Fig. 2.1
The cholangiocyte glycocalyx forms a biliary surface microclimate pH regulatory system stabilizing the “biliary HCO3 − umbrella.” The 20–40 nm glycocalyx on the apical membrane of a cholangiocyte may trap HCO3 − molecules in order to create an alkaline milieu which keeps bile acids in a negatively loaded state (bile salts) and, thereby, prevents carrier-independent invasion of apolar hydrophobic bile acids into cholangiocytes (and hepatocytes) (Figure modified after Maillette de Buy Wenniger et al. [70])

Fig. 2.2
Regulatory mechanisms of bile ductal secretion. Modification of the primary bile secreted by hepatocytes (canalicular bile) is a finely tuned mechanism operated by cholangiocytes, under the control of pro- (acetylcholine, bombesin, glucagon, secretin, VIP) and antisecretory (dopamine, endothelin-1, gastrin, insulin, somatostatin) signals of hormonal and cellular origin released in the peribiliary microenvironment. These peptides regulate the expression and activation of several ACs expressed by cholangiocytes that modulate the intracellular concentration of cAMP, a second messenger acting on PKA. Once activated, PKA stimulates the secretion of Cl− into the luminal area of the bile duct through the cAMP-dependent channel CFTR. This leads to the extrusion of HCO3 − and the reabsorption of Cl− by the AE2 antiport, thus providing the alkalinization of the bile and its fluidification, in concert with AQPs, which extrude water into the lumen
Genetically determined defects in some of these transport systems are associated with specific disease conditions. For instance, mutations in the CFTR gene inherited as an autosomal recessive trait are responsible for cystic fibrosis (CF). In CF, the gene defect leads to the mislocalization or the loss of function of the CFTR channel. The CF liver disease (CFLD) is a progressive chronic cholangiopathy characterized by focal necroinflammation, vanishing of the bile ducts and portal fibrosis leading to secondary biliary cirrhosis. Derangement of a correct Cl−/HCO3 − excretion in the bile duct lumen due to the CFTR loss of function is responsible for the reduced alkalinity of the bile and, subsequently, for the formation of bile plugs into the biliary tree due to its insufficient hydration. The altered biochemical properties of the bile make it more susceptible to bacterial infections (Figs. 2.3 and 2.4). Recent studies showing that CFTR regulates innate immune responses in the biliary tree have significantly changed the interpretation of the pathogenesis of CF-associated cholangiopathy (see below).



Fig. 2.3
The “biliary HCO3 − umbrella” hypothesis. It is hypothesized that cholangiocytes protect their apical surface against protonated apolar hydrophobic bile acid monomers by maintaining an alkaline pH above the apical membrane. Dysfunction of any of the elements involved in HCO3 − formation might weaken the biliary HCO3 − umbrella and contribute to fibrosing/sclerosing cholangitis. Therapeutic agents such as UDCA or norUDCA stabilize the biliary HCO3 − umbrella. ACh acetylcholine, ATP adenosine trisphosphate, CA carbonic anhydrase, CaCl Ca++-dependent chloride channel, cAMP cyclic adenosine monophosphate, CDCA chenodeoxycholic acid, CFTR cystic fibrosis transmembrane conductance regulator, ER endoplasmic reticulum, P2Y purinergic P2Y receptors, SR secretin receptor, TGR5 G protein-coupled bile acid receptor (The figure is cited from Beuers et al. [13])

Fig. 2.4
Defects of the “biliary HCO3 − umbrella” in various human cholangiopathies? (a) miR-506 is upregulated in cholangiocytes from PBC patients and downregulates AE2 and InsP3R3 leading to impaired biliary secretory functions. (b) A defective biliary HCO3 − umbrella could contribute to explain the enigmatic pathogenesis of various other fibrosing cholangiopathies. Genome screening of patients with PSC has disclosed GPBAR-1/TGR5 as a susceptibility gene that when defective may affect the biliary HCO3 − umbrella. TGR5 is expressed on cilia of intrahepatic and extrahepatic bile ducts, the site where bile duct alterations in PSC are observed (The figure is cited from Banales et al. [11] and Anathanarayanan [5])
2.3.4 Cholangiocytes in Inflammation
As previously outlined, cholangiocytes lining the smallest portions of the biliary tree are, together with HPC, the first site of reaction to liver injury, regardless of its origin. They acquire a reactive phenotype (RDC) characterized by de novo secretion of a number of proinflammatory and profibrotic cytokines (IL-1β, IL-6, and TGF-β, among others), chemokines, and nitric oxide. Thus, cholangiocytes and RDC are active players in the inflammatory reaction leading on one hand to liver repair, on the other to liver fibrosis, and, eventually, cancer [33, 66, 104]. In cholangiopathies, the biliary epithelium is exposed to cytokines and inflammatory mediators produced by infiltrating lymphocytes, macrophages, and hepatocytes as well. These inflammatory cytokines stimulate cholangiocyte proliferative responses, activate fibrogenic processes, and induce histocompatibility antigen expression. In a first study published in 2001, we showed that IL-1β, IL-6, TNF-α, and IFN-γ caused ductular cholestasis by interfering with cAMP-dependent ion transport mechanisms [98]. In a further study, we addressed the role of nitric oxide (NO) in biliary inflammation. NO has many effects depending on the NOS isoforms involved and the pathophysiologic contest. After induction of inducible NOS (iNOS or NOS2), the high concentrations of NO produced may result in the formation of reactive nitrogen oxide species (RNOS) responsible for a number of pathobiological effects. A strong reactivity for iNOS was reported in cholangiocytes of lipopolysaccharide (LPS)-treated rats, a model of sepsis-associated cholestasis. We have shown that LPS, TNF-α, and IFN-γ (proinflammatory cytokines potentially involved in inflammatory cholangiopathies) stimulate NO production in cultured cholangiocytes through an increase in iNOS gene and protein expression [99]. We also showed that micromolar concentrations of NO inhibit AC activity, cAMP-dependent fluid secretion, as well as cAMP-dependent Cl− and HCO3 − transport mediated by CFTR and by AE2, respectively [74]. Interestingly, the cholestatic effects of NO and of proinflammatory cytokines are prevented by iNOS inhibitors and by agents able to block the formation of RNOS [99]. By immunohistochemistry, iNOS expression has been detected in the biliary epithelium and RDC in a series of chronic liver diseases, indicating that these pathophysiologic events may actually occur in vivo [99].
In PSC, biliary structures, including interlobular and septal ducts, strongly express iNOS. The finding that the inhibitory effects of proinflammatory cytokines on cholangiocyte secretion and cAMP production are mediated by iNOS induction and NO production explains the histological findings of the “cholangitis lenta” typical of sepsis, but also links chronic inflammation with the cholestatic manifestation of cholangiopathies prior to the ductopenic phase. Proinflammatory cytokines would induce iNOS expression on cholangiocytes, with production of micromolar concentrations of NO and generation of RNOS. RNOS, interfering with cAMP-mediated fluid and electrolyte transport, may cause a reduction in bile hydration and alkalinity that would further increase bile duct and hepatocellular damage. Positive nitrotyrosine immunoreactivity in PSC samples confirms the potential role of RNOS and protein nitrosylation in vivo [99]. Given the harmful effects of NO on cholangiocyte secretory functions and on DNA repair mechanisms, selective inhibition of iNOS expression is of potential clinical relevance. Furthermore, these data provided the first functional proof of the presence in cholangiocytes of Toll-like receptors responsive to endotoxins. These findings are even more relevant now in the light of the hypothesized changes in innate immunity and in gut microbiome in PSC.
Production of inflammatory mediators in epithelial cells may also be triggered by a primary, genetically determined dysfunction of epithelial cells. Two examples relevant for human diseases are congenital hepatic fibrosis and cystic fibrosis-related cholangiopathy (see Sect. 2.3.5). Congenital hepatic fibrosis (CHF) is a dysgenetic cholangiopathy caused by a genetic defect in fibrocystin, a ciliary protein of unknown function expressed on ductal epithelia [122]. In CHF a low level of inflammatory reaction can be greeted by secretion of proinflammatory cytokines in conditions of cell dysfunction; we have shown that fibrocystin-defective cholangiocytes are able to release chemokines (CXCL1, CXCL10, CXCL12) in a way dependent on a perturbation of the β-catenin signaling ([102]; Strazzabosco M. and Fabris L. personal data). These secretory functions enable cholangiocytes to recruit macrophages to the peribiliary region. Another example of this mechanism (see below) is the secretion of proinflammatory cytokines by CFTR-defective cholangiocytes in CF. These observations support the concept that a genetically determined epithelial cell dysfunction may trigger a persistent and long-lasting, smoldering inflammation unable to resolve liver injury, which may ultimately lead to organ scarring, a process termed as “parainflammation” by Medzhitov [73] or auto-inflammation. We hypothesize that this phenomenon may be relevant in PSC.
2.3.5 Cholangiocytes and Innate Immunity
Because of its central anatomical localization, and the direct vascular connections with the intestine, the liver is constantly exposed to high amounts of gut-derived microorganisms and their toxic products. Cholangiocytes were originally thought to participate to the immune response only by secreting immunoglobulin (Ig) A into the bile [62, 79]. However, an increasing number of studies indicate that the role of biliary tree involvement in immune defenses is much more complex and driven by a number of sensors [22, 107]. Perturbation of these immune responses could lead to the development of severe chronic diseases, such as CFLD [36, 93].
In healthy liver, cholangiocytes cooperate to the self-protection against gut-derived pathogens and toxins, a function driven by several redundant signals, including those mediated by Toll-like receptors (TLRs) [19], nuclear receptors (NRs) [38], and antimicrobial peptides, like defensin and cathelicidin [26, 34]. Cholangiocytes can sense and react to pathogens and endotoxins present in the bile and clear them, by recognizing structural elements of bacterial origin, such as lipopolysaccharides (LPSs), DNA or RNA fragments, and flagellin. Collectively, these bacterial components are known as pathogen-associated molecular patterns (PAMPs) and are detected by the TLRs [10, 53, 94].
2.3.5.1 TLR Signaling
TLRs are a family of nine transmembrane receptors formed by an extracellular domain, rich in leucine and cysteine residues, responsible for the PAMP recognition, and an intracellular domain, Toll/interleukin-1 receptors (TIR), which are able to bind different adaptor molecules (MyD88, Mal, TRIF, and TRAM) [10, 12, 53, 94]. Cholangiocytes constitutively express TLR2, TLR3, TLR4, TLR5, and TLR9 [108, 110]. In cholangiocytes, the pathway activated by TLR4 in response to PAMPs is the best understood, while other TLR-mediated signaling are less characterized together with their ligands. TLR2 is a receptor for the lipoteichoic acid (LTA), a component of the bacterial membrane, and, together with TLR4, it is involved in the protection from C. parvum [83]. TLR3 is a sensor for viral dsRNA and is upregulated in several chronic inflammatory cholangiopathies, such as primary biliary cirrhosis and biliary atresia [50, 80]. TLR9 is a receptor for CpG DNA, short-stranded DNA typical of bacteria, reported to be upregulated in PSC [56]. TLR4 is the main sensor for LPS; following LPS stimulation, TLR4 dimerizes and activates an intracellular signal cascade mediated by transforming growth factor-beta-activated kinase (TAK)1, ultimately resulting in the NF-κB nuclear import. Usually, the NEMO/IKKα/IKKβ complex is bound to the NF-κB subunits p50/p65 and retains them in a cytoplasmic inactive complex. Upon TAK1 activation, one of the components of this complex, the inhibitory protein kappa-Bα (IκBα), is phosphorylated and degraded, allowing the translocation of the NF-κB subunit p65 into the nucleus [8]. Once into the nucleus, p65 stimulates the secretion of a wide range of proinflammatory cytokines and chemokines, such as tumor necrosis factor alpha (TNF-α), IL-1, IL-6, IL-8, IL-12, G-CSF, and LIX [2, 94, 108]. Alternative to NF-κB, TLR4 may also activate the MAPK signaling stimulating secretion of proinflammatory mediators via the activator protein 1 (AP-1) [120]. Notably, in healthy conditions, the liver is equipped with fine, self-limiting regulatory mechanisms that negatively regulate TLR4 or its co-receptors, such as MD-2, thus preventing the initiation of an inflammatory response potentially induced by the presence of PAMPs [1]. Among them, cholangiocytes express high levels of interleukin-1 receptor-associated kinase M (IRAK-M) that negatively regulates TLR4 expression. In contrast, TLR4 is further upregulated in response to inflammatory mediators secreted via NF-κB stimulation. Additional mechanisms of natural tolerance aimed at modulating TLR4 expression through a negative regulatory loop are represented by miRNA. Upregulation of miR-146 occurs in response to LPS stimulation of TLR4, thus providing a partially inhibitory mechanism on the TAK/TRAF complex, which contrasts the activation of the TLR4-induced NF-κB-mediated cytokine secretion [97] (Fig. 2.5). Derangement of one or more of these checkpoints regulating the immune tolerance in cholangiocytes may elicit exaggerated inflammatory responses when pathogens or endotoxins are present in the bile.


Fig. 2.5
TLR and CFTR modulation of proinflammatory cytokine secretion by cholangiocytes. (a) In normal condition, following activation by PAMPs (i.e., LPS), TLRs stimulate the secretion of a range of NF-κB-dependent proinflammatory cytokines, which mount and sustain the immune response. This reaction is negatively regulated by several factors, in particular by PPAR-γ, which stimulates the expression of IκBα, a protein that prevents p65 nuclear import by retaining it in the cytoplasm, and by miR-146, a TLR inhibitor. Moreover, c-Src, a TLR activator, is kept inactive by a multiprotein complex including CFTR and other bridge proteins. (b) In CFLD, the defective CFTR induces a disaggregation of this complex and allows the phosphorylation of c-Src that, by activating TLR, stimulates cholangiocyte secretion of proinflammatory cytokines
Recent studies suggest that, when the apical Cl-channel CFTR is defective, TLR4-dependent inflammatory responses are upregulated. In CFTR-KO mice, experimental colitis induced by dextran sodium sulfate stimulates a brisk inflammatory response centered on the bile ducts that is not observed in WT littermates. This response is characterized by an abundant peribiliary accumulation of CD45+ inflammatory cells mainly encompassing F4/80+ macrophages and NIMP-R14+ neutrophils, resulting from a dysregulation of the TLR4/NF-κB axis [35] due to an aberrant phosphorylation of c-Src [37]. In normal conditions, c-Src is sequestered and maintained in an unphosphorylated state by a multiprotein complex, composed by CFTR together with the bridge proteins EPB-50, Cbp, and Csk. Mutations in the CFTR gene lead to the degradation of this complex. Therefore, in CF patients, disaggregation of this complex allows an aberrant, persistent phosphorylation of c-Src that, in turn, activates TLR4, further stimulating the hypersecretion of proinflammatory cytokines in response to PAMPs (Fiorotto R. personal data). This mechanism has great translational potential. In a mouse model of CFLD (CFTR-KO mice), our group has recently shown that the treatment with PPAR-γ agonists, such as pioglitazone and rosiglitazone, deactivation of the TLR4 signaling, is able to partially abrogate the proinflammatory cytokine production by CFTR-KO cholangiocytes. In CFTR-KO DSS-treated mice, this approach led to a significant reduction in the periductal inflammatory infiltrate, in association with an amelioration of the CFLD phenotype [93] (Fig. 2.5).
These observations may be extended to the treatment of other inflammatory cholangiopathies. Nuclear receptors (NRs) are a wide group of transcription factors containing two highly conserved domains: a central DNA-binding domain and a carboxy-terminal ligand-binding domain. Among the different receptors belonging to this superfamily, the glucocorticoid receptor (GR), the retinoic acid receptor (RAR), the vitamin D receptor (VDR), the liver X receptors (LXRs), and the peroxisome proliferator-activated receptors (PPARs) are those most extensively studied and characterized. All of them share the strong ability to bind the retinoic X receptor (RXR) that is critical for exerting the transcriptional activity [38]. NRs regulate several functions, including cell proliferation and apoptosis, cell homeostasis, carbohydrate and lipid metabolism, and cell interactions involved in tissue repair [111]. In the biliary epithelium, NRs control a range of fundamental physiological functions, from detoxification of bile acids (VDR, FXR) to bile secretion (GR, FXR) [38]. Moreover, VDR, LXRs, and PPARs, acting as negative regulators, are able to transrepress the proinflammatory signals stimulated by PAMPs. PPARs are particularly relevant, since all the isoforms can suppress the pathways activated by the PAMPs-TLRs interaction. PPAR is a family of NRs composed by three isoforms: PPAR-α, PPAR-β/δ, and PPAR-γ, all expressed, at different levels, in normal cholangiocytes [93]. Among them, PPAR-γ is a known modulator of the LPS-induced proinflammatory response, by either directly blocking the production of proinflammatory cytokines or interfering with the activation of NF-κB, STAT1, and AP-1. PPAR-γ may interfere with NF-κB at different levels, by binding the p65/p50 complex, thus inhibiting the p65 nuclearization, or by inducing IκBα expression, which retains p65 in the cytoplasm. PPAR-γ may also inhibit MAPK activation induced by the phosphorylation of c-Jun N-terminal kinase (JNK), thereby preventing AP-1 stimulation.
2.3.5.2 Cholangiocyte Secretion of IgA and Antimicrobial Peptides
In addition to secreting secretory IgA (sIgA) into the bile, cholangiocytes may secrete a variety of peptides with antimicrobial properties. Among them, defensin and cathelicidin provide the first line of the immune defense against Gram+ and Gram- bacteria, fungi, and other pathogens by disrupting their cell membranes (Fig. 2.6). β-defensins (hBD1 and hBD3) are cationic peptides widely expressed by healthy cholangiocytes, in contrast with hBD2, which is undetectable in normal conditions. Antimicrobial activity of hBD1 is particularly effective against P. aeruginosa and Gram- bacteria. Instead, hBD2 biliary expression is upregulated in infectious cholangiopathies, dependent on a TLR-2/TLR-4 mechanism leading to the activation of NF-κB, as shown in acute cholangitis from C. parvum [19]. Moreover, hBD2 expression on cultured cholangiocytes can be induced by stimulation with TNF-α and IL-1β, whose release in the portal tract is increased in several chronic cholangiopathies [49]. These data highlight the concept that whereas hBD1 and hBD3 represent an innate protective mechanism of the healthy bile ducts, hBD2 is involved in specific disease conditions of infectious origin as well as in chronic cholangiopathies (Fig. 2.6). Cathelicidins are another family of antimicrobial cationic peptides, active on a broad spectrum of pathogens, protozoa, and fungi [58]. Cathelicidins are particularly sensitive to PAMPs derived from E. coli [41]. Their expression is stimulated by the interaction of chenodeoxycholic and ursodeoxycholic acids with the nuclear receptors FXR and VDR. Notably, in experimental models of biliary obstruction in rats (bile duct ligation), hBD1 but not cathelicidin expression was induced [51], thus confirming their involvement in specific disease settings (Fig. 2.6).


Fig. 2.6
Cholangiocyte involvement in innate and adaptive immunity. Bile ducts act as a first defense barrier against a variety of gut-derived pathogens and toxic products. (a) In normal conditions, cholangiocytes may secrete a variety of immunologically active factors, including sIgA, cathelicidins, hBD1, and hBD3 and express low levels of HLA-I. These factors cooperate to hinder toxins, fungi, and bacteria eventually present in the bile. (b) Following liver damage of different origin (immune-mediated or infections), cholangiocytes react by secreting de novo antimicrobial peptides, such as hBD2 (in a TLR2/4-NF-κB dependent mechanism), and by expressing de novo HLA-II and CD86, which enable them to behave as APCs. Therapeutic agents acting on nuclear receptors, i.e., FXR and VDR stimulated by ursodeoxycholic (UDCA) and chenodeoxycholic acid (CDCA), respectively, can modulate secretion of cathelicidins
2.3.6 Cholangiocyte Involvement in Antigen Presentation
The ability to behave as antigen-presenting cells (APCs) is an important immunobiological function played by cholangiocytes. In normal conditions, cholangiocytes express only low levels of human leucocyte antigen (HLA) class I, while class II, necessary for antigen presentation to CD4+ T cells, is not detectable [27]. HLA class II expression on the biliary epithelium has been detected in PSC biopsy specimens and in some PBC cases [9, 18]. In vitro experiments in cultured cholangiocytes showed that infection with cytomegalovirus [92] or treatment with proinflammatory cytokines (IL-1, IFN-γ, TNF-α) significantly increased the expression of HLA class I and stimulated the neo-expression of HLA class II. Of note, the membrane receptors CD80 and CD86, which cooperate with HLA class II for the activation, proliferation, and cytokine secretion of T cells, are not constitutively expressed on normal cholangiocytes. However, unlike HLA class II, in vitro expression of CD80 and CD86 has not been induced by cytokine treatment [64], suggesting that cholangiocyte’s ability to act as APC needs a close interaction with other inflammatory cells in order to start or sustain the immunological response that can lead to T cell activation. Immunohistochemical analysis of tissue specimens from patients with PSC and PBC has revealed however CD86 expression in bile ducts, likely indicating a more direct role of cholangiocytes in promoting the necroinflammatory damage featuring these two immune-mediated cholangiopathies [115] (Fig. 2.6).
Conclusions
In addition to the functions classically recognized to normal bile ducts, including bile alkalization, fluidification, and transport, a growing body of data support the involvement of cholangiocytes in inflammation and innate immunity. This is not surprising, considering the capabilities of cholangiocytes to mount a reparative response to many forms of liver damage. Reactive cholangiocytes have the ability to produce a wide range of soluble factors involved in inflammation, such as cytokines, chemokines, growth factors, sIgA, and antimicrobial peptides, along with the ability to express HLA class I and II. The imbalance of signals and pathways orchestrating the immunobiological competence of cholangiocytes is emerging as a new pathogenetic mechanism in specific biliary disease settings. CFLD is paradigmatic of this concept. In spite of the recent advances, a thorough knowledge of the complex immunobiological functions played by cholangiocytes, both in normal and diseased conditions, is still far to be achieved, and further studies are needed.
Acknowledgments
We would like to thank Professor Ulrich Beuers who produced three figures for this chapter.
References
1.
Abreu MT, Fukata M, Arditi M. TLR signaling in the gut in health and disease. J Immunol. 2005;174:4453–60.PubMed
2.
Akira S, Takeda K, Kaisho T. Toll-like receptors: critical proteins linking innate and acquired immunity. Nat Immunol. 2001;2:675–80.PubMed
3.
Alvaro D, Alpini G, Jezequel AM, Bassotti C, Francia C, Fraioli F, Romeo R, Marucci L, Le Sage G, Glaser SS, Benedetti A. Role and mechanisms of action of acetylcholine in the regulation of rat cholangiocyte secretory functions. J Clin Invest. 1997;100:1349–62.PubMedPubMedCentral
4.
Alvaro D, Metalli VD, Alpini G, Onori P, Franchitto A, Barbaro B, Glaser SS, Francis H, Cantafora A, Blotta I, Attili AF, Gaudio E. The intrahepatic biliary epithelium is a target of the growth hormone/insulin-like growth factor 1 axis. J Hepatol. 2005;43:875–83.PubMed
5.
Ananthanarayanan M, Banales JM, Guerra MT, Spirli C, Munoz-Garrido P, Mitchell-Richards K, Tafur D, Saez E, Nathanson MH. Post-translational regulation of the type III inositol 1,4,5-trisphosphate receptor by miRNA-506. J Biol Chem. 2015;290:184–96.
6.
Andersen JB, Spee B, Blechacz BR, Avital I, Komuta M, Barbour A, Conner EA, Gillen MC, Roskams T, Roberts LR, Factor VM, Thorgeirsson SS. Genomic and genetic characterization of cholangiocarcinoma identifies therapeutic targets for tyrosine kinase inhibitors. Gastroenterology. 2012;142:1021–1031.e15.PubMed
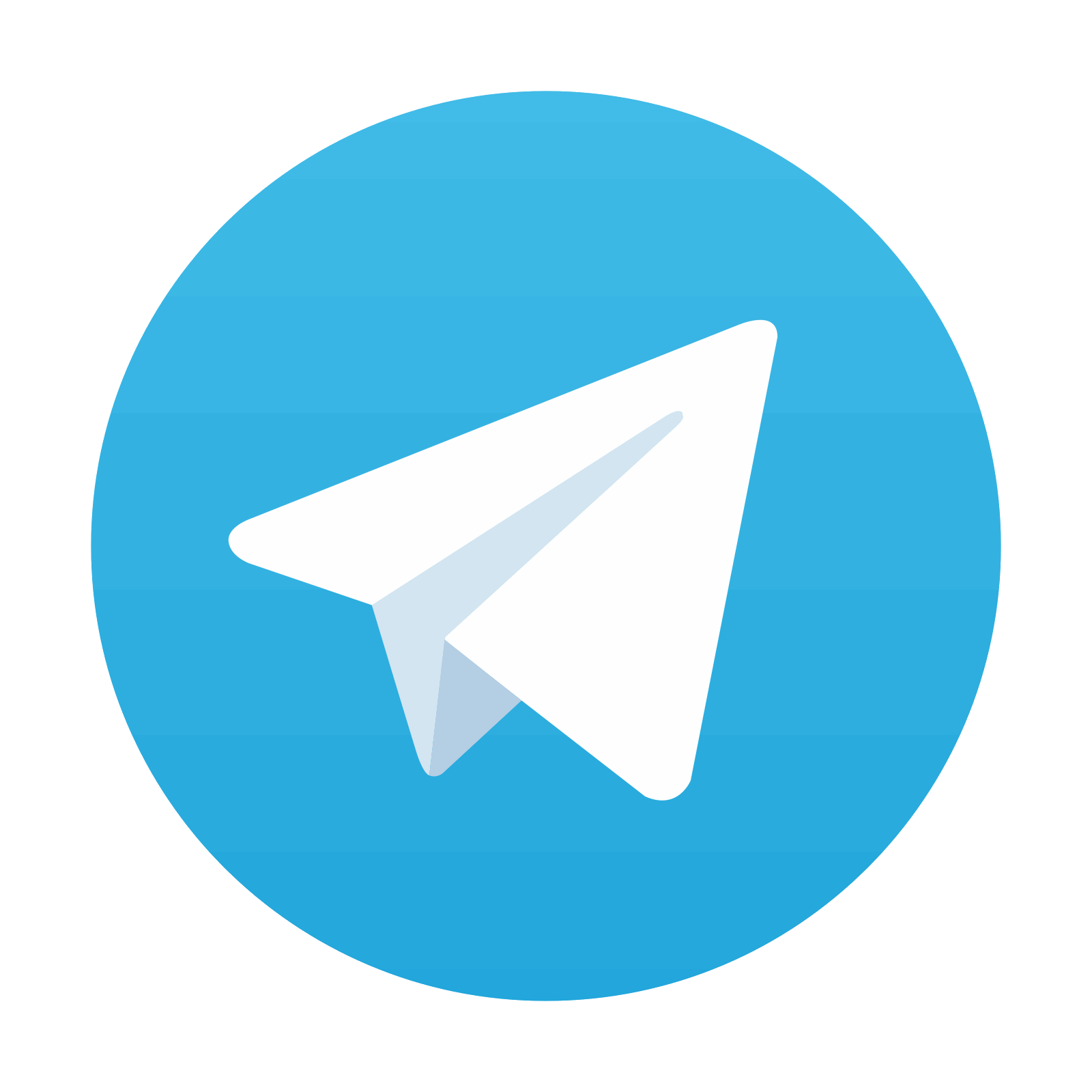
Stay updated, free articles. Join our Telegram channel

Full access? Get Clinical Tree
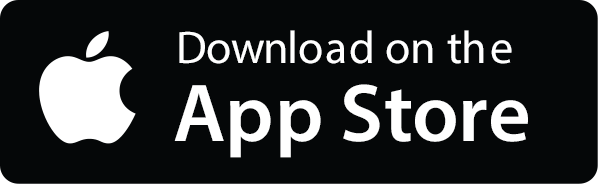
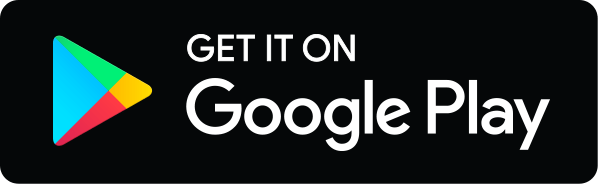