenic bacteria [103]. However, the relationship between IgA and luminal bacterial is not unidirectional. We know from studies of germfree animals that IgA production is acutely dependent on the presence of luminal microbes (Benveniste et al. [104, 105]). The induction of IgA responses can occur in either a T-cell-dependent or T-cell-independent manner (via the cytokines BAFF and APRIL) facilitating the rapid recognition and response to commensal-derived signals [21, 22].
Despite the known roles for IgA in maintaining mucosal homeostasis, patients who lack the molecule (selective IgA deficiency) have relatively few clinical sequelae. Of those that are known to exist, the most common are recurrent sinopulmonary infections, allergies, autoimmune diseases, and progression to more significant forms of humoral immunodeficiency [106]. Associations between selective IgA deficiency and IBD have been reported (the prevalence of IBD in healthy or IgA-deficient individuals is 3.9% vs. 0.81%, respectively), suggesting that IgA provides a degree of protection from IBD [107, 108]. Under normal circumstances, the B-cell responses that result in the production of IgA are limited to the MLNs and do not occur in systemic secondary lymphoid structures. One hypothesis as to how inappropriate B-cell responses may contribute to IBD pathogenesis centers on the breakdown of this normal compartmentalization [109, 110]. In support of this hypothesis, systemic humoral responses to bacterial components, such as membrane and flagellar proteins, have been found in children with IBD [111].
T Cells and IBD
T cells play many roles in the immune system including cell-mediated immunity against tumors and virus-infected cells, providing help to stimulate B-cell activation and antibody class switching, and differentiating into key effector cell types that mediate immunity to a vast array of pathogens. Additionally, in the case of regulatory T cells (Tregs), T cells play important roles in attenuating inappropriate inflammatory responses and promoting tolerance. It is perhaps not surprising then that problems in any of these diverse functions can predispose to multiple human disease states including neoplasms, infections, allergies, and autoimmunity. T cells originate in the bone marrow but mature in the thymus. With a similar goal to the checkpoints discussed earlier for B cells, the thymus is the location where T cells undergo selection to ensure that their T-cell receptor (TCR) is both capable of interacting with MHC and not autoreactive. T lymphocytes begin to populate the lamina propria by 12 weeks of gestation, and Peyer’s patches consisting of B-cell follicles and follicular CD4+ and CD8+ T cells are detected by 16–18 weeks of gestation [112]. Dendritic cells are detected at 19 weeks, representing the last of the required cellular components of an adaptive immune response [113]. The number of Peyer’s patches steadily increases from 80 to 120 at birth to 250 by adolescence [114].
There are two major classes of T cells, CD4+ and CD8+. CD8+ T cells are responsible for cell-mediated immunity and provide an essential role in surveillance of tumor and virus-infected cells and are otherwise outside the scope of this chapter [115]. Naïve CD4+ T-helper (TH) cells circulate the lymphatic tissues in search of their cognate MHC-peptide complex. Constitutive expression of the selectin CD62L (L-selectin) and the chemokine receptor 7 (CCR7) ensures that naïve T cells bind to glycosylated CD34 and the chemokine ligand 21 expressed on endothelial cells of high endothelial venules [116]. The interaction of CD62L and CD34 (among other glycosylated endothelial molecules) promotes a rolling action of the T cell across the endothelial surface. The T-cell surface adhesion molecule LFA-1 then binds to ICAM-1 and ICAM-2 promoting firm adhesion and crossing of the endothelial lining into the lymphoid tissue. Within the underlying lymphatics await DCs loaded with antigen in variable states of activation. Naïve T cells search the DC selection for a recognizable MHC-peptide complex. If none is found, the cells exit the lymph node, return to the circulation, and reenter other nodes to repeat this process.
When a naïve TH cell finds a suitable cognate MHC-peptide complex, it is activated via recognition of antigen via their T-cell receptor (TCR) (signal 1), as well as interactions with co-stimulatory or inhibitory molecules on the APC (signal 2) [57]. Recognition of cognate MHC-peptide complex by the TCR in the absence of co-stimulation results in anergy, defined as a lack of response upon reexposure to the same antigen in the future. A third signal, coming in the form of paracrine or autocrine cytokine signals, can enhance T-cell activation. A key consequence resulting from activation of CD4+ T cells is cytokine secretion, and the particular pattern of cytokines secreted orchestrates the type of the ensuing immune response. There are at least four commonly recognized subsets of TH cell, each with a distinct cytokine expression profile. TH1 cells are characterized by T-bet expression, are induced by IFNγ and IL-12, and produce IL-2, IFNγ, and TNF-α to help fight intracellular infections. TH2 cells are characterized by GATA3 expression, are induced by IL-4 and IL-2, and produce IL-4, IL-5, IL-9, and IL-13 to help fight parasitic infections. TH17 cells are characterized by RORγt expression; are induced by TGFβ, IL-6, and IL-21; and produce IL-17, IL-21, and IL-22 to help fight bacterial infections [65, 117]. A forth TH subset, regulatory T cells (Tregs), will be discussed separately. Full activation of T cells takes 4–5 days and is accompanied by clonal expansion and homing of effector cells to sites of tissue inflammation (Fig. 2.3).


Fig. 2.3
Activation pathways, transcription factors, and effector cytokine profiles of the major TH cell subsets. The ultimate functional phenotype of mature effector T cells depends upon the type and amount of antigen, and the cytokine milieu present at the time of activation. Activate TH cell subsets have characteristic transcription factor and cytokine expression patterns
Within the lamina propria of the intestine, CD4+ and CD8+ T cells bearing the conventional TCR are roughly equally represented. Once activated, these effector T cells leave the lymph node and return to the circulation via the upregulation of the 47 integrin and CCR9 chemokine receptor [118]. Activated T cells produce pro-inflammatory cytokines, such as TNF, that stimulate endothelial cells to upregulate adhesion molecules such as E-selectin (which recruits monocytes and neutrophils) and VCAM-1 and ICAM-1 (both of which recruit activated T cells). TNF and IFN likewise act to alter the vascular permeability, endothelial cell shape, and blood flow, resulting in enhanced infiltration of inflammatory cells into the tissue. These inflammatory cascades set in motion by activated T cells, and the significant structural alterations they cause in the inflamed tissue, eventually trigger the signs and symptoms characteristic of active IBD.
There are multiple lines of evidence strongly supporting the notion that activated CD4+ T cells are a central feature of human IBD. First, T-cell-driven animal models of colitis mimic human IBD in both histologic features and susceptibility to similar treatment regimens [84, 106, 109, 119]. In human IBD, IL-17-secreting TH17 cells have now been an established observation in CD, while UC represents an atypical TH2 response [98, 120, 121]. Furthermore, the IL-23 receptor variant associated with CD results in impaired IL-17 production and is a protective genetic variant [122]. Additionally, T-cell clones have been identified in patients with CD, and similar populations that specifically recognize endogenous gut bacteria promote colitis in animal models [123, 124]. Finally, there are both established and emerging therapies for IBD that are directed toward the destruction or deterrence of activated effector T cells, or blockade of Th1-driving cytokines [125].
Regulatory T Cells
Regulatory T cells (Tregs) are a subset of T lymphocytes that play an important role in the prevention and control of many autoimmune and allergic diseases [126]. Tregs are characterized by the expression of Foxp3, are induced by IL-2 and TGFβ, and produce TGFβ and IL-10. There are two generally accepted mechanisms of Treg development. So-called “naturally occurring” Tregs acquire Foxp3 expression while undergoing selection in the thymus. Alternatively, “inducible” Tregs develop in the periphery from mature CD4+ TH cells [126]. The importance of Tregs to normal human physiology is perhaps best typified by descriptions of patients with immunodysregulation, polyendocrinopathy, enteropathy, and X-linked (IPEX) syndrome. IPEX is caused by mutations in the FOXP3 gene and results in the clinical phenotype of severe autoimmune phenomena including autoimmune enteropathy, dermatitis, thyroiditis, and type 1 diabetes which can result in death within the first 2 years of life if bone marrow transplant is not successful [127].
A discussion of Tregs in the context of IBD is perhaps most effective when one considers their intimate relationship with TH17 cells. Despite their very different effector functions, Treg and Th17 cells share the requirement for TGFβ to develop from naïve TH cells. Furthermore, naïve TH cells will initially upregulate both Foxp3 and RORγt when stimulated by TGFβ or TGFβ and IL-6. Foxp3 can inhibit the transcriptional activity of RORγt, and as such in Treg-inducing conditions (high TGFβ), Foxp3 expression and ultimately Treg development will be favored. Alternatively, in more pro-inflammatory conditions (with low TGFβ and high IL-6, IL-21, or IL-1β), FoxP3 activity is inhibited and TH17 differentiation is favored [128]. In addition to developmental competition, Tregs can directly inhibit the function of TH17 cells via the secretion of IL-10, providing an additional layer of cross-regulation between these two T-effector-cell populations [129].
Though the importance of Tregs in suppressing mucosal inflammation in murine models has been well established, and Treg populations have been characterized in patients with IBD, specific Treg defects that may predispose to IBD in humans have not been identified [130, 131]. For example, CD4+CD25+ Tregs are present in the lamina propria of IBD patients, and they have similar in vitro T-cell suppressive activity as controls [132]. Contrary to what one might expect, Tregs are more common in inflamed bowel of patients with IBD [133]. Thus, the inflammation observed in IBD is unlikely to be due to a quantitative or functional defect in Tregs. As such, the specific contribution of Tregs to IBD pathogenesis is an ongoing area of investigation.
Commensal Bacteria and IBD
The role of commensal bacteria in IBD pathogenesis will be reviewed in detail in another chapter of this book. However, commensal bacteria play an important role in the normal development of the mucosal immune system and are required for the development of colitis in most murine models of IBD. Additionally, the presence of antibodies to bacterial antigens, and the beneficial effects of antibiotics and probiotics in most patients with IBD suggest an important role of bacteria in the instigation or perpetuation of human IBD. Thus, understanding the mechanisms by which commensal bacteria interact with the innate and adaptive immune system is highly relevant to understanding IBD pathogenesis.
As discussed previously, IECs express innate pattern recognition receptors including Toll-like receptors (TLRs), NOD-like receptors (NLRs), and GPCRs that recognize microbial components and modulate immune responses via the regulation of pro-inflammatory cytokines, chemokines, and antimicrobial peptides [11]. Experimental evidence suggests that interaction between commensal gut flora and PRRs protects against colitis and enhances the mucosal barrier [134, 135]. Furthermore, commensal bacteria play an important role in promoting B-cell development and tolerance, an important developmental checkpoint that fails in patients with autoimmunity [99].
Despite multiple mechanisms by which commensal bacteria help maintain mucosal homeostasis, there are at least four mechanisms by which commensal bacteria could be the targets of inappropriate immune responses. First, infected epithelial cells may undergo apoptosis (either spontaneously or as a result of immune-mediated cytotoxicity), and apoptotic fragments containing commensal bacteria may be cross-presented APCs via MHC class I to CD8+ T cells [136]. Second, DCs and macrophages may acquire bacteria directly, presenting bacterial antigen on MHC class II to CD4+ T cells. Third, lymphocytes may be activated by bacterial antigen in the lamina propria directly via PRRs or indirectly via APCs. Fourth, APC-containing bacteria or bacterial components may migrate to draining mesenteric lymph nodes where they could interact with lymphocytes [137]. Thus, multiple pathways exist with the potential of presenting commensal bacterial products to lymphocytes to result in inappropriate and potentially pathogenic inflammatory responses (Fig. 2.4).


Fig. 2.4
Potential pathways through which bacterial antigens may activate mucosal T cells. (1) Apoptosis of infected epithelial cells containing bacterial antigens by macrophages (Mφ) and other scavenger cell types. (2) Luminal sampling and acquisition of bacteria by mucosal dendritic cells (DCs). (3) Direct or indirect activation of T cells by bacterial antigens in the lamina propria via pattern recognition receptors (PRRs) or APCs. (4) Migration of APC-containing bacteria or bacterial components to draining mesenteric lymph nodes (MLN)
The Pediatric Immune System
Maturation of the mucosal immune system is a continuum with no definitive markers defining a “mature” and “immature” status. The only evidence available from which to draw conclusions unique to the pediatric mucosal immune system is from studies of human neonates or animal studied at the time of weaning. The most noticeable difference between developing (neonatal) and established immune responses would appear to be in adaptive immunity. The rationale for this assumption is that adaptive immune responses are antigen specific and thus require postnatal exposure to dietary and microbial antigen to develop immunologic memory. In contrast, innate immune responses are for the most part germ line-encoded through recognition of microbial ligands by pathogen recognition receptors. Indeed, fetal intestinal epithelial cell lines exhibit responsiveness to inflammatory stimuli and bacterial products [138]. This section will briefly address two commonly accepted differences in the immune systems of children and adults.
Neonates are capable of some humoral and cellular immune responses at the time of birth. At birth, the gastrointestinal tract encounters microbes that are resident in the birth canal and the surrounding environment. Within hours after birth, the gastrointestinal tract begins colonization with commensal bacteria. Specific secretory IgA responses to organisms such as Escherichia coli are produced within the first week of life, likely via T-cell-independent mechanisms of B-cell class switching discussed earlier [139, 140]. Infants and young children are capable of generating the full spectrum of functional TH cells and T-cell-dependent B-cell responses, though it is important to note that T-cell-independent B-cell immune responses do not reach full maturity until about 15–14 months of life [141]. This humoral deficiency is compensated to a large degree by passive transfer of antibodies and immune cells across the placenta and via breastfeeding [142]. Nonetheless, impaired B-cell responses to T-cell-independent antigens may leave young children susceptible to infection by polysaccharide-encapsulated bacteria [143].
The second major difference is the tendency of the young mucosal immune system to generate allergic immune responses to oral and environmental antigens. This is generally believed to be one explanation for the high prevalence of atopic conditions in children [144]. The evidence for this unique feature of the immature immune system exists in epidemiologic and translational studies of children with food allergy [145, 146] and animal models demonstrating impaired oral tolerance in young mice [147]. How many of the aforementioned features of the immature immune system relate to human IBD remains to be explored.
Summary
As discussed in other chapters of this book, it is obvious that all recent advances in the field of novel therapeutic approaches, notably biological therapies, are directly derived from knowledge harnessed by studies of mucosal immunity in the normal and inflamed intestine [125]. In spite of these striking advances, some aspects of gut immunity remain unclear. Perhaps the most critical one is how the mucosal immune system interacts with the endogenous commensal flora under physiological and pathological conditions, a fundamental issue considering the widely accepted notion that IBD is an abnormal immune response to the autologous enteric bacteria in genetically susceptible individuals. The characterization of the relationship between the gut microbiota and the immune system is ongoing and greatly improved through the use of shotgun metagenomic sequencing. As we understand more about the residents of the gut microbiota and the function, the interaction with the immune system provides new insights on the initiation of the inflammatory response in IBD.
Another critical aspect of gut immunity is the nature of the mucosal immune response mediating inflammation during the course of a chronic disease, as typically found in IBD. It is plausible, if not probable, that long-lasting intestinal inflammatory processes like CD and UC undergo substantial changes of the underlying biological response regardless of the initial triggering events. This point is fundamentally important for pediatric IBD, when the disease is at its earliest possible stages of detection, and there is perhaps an opportunity to change the natural history of the disease, a far more difficult goal to attain in adult IBD patients with a long clinical history. Another unique population is patients with very early-onset IBD, in whom genomics is a larger disease-driving force than older populations, including genes of immunodeficiency.
It is not a coincidence that the disease develops as the immune system and gut microbiome are in the process of maturation, largely in a codependent manner. The relationship between host genetics and developing microbiome can be seen through germfree (GF) murine models. GF mice have underdeveloped gut-associated lymphoid tissue. In an environment with specific-defined flora, the gut microbiota elicits host-specific T-cell response and differentiation [89]. Concurrently, the aberrant T-cell development of GF mice shapes the gut microbiome, as seen by the different phylogenetic compositions of the microbiota in Rag1-deficient mice [125]. Zebra fish models show similar findings, in which Rag1-deficient zebra fish had overgrowth of Vibrio species [98]. Studying early events of IBD pathogenesis and following them up during disease evolution into chronicity are obviously difficult in humans and more so in children, but this can be accomplished in animal models, which are starting to generate concrete evidence of substantial changes in the gut immune response from the early to the chronic stages of inflammation. Switches from a TH1 to a TH2 pattern occur in at least two models of experimental IBD. In the colitis of IL-10-deficient mice and the ileitis of SAMP1/YitFc mice, both of which are Th1 mediated initially, there is a marked increase in disease-mediating Th2 cytokines in late disease, e.g., IL-4 and IL-13 in the colitis model and IL-5 and IL-13 in the ileitis model [148, 149]. The lesson here is that, even though the clinical manifestations of IBD remain constant, the underlying pathogenic immunopathology changes rather dramatically over time. Therefore, therapies that are effective in early disease may no longer be effective in chronic disease, as again exemplified by the above animal models where blockade of Th1 cytokines is therapeutically effective in early but not late inflammation, a period when blockade of Th2 cytokines ameliorates disease.
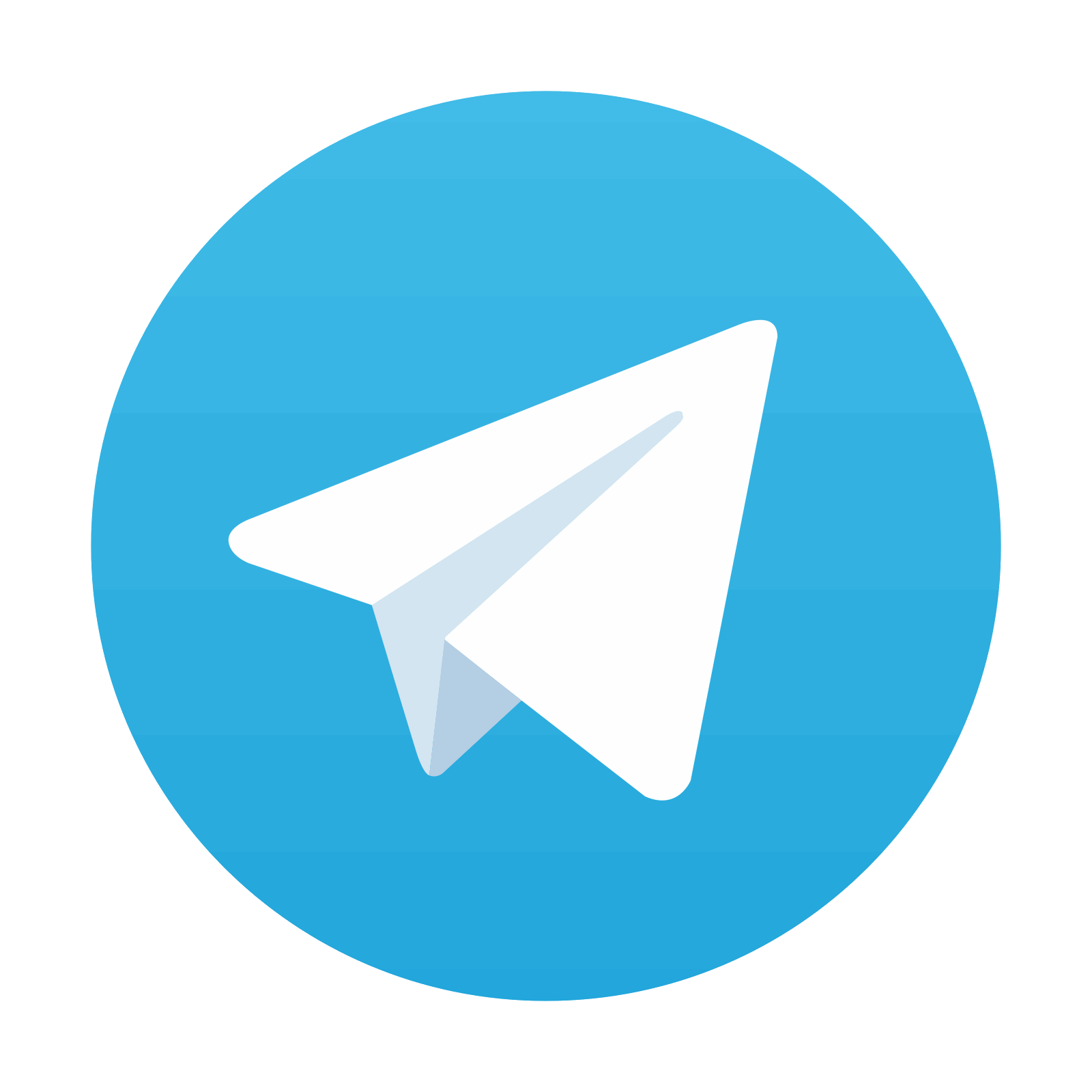
Stay updated, free articles. Join our Telegram channel

Full access? Get Clinical Tree
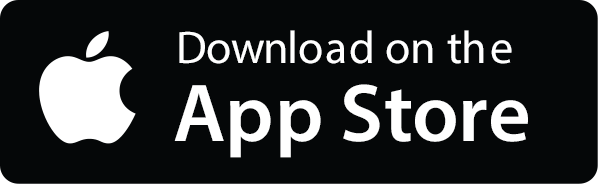
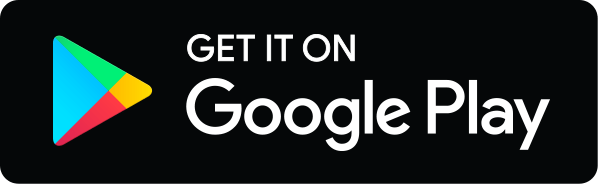