© Springer International Publishing AG 2017
Petar Mamula, Andrew B. Grossman, Robert N. Baldassano, Judith R. Kelsen and Jonathan E. Markowitz (eds.)Pediatric Inflammatory Bowel Disease10.1007/978-3-319-49215-5_44. The Gut Microbiota and Inflammatory Bowel Disease
(1)
Division of Gastroenterology, Hepatology and Nutrition, The Children’s Hospital of Philadelphia, Perelman School of Medicine at the University of Pennsylvania, 3401 Civic Ctr. Blvd., Philadelphia, PA 19104, USA
(2)
Perelman School of Medicine at the University of Pennsylvania, Hospital of University of Pennsylvania, Philadelphia, PA, USA
Keywords
MicrobiomeInflammatory bowel diseaseImmune systemHost-microbe interactionsFecal transplantShotgun metagenomicsDysbiosisAbbreviations
AIEC
Adherent and invasive E. coli
AMP
Antimicrobial peptide
CD
Crohn disease
CDI
C. difficile infection
EN
Enteral nutrition therapy
FMT
Fecal microbiota transplantation
GWAS
Genome-wide array studies
IBD
Inflammatory bowel disease
ILC
Innate lymphoid cells
MAP
Mycobacterium avium subspecies paratuberculosis
NLR
Nucleotide-binding domain and leucine-rich repeat-containing receptor
NOD1
Nucleotide-binding oligomerization domain protein 1
PAMP
Pathogen-associated molecular pattern
PRR
Pattern recognition receptor
SCFA
Short-chain fatty acids
TLR
Toll-like receptor
TNF
Tumor necrosis factor
UC
Ulcerative colitis
Introduction
The inflammatory bowel diseases (IBDs), comprised of Crohn disease, ulcerative colitis, and indeterminate colitis, are chronic inflammatory diseases of the gastrointestinal tract. They are due to an aberrant immune response to environmental factors in a genetically susceptible host. The gut microbiota is thought to be a critical environmental factor in the development of IBD. Crohn disease (CD) can cause transmural inflammation throughout the GI tract and may be characterized histologically by granulomatous formation, as well as the presence of strictures and fistulae. Ulcerative colitis (UC) is typified by mucosal inflammation limited to the colon. A subset of patients are classified as “indeterminate colitis” based on having features of both CD and UC. However, within these classifications, there is significant heterogeneity in disease phenotype. This arises, in part, due to the host genomics, including multiple susceptibility genes, and possibly because of the variable composition of the gut microbiota [1].
There is significant evidence to support the role of gut microbes in the development of IBD. Animal studies of IBD have demonstrated that germ-free animals show little sign of inflammation [2]; however, inflammation develops with exposure to microbes [3]. Adaptive immune responses to bacterial antigens have been shown to lead to the spontaneous development of colitis through immune activation and/or the loss of immune tolerance in various models [4]. From a clinical standpoint, inflammation in CD and UC occurs predominantly in the terminal ileum (in CD) and colon (both UC and CD) where the greatest concentrations of bacteria are found. Antibiotics can have efficacy in the treatment of IBD [5–7], and recently, the therapy using a combination of antibiotics has been shown to be effective in patients with severe colonic disease [8]. Furthermore, the fecal flow exacerbates IBD and surgical diversion of the flow ameliorates the disease [9, 10]. From a more descriptive standpoint, studies have found that there are increased amounts of bacteria in the mucus layer in biopsy specimens of patients with IBD as compared to controls [11]. However, genetic studies have provided some of the strongest support for the role of the microbiota in the development of IBD. Genome-wide association studies (GWAS) have identified >200 genetic risk loci, with 28 shared between CD and UC [12, 13]. Many of the genes and genetic loci identified involve pathways which are critical for the protection of the host against the gut microbiota, such as regulation of the epithelial barrier, microbial defense, and autophagy, as well as pathways involving regulation of the innate and adaptive immune systems [12]. Together, these aberrations support the notion that IBD is due to the inability of the host to protect against microbial invasion combined with an unrestrained immune activation.
Characteristics of the Gut Microbiome
The human gut microbiome is one of the most densely populated bacterial communities on Earth with up to 1011 organisms per gram of fecal weight composed of over 1000 species, most of which are obligate anaerobes [14, 15]. The bacterial concentration, as well as complexity, increases proximally from the stomach and duodenum, where there are approximately 102–103 aerobic organisms/gram luminal contents, to 1011–1012 distally where anaerobic organisms predominate in the cecum and colon [4]. Throughout, the collective genome of the bacteria is 100-fold greater than that of its human host [16]. Indeed, humans should be viewed as a biologic “supraorganism” that is dynamic and carries out functions in parallel or cooperatively [17]. Roles of the microbiota include immune education and metabolism. Although there are over 50 bacterial phyla on Earth, the majority of the bacteria in the human adult gut largely belong to one of four phyla, Actinobacteria, Firmicutes, Proteobacteria, and Bacteroidetes [18, 19].
Most gut microbes are obligate anaerobes, many of which are fastidious and difficult to grow in vitro making traditional culture techniques of limited value in characterizing the composition of the gut microbiota. The development of culture-independent methods, mainly through the use of high-throughput DNA sequencing, has provided new means to evaluate the gut microbiome and its relationship to IBD. There are two primary methods that utilize deep-sequencing technologies to characterize the microbiome. The first approach uses small-subunit ribosomal RNA (16S rRNA gene sequences (for Archaea and Bacteria) or 18S rRNA gene sequences (for eukaryotes)) as stable phylogenetic markers to define the lineages present in a sample [20]. Another approach uses shotgun metagenomic sequencing. This sequences the total community DNA, thereby allowing for the microbial community structure and genomic representation of the community to be evaluated. The genomic community evaluation provides an understanding of the functions encoded by the genomes of the gut microbiota [16]. Metatranscriptomics and metaproteomics provide a deeper understanding of microbial function through direct evaluation of gene expression [21].
These advances in sequencing technologies have allowed investigators to characterize the bacterial composition of the gut throughout different stages of life, a critical step in the study of health and disease. Colonization of the gut begins at birth, and individual characteristics of the gut microbiome begin to arise during infancy and throughout the first year of life. This process is dependent on several factors including the mode of delivery and form of infant feeding. During the first year of life, the human gut microbiome becomes more stable and adult-like [22] concurrent with the introduction of solid foods into the diet [23]. Interindividual differences in the characteristics of the bacterial microbiota observed early in life, within months, persist at 1 year of life [22]. Indeed, interindividual differences in the gut microbiome are the largest source of variance among healthy individuals that appear to be relatively stable over time, at least in the short term [18].
The driving force behind interindividual variation remains to be determined; however, several studies provide indirect evidence that early environmental exposures may play a role. Palmer and colleagues studied the microbiome in 14 full-term healthy infants and discovered significant variability among the children, although marked similarity in bacterial communities in the one set of fraternal twins [22]. This suggests that interindividual characteristics of the gut microbiome may be shaped by environmental factors early in life. These characteristics may persist throughout the host’s life and may be independent of genetic factors [18].
Diet and the Gut Microbiome
As the incidence of certain diseases has increased, there have been many environmental changes that have occurred over the last several decades. These changes in modern lifestyle have been implicated in the alteration of the gut microbiome, including improved sanitation, increase in antibiotic use, less crowded living conditions, decline in H. pylori, smaller family size, vaccinations, refrigeration, decline in parasite infections, sedentary lifestyle, cesarean section, food processing, and diet changes [24].
The development of agriculture and domestication of animals have been major factors in recent human evolution [25] with the resultant changes in diet perhaps altering the host-gut microbiome relationship [26]. Over time in industrialized nations, there has been a reduction in fiber consumption with an increase in simple sugars, fats, and proteins. It has been hypothesized that this change in diet may have altered the interaction of the host and the microbiota in a manner that has played a role in the increasing incidence of metabolic disorders [26]. Furthermore, fluctuations in diet may have consequences for the bacteria and the host, allowing for predisposition to invasion or inflammation [27].
There has been recent evidence demonstrating the relationship between the gut microbiota and diet. An analysis of fecal 16S rRNA sequences from 60 mammalian species indicated clustering according to host phylogeny as well as clustering according to diet (herbivore, carnivore, and omnivore) [28]. Cross-sectional studies using shotgun metagenomic sequencing have suggested that there has been a functional evolution of the gut microbiome in relation to diet [29]. Microbial genes encoding for enzymes involved in carbohydrate and amino acid metabolism are dissimilar between herbivores and carnivores [29].
A study by Wu et al. focusing on the effect of diet on the gut microbiome revealed differences in the impact of habitual long-term vs. short-term diet [30]. Long-term diet, similar to a “Westernized” diet (high in meats and fats, low in carbohydrates), was associated with high levels of Bacteroides and low levels of Prevotella genera. Diets high in carbohydrates but low in animal protein and fat had higher levels of the Prevotella and lower levels of Bacteroides. These results provide an explanation for previously described clustering of individuals into “enterotypes” dominated by Bacteroides and Prevotella based on the composition of the gut microbiota and not correlated to host properties such as age, gender, ethnicity, or body mass index [31]. These observations are also consistent with a study comparing the gut microbiome of children from a village in the West African country of Burkina Faso to those in Europe [32] where the inverse relationship between Bacteroides and Prevotella genera was also noted. These three studies suggest that long-term diet helps to distinguish a gut microbiota community or enterotype that is associated with a “Westernized” diet rich in Bacteroides from an enterotype associated with an agrarian diet where the bacteria of the Prevotella genus predominate. In addition, studies of monozygotic twins to assess host genotype influences on enterotypes showed most twin pairs had similar enterotypes longitudinally, although many of these subjects likely share similar diets and environments [33]. Enterotypes may function as a marker of disease; however, further studies are needed.
Gut Microbiota-Host Interactions at the Mucosal Interface
The alteration of the gut microbiota has had a direct effect on the host’s immune system. Mammalian hosts have coevolved to exist with our gut microbiota in a mutualistic relationship where we provide a uniquely suited environment in return for physiological benefits provided to us by our gut microbiota [34]. Examples of the latter include the fermentation of indigestible carbohydrates to produce short-chain fatty acids (SCFA) that are utilized by the host, biotransformation of conjugated bile acids, synthesis of certain vitamins, degradation of dietary oxalates, and education of the mucosal immune system [34]. Indeed, when viewed as a whole, the “supraorganism” of the gut can carry out enzymatic reactions distinct from those of the human genome and harvest energy that would otherwise be lost to the host. The consequences of these enzymatic reactions suggest that over the millennia, mammalian metabolism, physiology, and disease have shaped and have been shaped by the gut microbiota. Commensal bacteria may also directly inhibit the growth of specific pathogens, such as Clostridium difficile, by competitive inhibition thus preventing an adequate niche for expansion.
Relevant to pediatric IBD, the gut microbiota develops between birth and 3 years of life. In the case of very early-onset IBD, that is during the time period that the child presents with disease or develops symptoms, and each exposure to the infant results in a change in the microbial structure. There has been a drastic increase in the incidence of VEO-IBD, pointing to an environmental contribution to the disease despite the strong genetic drivers identified in this population. The relationship between host genetics and developing microbiome can be seen through germ-free (GF) murine models. GF mice have underdeveloped gut-associated lymphoid tissue. In an environment with specific defined flora, the gut microbiota elicits host-specific T-cell response and differentiation [35]. Concurrently, the aberrant T-cell development of GF mice shapes the gut microbiome, as seen by the different phylogenetic composition of the microbiota in Rag1-deficient mice [36]. Zebrafish models show similar findings, in which Rag1-deficient zebrafish had overgrowth of Vibrio species [37].
In general, the interaction between the gut microbiota and the mammalian host is complex but can be roughly divided into three major categories: the innate immune system, the adaptive immune system, and the intestinal epithelial interface.
The Innate Immune System
The innate immune response is the first line of defense against microbes and is a rapid response. It encompasses receptors that recognize the microbial patterns: pattern recognition receptors (PRRs) that serve as sensors of pathogen-associated molecular patterns (PAMPS) and reside in the lumen of the intestine [38]. The most studied PRRs are the Toll-like receptors (TLRs). PRRs are expressed on many cell types and activate an inflammatory response via NF-ĸβ activation, cytokine production, and recruitment of acute inflammatory cells [39]. TLR signaling in the intestine is important in homeostasis of the intestine through a variety of functions including epithelial cell proliferation [40], IgA production [41], antimicrobial cytokine production and peptide expression, and maintenance of tight junctions [42]. Nucleotide-binding domain and leucine-rich repeat-containing receptors (NLRs), another class of innate immune receptors, have the ability to respond to different stimuli with an inflammatory response. Examples of NLRs include nucleotide-binding oligomerization domain protein 1 (NOD1) and NOD2. NOD2 is highly expressed in monocytes and Paneth cells, and its ligand is common to both gram-positive and gram-negative bacteria. Relhman recently demonstrated via murine models that NOD2 is integral in the interaction between the host and the microbiota and for the development of the intestinal flora [43]. Disruptions in TLR and NLR expression have been associated with intestinal dysbiosis [44, 45].
The Adaptive Immune System
Innate immune signaling through the activation of PRRs or NLRs cannot distinguish between commensal and pathogenic bacteria. The adaptive immune system, involved in both humoral and cell-mediated immunity, has evolved to regulate immune responsiveness by selectively responding to or ignoring individual antigens based on previous encounters [46]. Failure to maintain the latter, known as immune tolerance, results in unrestrained immune activation and subsequent inflammation in the absence of a microbial pathogen, the hallmark of immune-mediated diseases such as IBD. Studies in germ-free mice demonstrate that the gut microbiota plays a critical role in helping to shape adaptive immune function through the production of IgA [47], development of Th17-producing lymphocytes [48], as well as T regulatory cells [49], which play a critical role in the maintenance of immune tolerance [50].
In addition, innate lymphoid cells (ILCs) are innate immune cells without antigen-specific responses. These are functionally associated with T cells with lymphoid lineage, of which there are three types, that also regulate effector T-cell response against commensal organisms. Group 3 ILCs are RORγt+ and produce IL-22 and/or IL-17 with stimulation by IL-23 and IL-1ß. ILCs are regulated by commensal organisms as the production of IL-22 is reduced in the absence of the microbiota [51]. Similar to the innate immune system, multiple gene variants associated with IBD involve components of the adaptive immune response including T- and B-cell regulation and the IL-23/Th17/T regulatory cell axis [12].
Intestinal Epithelium
The intestinal epithelium functions not only as a physical and chemical barrier to separate the luminal gut microbiota from the host, through mucus secretion, for example, but also produces antimicrobial peptides (AMPs) such as defensins, lysozyme, C-type lectins, and cathelicidin, some of which are produced by Paneth cells located at the base of small intestinal crypts [52]. Human genetic variants associated with IBD have been identified in a number of these pathways demonstrating that alterations in host innate immune protection from the gut microbiota play a role in the development of IBD. Among these include genes involved in epithelial barrier function, restitution, and solute transport as well as genes known to have an effect on the biology of Paneth cells [12, 53]. With respect to the latter, genetic polymorphisms in ATG16L1, associated with Crohn disease, lead to alterations in Paneth cells in both mice and humans that have functional consequences predisposing mice to the development of intestinal inflammation in response to bacteria and viruses [53, 54]. Paneth cell products, such as defensins, not only protect the host mucosal surface but can also help to shape the composition of the gut microbiome [55].
IBD and the Human Gut Microbiome
Epidemiological evidence provides strong evidence for the role of the environment in the pathogenesis of IBD. Over the last several decades, there has been an increase in the incidence of inflammatory bowel disease that is too rapid to be attributed solely to genetic factors. The association with residence in or immigration to industrialized nations [56], the consumption of a “Westernized” diet rich in fat and red meat [57], and the use of antibiotics at a young age [58] all implicate an alteration in the gut microbiota as a possible etiologic factor that may be playing a role in the increased incidence of IBD. Further support of this notion is the “hygiene hypothesis” suggesting that humans living in more industrialized societies are exposed to fewer microbes or less complex microbial communities at an early age leading to the development of an immune system less able to “tolerate” exposure to the microbial-laden environment in later life resulting in inappropriate immune activation [59].
Several theories have been suggested to explain the role of the gut microbiota in the pathogenesis of IBD: (1) specific microbial pathogens that induce intestinal inflammation, (2) host genetic defects in containing commensal microbiota in combination with defects in host mucosal immunoregulation, and (3) dysbiosis of commensal microbiota [4]. Multiple studies have been performed evaluating the role of specific bacteria in the development of IBD, such as E. coli and Mycobacterium avium subspecies paratuberculosis (MAP). In CD, there have been consistent findings of increased mucosa-associated E. coli in both the ileum and colon. The E. coli isolated in CD is often an adherent invasive E. coli (AIEC) phenotype, which is characterized by the invasion of epithelial cells and replication within macrophages [60] without causing cell death and induces the secretion of the tumor necrosis factor (TNF)-α [60, 61]. CD-associated AIEC strains are also capable of adhering to ileal enterocytes in patients with CD, however, not from control enterocytes [62].
MAP has also been implicated as a causal organism in the development of IBD. It is the known cause of Johne’s disease in cattle which, similar to the histologic appearance of human CD, leads to chronic granulomatous enteritis. There have been multiple studies exploring the role of MAP in CD; however, controversy remains whether this organism indeed has a causal role. Some studies have shown remission in patients who have been treated with anti-MAP therapy; however, many argue that this has not proven causality. A large randomized controlled trial using combination antibiotics which have proven efficacy against MAP was performed by the Australian Antibiotic in Crohn’s Disease Study Group [63]. This demonstrated an increase in steroid-induced remission; however, there was no significant effect on long-term maintenance of remission. Another criticism of this study was that patients were not assessed for the presence of MAP prior to initiation of therapy. As further studies are performed in MAP and IBD, perhaps a better understanding of this relationship will come to light [60].
Another consistent finding seen in multiple studies in patients with CD is that the phylum Firmicutes has been shown to be reduced [61, 64–68]. Specifically, Faecalibacterium prausnitzii, a Firmicutes, has been found to be decreased in IBD [69–71]. Furthermore, a decrease in F. prausnitzii was predictive of recurrence of disease in patients with CD undergoing ileal resection. There have also been studies that have shown a decrease in the presence of Faecalibacterium prausnitzii in fecal samples and biopsy specimens [69, 72]. In animal studies, F. prausnitzii can induce an anti-inflammatory response by increasing IL-10 as well as produce short-chain fatty acids, both of which may protect against the development of intestinal inflammation [61]. Concurrent with a reduction in Firmicutes, multiple studies have reported a concomitant increase in the abundance of Proteobacteria (including E. coli) [68, 73, 74] and Enterobacteriaceae [75, 76].
To control for the influence of genetics on the microbiome, there have been several studies performed comparing the microbiota of twin pairs. Dicksved and colleagues compared the intestinal microbiome of identical twins concordant or discordant for CD. Total bacterial diversity was decreased among patients with CD. Within twin sets, both healthy twins and twins concordant for CD had closely matched bacterial community profiles. In comparing the twin pairs discordant for CD, however, there was a difference between the fecal microbiome of those with CD and the healthy twin. This suggests that the structure of the bacterial communities is more closely associated with the disease activity rather than the genetics of the host [77]. In another study focusing on twins, Willing and colleagues characterized gut microbial communities in 40 twin pairs who were concordant or discordant for CD or UC. There were differences in the bacterial communities of patients with CD, and there were phenotypic differences as well among ileal and colonic disease as compared to the healthy subjects. There was a decrease in two genera of core commensals in patients with ileal CD, Ruminococcaceae family (including Faecalibacterium) and Roseburia (a member of the Firmicutes phylum) [78]. Consistent with prior studies, there was an increase in Enterobacteriaceae, including E. coli in some of the patients with ileal CD [79].
The alterations in the gut microbiome that are associated with IBD are often described as being “dysbiotic,” implying that there is a functional imbalance between enteric bacteria with potentially pathogenic influences and bacteria who have a benign or beneficial effect on the host [80]. There is currently no clear evidence to confirm this notion in humans. An alternative explanation is that the observed alteration in the gut microbiome of patients with IBD is simply a consequence of the intestinal inflammatory response without consequence to the host. Additionally, in a human study of pediatric ulcerative colitis, evaluation of normal terminal ileum biopsies revealed a loss of goblet cells, depletion of the mucous layer, and loss of bacterial diversity despite a lack of inflammation in the sampled location, which may be due to a systemic effect to the gut epithelial lining independent of local inflammation [81].
There is, however, evidence for a functional effect of a “dysbiotic” intestinal microbiota in animal models. Investigators studying mice deficient in the immune regulatory transcription factor T-bet observed alterations in the intestinal microbiome that occurred simultaneously with the development of spontaneous colitis. Transfer of this bacterial community induced colitis in wild-type mice [82]. In a follow-up study, the investigators identified the presence of Klebsiella pneumoniae and Proteus mirabilis correlated with colitis in these mice [83]. Mice deficient in another immune regulator, the NLRP inflammasome, also develop spontaneous colitis, the susceptibility to which can also be transferred to wild-type mice [44]. Together, these studies suggest a causal role for the microbiota in IBD.
Diet, IBD, and the Gut Microbiome
Several investigators have examined the association of dietary patterns and the incidence of IBD [57, 84]. A systematic review of this subject found consistent results showing that high dietary intake of total fats, polyunsaturated fatty acids, omega-6 fatty acids, and meat was associated with an increased risk of CD and UC; high fiber and fruit intakes were associated with a decreased CD risk; and high vegetable intake was associated with a decreased UC risk [84]. These studies support a potential role for dietary patterns in the pathogenesis of IBD. Together with the recent data characterizing the impact of diet on the gut microbiome and its association with enterotypes [30], it is tempting to speculate that the alteration of gut microbiota community structure through the consumption of agrarian vs. a “Westernized” diet may play a role in either reducing or increasing, respectively, the risk for the development of IBD. This notion would be consistent with the increased incidence of IBD localized globally in more industrialized societies.
Enteral nutrition (EN) therapy, which has shown efficacy in the induction and maintenance of remission in patients with CD [85, 86], may ultimately provide additional support for the role of diet and the gut microbiota in the pathogenesis of IBD. As discussed in a separate chapter, EN is an attractive therapeutic option compared to pharmacological agents, as there are no serious associated side effects. While proven to be effective as therapy in CD, the mechanism of action of nutritional therapy has not been fully characterized. A recent study of pediatric CD patients on exclusive EN (90% of total caloric intake by dietary formula) compared to partial EN (53% by formula) was superior at improving symptoms and quality of life as well as inducing mucosal healing, suggesting that the elimination of solid table foods may be the key to why EN is therapeutic [87]. In addition, the alteration of the gut microbiota may be another possible mechanism of action. In the same study of pediatric CD patients, effective EN therapy changed the microbiota within 1 week and reduced the dysbiosis seen initially [88]. Leach and colleagues evaluated the fecal microbiome of patients with CD who were treated with EN and compared them to healthy control subjects on a regular diet [89]. Prior to initiation of EN, the two cohorts had similar diversity of bacteria present. At the 8-week follow-up, there was a significant decrease in diversity in the patients treated with EN that was sustained for several months following completion of therapy. Nutritional therapy highlights the importance of characterizing the interactions between diet, the gut microbiota, and the mucosal immune system.
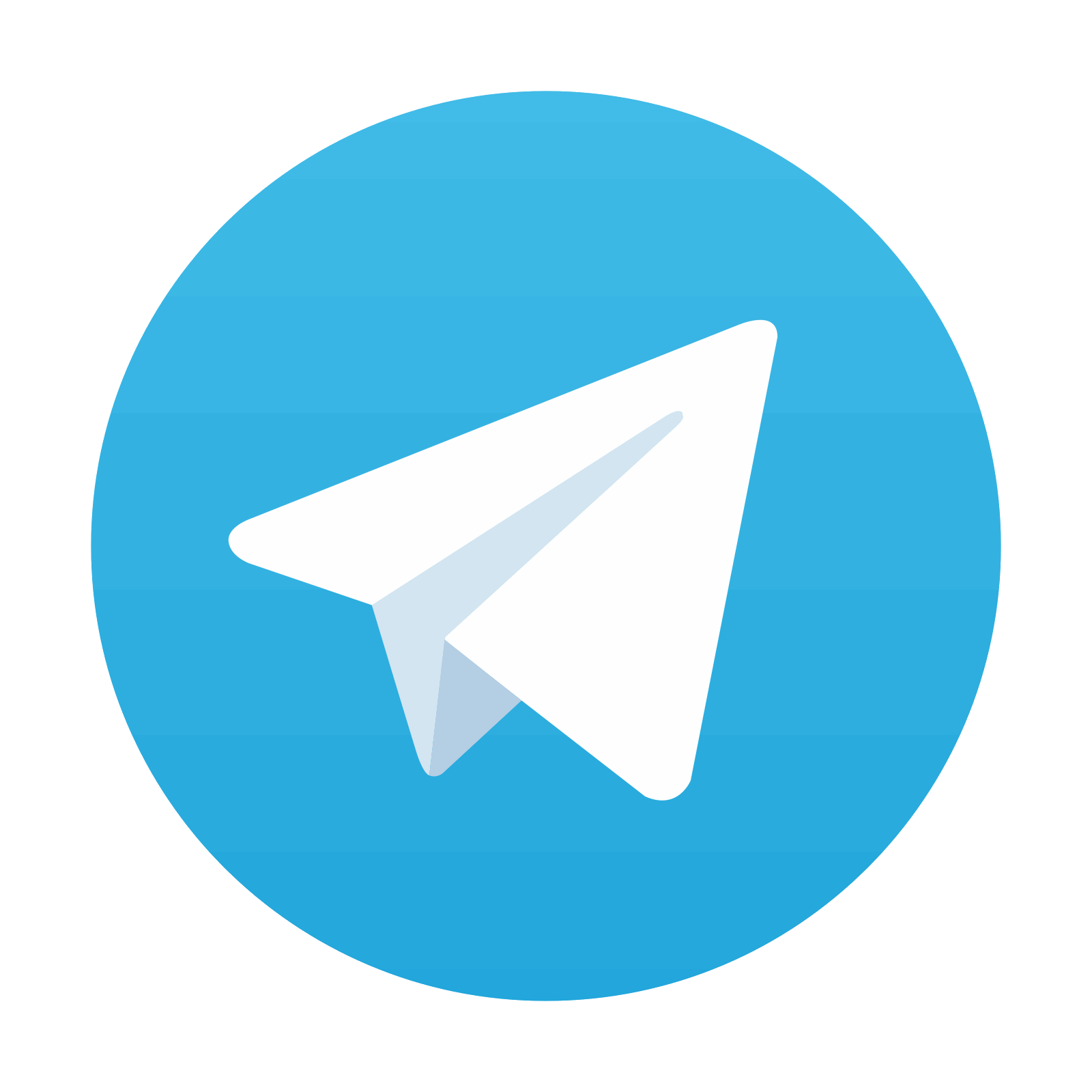
Stay updated, free articles. Join our Telegram channel

Full access? Get Clinical Tree
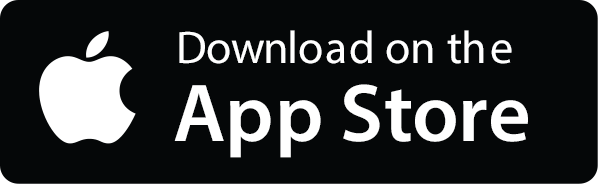
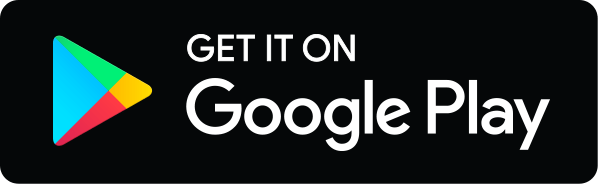