Clinical/biochemical indicator
1 point
2 points
3 points
Serum bilirubin (mg/dL)
<2
2–3
>3
Serum albumin (g/dL)
>3.5
2.8–3.5
<2.8
Prothrombin time (s > control)
<4
4–6
>6
Encephalopathy (grade)
None
1 or 2
3 or 4
Ascites
Absent
Slight
Moderate
A static model to predict the relative change of drug exposure in cirrhotic patients has been developed. Although further evaluation of the model needs to be made, the Child-Pugh based tool may help clinicians in adjusting drug dose regimens in this problematic patient population. The model is based on ratios of the altered drug area under the concentration-time curve (AUC*) in a typical cirrhotic patient to the AUC measured in a typical healthy subject, predicted as a function of the Child-Pugh classes A, B, or C. A web-based version can be found at www.ddi-predictor.org [10].
Drug-Drug Interactions
Patients with cirrhosis have many risk factors that may predispose them to drug-drug interactions and subsequent adverse drug reactions [11]. The magnitude of drug-drug interactions is expected to vary with the severity of liver impairment, however, there are very few studies documenting the impact of drug interactions on drug exposure in patients with cirrhosis. A limited amount of data suggests minimally decreased irreversible CYP3A enzyme inhibition and significantly increased free fraction (from decreased plasma protein binding) in patients with liver disease [12]. Cirrhosis also results in blood shunting around hepatocytes which may reduce drug delivery to metabolizing enzymes. In addition to alterations in drug metabolism through the CYP system, these patients are often times prescribed multiple medications to manage complications of their cirrhosis which would only increase their odds for a drug-drug interaction. A sound knowledge of the principles of dose adjustment in cirrhosis and an awareness of the most important potential drug-drug interactions of the therapeutic agents used to treat the complications of liver disease in this population is essential.
Recommendations for Select Medications in Surgical Patients
Analgesics
Acetaminophen is commonly recommended as a first-line analgesic due to its overall tolerability. However, the use of acetaminophen in patients with liver dysfunction is often avoided due to the established relationship between acetaminophen overdose and hepatotoxicity. Acetaminophen is primarily metabolized in the liver by three separate pathways: conjugation with glucuronide, conjugation with sulfate, and oxidation via the cytochrome P450 enzyme pathway, primarily CYP2E1, to form a reactive and hepatotoxic metabolite N-acetyl-p-benzoquinone imine (NAPQI). With therapeutic doses, NAPQI undergoes rapid conjugation with glutathione. Acetaminophen metabolites are mainly excreted in the urine.
In acute or chronic overdose, the glucuronidation and sulfation pathways become saturated. When this occurs, more of the toxic metabolite NAPQI is formed by CYP450-mediated N-hydroxylation. When glutathione stores are depleted with increased exposure to acetaminophen, NAPQI accumulates resulting in liver damage [13].
Pharmacokinetic studies in patients with severe liver disease have shown an increase in half-life, an increase in the AUC, and decreased plasma clearance [14–16]. With an increased acetaminophen exposure, patients are at higher risk of overdose and subsequent toxicity. Acetaminophen is contraindicated in patients with severe hepatic impairment or severe active liver disease and should be used with caution by limiting the total daily dose to no more than 2 g/day in patients with mild to moderate hepatic impairment or active liver disease.
Opioid Analgesics
The metabolism of oxycodone depends on oxidation by CYP3A4 and CYP2D6, which transform oxycodone to noroxycodone and the active metabolite oxymorphone. An impairment in oxycodone metabolism might occur as a result of decreased liver blood flow and/or decreased liver metabolism. Data from a study involving 12 patients with hepatic impairment following a single dose of controlled release oxycodone 20 mg, the oxycodone AUC was increased by 90% and the half-life was prolonged by 2 h. Oxymorphone AUC values were lowered by 50%. These data suggest that oral oxycodone should be initiated at lower doses in patients with hepatic impairment and/or the dosing interval should be increased in patients with severe liver cirrhosis [17].
Morphine undergoes first-pass metabolism after oral administration and is approximately 30–40% bioavailable. It is also a moderate to highly extracted drug with a hepatic extraction ratio of ~0.7. Decreased total clearance is mostly due to a decrease in liver blood flow and a small decrease in intrinsic clearance. Several studies have shown impairment in the metabolism of morphine in patients with liver disease. In a study by Mazoit et al., morphine’s half-life doubled (201 vs. 111 min) and the clearance decreased by 37% in patients with cirrhosis (hypoalbuminemia, hyperbilirubinemia, and prolonged prothrombin time) as compared to normal subjects after a single dose of intravenous morphine was given [18]. The authors recommend that the dosing interval of morphine may need to be increased by 1.5–2 times in patients with cirrhosis to avoid accumulation and untoward effects. A study by Hasselström et al. reiterates that the metabolism of morphine is impaired significantly in patients with severe cirrhosis (Child-Pugh B or C). In addition to finding an increased half-life (4.2 vs. 1.7 h) and decreased clearance (11.4 vs. 28.0 ml/min/kg), the investigators found the oral bioavailability of morphine in patients with hepatic impairment is likely to be increased (100% vs. 47%) due to decreased first-pass metabolism [19]. These studies suggest that if morphine is given intravenously to patients with cirrhosis, the dosing interval should be increased. For oral administration, a consideration should be made to decrease the dose in addition to increasing the dosing interval.
Hydromorphone is a semisynthetic opioid that also undergoes first-pass metabolism, resulting in low (~24%) bioavailability. Hydromorphone is extensively metabolized via glucuronidation in the liver, with greater than 95% of the dose metabolized to hydromorphone-3-glucuronide along with minor amounts of 6-hydroxy reduction metabolites. After oral administration of hydromorphone at a single 4 mg dose, C max and AUC were increased fourfold in patients with moderate (Child-Pugh B) hepatic impairment compared with subjects with normal hepatic function [20]. This increase in overall bioavailability was likely a consequence of reduced first-pass metabolism. The half-life of the drug in patients with hepatic impairment was the same as that in controls. The authors concluded that a reduction of hydromorphone dose is necessary in patients with moderate liver disease. The pharmacokinetics of hydromorphone in patients with severe hepatic impairment have not been studied. A further increase in Cmax and AUC of hydromorphone in this group is expected. As such, the starting dose should be even more conservative.
Antiemetics
The pharmacokinetics of metoclopramide were studied in eight patients with severe alcoholic cirrhosis (Child-Pugh class C) as compared to eight healthy volunteers [21]. A single 20 mg dose of intravenous (IV) and oral metoclopramide was given. A 50% reduction in clearance (0.34 ± 0.09 vs. 0.16 ± 0.07 L/kg/h, p < 0.05) was observed following both routes of drug administration. The authors concluded the adverse effects of metoclopramide observed in marked hepatic impairment are likely to be due to accumulation of the drug as a result of lowered clearance. A 50% dose reduction should be recommended in patients with cirrhosis. Considering that 20% of metoclopramide is also excreted unchanged in the urine, metoclopramide is best avoided if severe hepatic impairment is accompanied by severe renal dysfunction.
Ondansetron is well absorbed from the gastrointestinal tract and undergoes some first-pass metabolism. Mean bioavailability is approximately 60% and ondansetron is extensively metabolized in humans. In vitro metabolism studies have shown that ondansetron is a substrate for CYP450 enzymes, including CYP1A2, CYP2D6, and CYP3A4 (predominant). In patients with hepatic impairment, clearance is reduced twofold (28.3 L/h. vs. 14.7 L/h) and the mean half-life is increased to 14.3 h compared with 5.7 h in healthy individuals. Specifically, in patients with severe hepatic impairment (Child-Pugh C), half-life is increased to 20 h. In these patients, a total daily dose of 8 mg should not be exceeded [22].
A subsequent study analyzed 19 patients with varying degrees of hepatic impairment from chronic liver disease and compared them to six healthy volunteers after a single intravenous dose of ondansetron (8 mg) [23]. The patients with mild to moderate hepatic impairment had similar pharmacokinetic changes: decreased total plasma clearance (CLp 211–299 vs. 478 ml/min), increase in area under the curve (AUC 446–633 vs. 279 ng/L/h), and a longer half-life (t1/2 9.1–9.2 vs. 3.6 h). The changes were even more apparent in patients with severe hepatic impairment (CLp 96 mL/min, AUC 1383 ng/L/h, t1/2 20.6 h).
Antimicrobials
Cirrhosis has multiple effects on the pharmacokinetic parameters of many antimicrobials. Appropriate antibiotic therapy selection and individualized dosing can contribute to optimal clinical outcomes while decreasing the risk of side effects. When individualizing dosing regimens, one should consider the indication, the site and severity of infection, and the duration of therapy. For example, shorter courses of therapy (<7 days), may not require dose adjustments. In general, dose adjustments should be considered in the setting of decompensated cirrhosis for antibiotics that undergo phase I metabolism, have high protein binding, or are associated with high rates of hepatotoxicity [24]. A review of commonly used antimicrobials in surgical patients that undergo hepatic metabolism is included below.
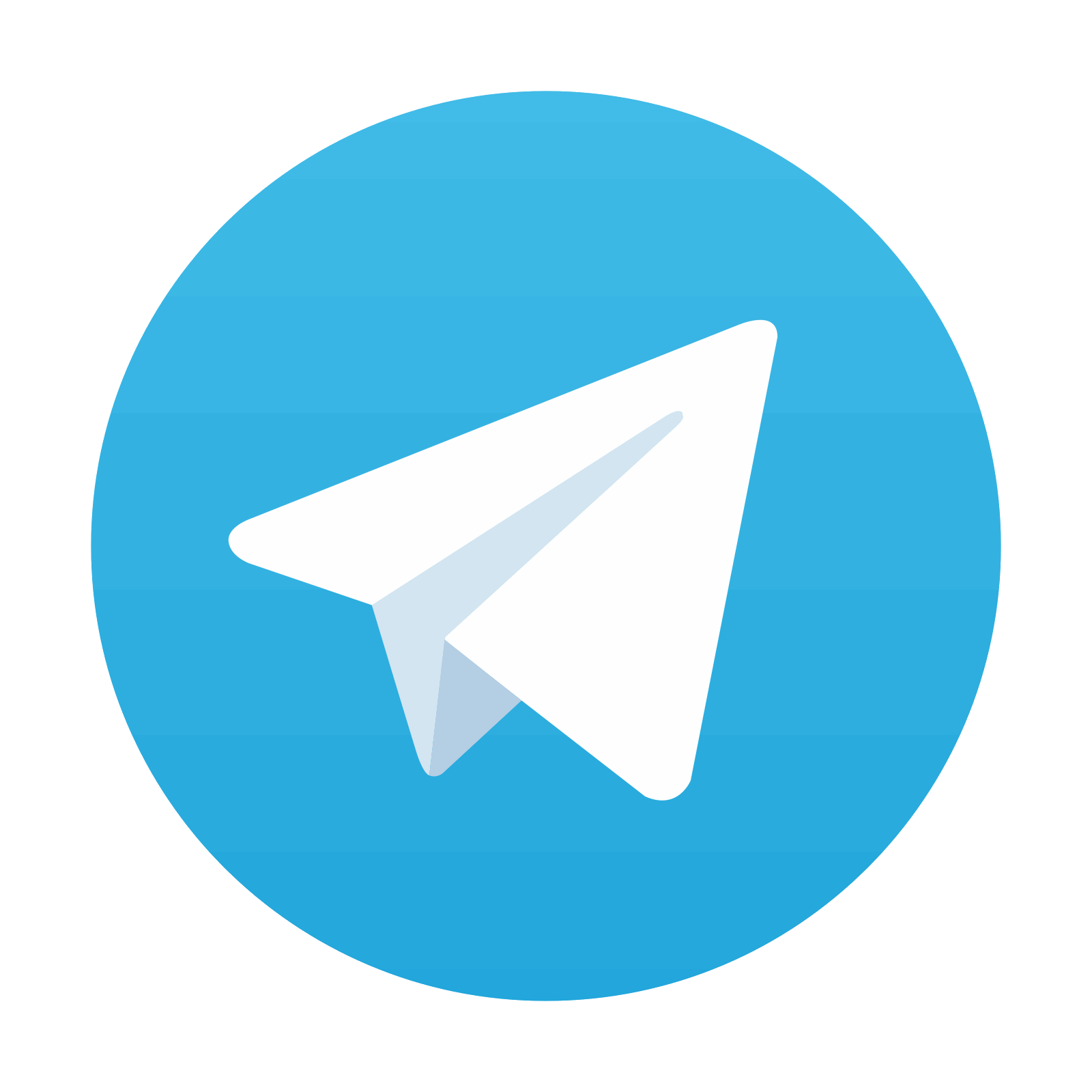
Stay updated, free articles. Join our Telegram channel

Full access? Get Clinical Tree
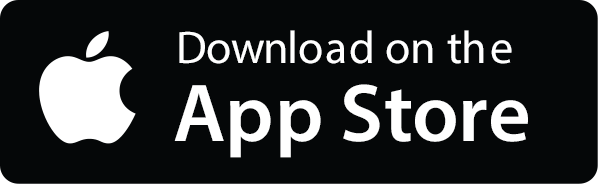
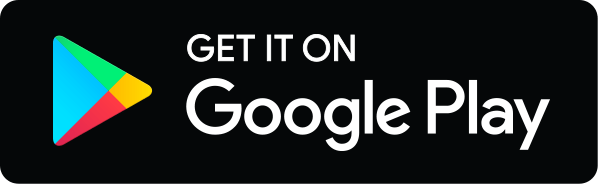