Abstract
Control of food intake and body weight involves multiple pathways and body systems. Recent evidence, in part from the effectiveness of bariatric surgical procedures in treatment of obesity and metabolic disease, but also from the availability of transgenic mice, suggests that the gut can play a predominant role in the long-term regulation of food intake, appetitive behavior, and overall energy balance. Here we review the mechanisms in the gut and the pathways from the gut to the brain that can influence these processes. Information is also included on how these mechanisms and gut-brain pathways may change in obesity and metabolic disease, and the role the gut microbiota might play in mediating these changes.
Keywords
Enteroendocrine cells, Nutrient sensing, Anorexigenic, Orexigenic, Vagal afferents, Cholecystokinin, Glucagon-like peptide-1, Leptin, Ghrelin, Brainstem, Hypothalamus, Limbic system, Bariatric surgery, Gut microbiota
Control of food intake and body weight involves multiple pathways and body systems. The traditional view of energy balance proposes that input from the gastrointestinal (GI) tract is largely involved in short-term regulation of food intake associated with signals arising before, during, and after meals. Other signals related to metabolic state indicate the degree of stored or available energy; these two systems ultimately regulate overall energy balance. However, recent evidence, in part from the effectiveness of bariatric surgical procedures in treatment of obesity and metabolic disease, but also from the availability of transgenic mice, suggests that the gut may play a more predominant role in long-term regulation of food intake, appetitive behavior, and overall energy balance than previously recognized.
62.1
Signals Arising From the Gut in Control of Food Intake
The presence of nutrients in the lumen of the GI tract provides mechanical and chemical stimuli that initiate feedback regulation of GI, pancreatic exocrine and endocrine function, gallbladder function, and also produce an inhibition of food intake. In the postprandial period, intestinal feedback decreases gastric emptying rate and stimulates pancreatic and biliary secretions necessary for efficient digestion and absorption of ingested nutrients. Both humoral and neural pathways mediate regulation of GI, biliary, and pancreatic function in the postprandial period. Consistent with these observations is that the GI tract produces peptide hormones and other modulators that, when injected exogenously, can influence gastric function, pancreatic and biliary secretions, and also regulate appetitive behavior and food intake. Denervation of the gut blunts at least in part these peptide-mediated functions implicating a neural mechanism.
62.1.1
Nutrient Sensing in the Gut
The GI tract is the largest endocrine organ in the body, consisting of many types of enteroendocrine cells (EECs) scattered along the entire length of the tract. EECs are basal-granulated cells dispersed in the epithelium and comprise the endocrine elements of the gut. Gut endocrine cells are distributed from the gastric cardia down to the distal colon and rectum but only make up 1% of the epithelial cell population. The cells are pyramidal or spindle-shaped and generally extend a cytoplasmic process to the gut lumen (i.e., “open” cells). However, in the oxyntic area of the stomach, the cells lie flat on the basement membrane and do not reach the epithelial surface (i.e., “closed” cells). The secretory granules vary between cell types in their immunochemical properties and electron microscopic morphology. Peptides secreted by gut endocrine cells, as in other secretory cells, are synthesized as precursors in rough endoplasmic reticulum (ER) and transported to the Golgi apparatus to be packed in secretory granules. The apical membrane of the open-type EEC is formed by microvilli. These microvilli constitute a sensory apparatus that detects changes in the chemical content of the lumen and modulates the endocrine secretion of the cell appropriately. Many different types of EECs have been identified and categorized by the regulatory peptides that they synthesize and secrete. However, recent data from transgenic mice in which the EECs express fluorescent markers indicate that many EECs express several gut hormones, challenging the long held concept that these cells secrete a single gut hormone. Different EECs are sensitive to different classes of macronutrients such as carbohydrates, fats, or proteins ; however, EECs respond to many other nonnutritive stimuli such as neurotransmitters and factors secreted by bacteria, toxins, and other luminal chemicals. To date, over 30 hormones have been identified in EEC, although the physiological role of all of these potential endocrine or paracrine peptides has yet to be established. Following release from EEC, gut peptides can act systemically by entering the circulation, or locally by a paracrine action. Paracrine actions may involve simple diffusion through the lamina propria. However, there is now good evidence to show that EEC can directly communicate with nerve terminals in the gut wall through a basolateral extension called a neuropod, providing direct evidence for the ability of gut peptides to influence neuronal activity. Gut peptides can potentially influence feeding behavior by entering the circulation and activating receptors in the brain where regions of the blood-brain barrier are leaky, or by activating extrinsic sensory neurons, such as the vagal afferent pathway.
62.1.2
Anorexigenic Signals From the Gut
The recognition that the gut produces anorexigenic signals that inhibit food intake dates from the observations of sham-feeding experiments. Pavlov first reported the effect of sham feeding on food ingestion. He demonstrated that dogs with open gastric fistulas did not experience satiety but would eat continuously for several hours. In a groundbreaking experiment, Smith and Gibbs showed that injection of cholecystokinin (CCK), a hormone synthesized and released from EEC of the small intestine in response to fats and proteins, could inhibit sham-feeding in rats. The importance of intestinal control of food intake stems from this initial observation. There is a good understanding of the way CCK acts to decrease the food intake and it is likely an important physiological mechanism in the control of meal size. Most of the other GI hormones and factors released from the gut in response to ingested nutrients have subsequently been found to also inhibit food intake. The evidence for a physiological role of some of these endogenous factors is not as strong as CCK and is an area of active investigation.
62.1.2.1
CCK
The action of exogenous and endogenous CCK on food intake is quite well understood and there is now overwhelming evidence that CCK plays a physiological role in the regulation of food intake, principally via an effect on meal size and duration. CCK is secreted from I cells located principally in the duodenal mucosa. However CCK-containing EECs are found along the length of the small intestine. Exogenous injection of CCK decreases meal size in many species, including rodents, nonhuman primates, and humans. The ability of CCK to decrease food intake is mediated by an action of CCK at the CCK type 1 (CCK1R) as demonstrated by the much decreased potency of gastrin to decrease food intake, the effectiveness of specific and potent CCK1R antagonists, and by the lack of response to exogenous CCK in transgenic mice with a deletion of the CCK1R. Moreover, the ability of fats and protein in the intestinal lumen, which release CCK from I cells, to decrease food intake is also abolished by CCK1R antagonist, showing a role for endogenous CCK in intestinal feedback control of food intake. Administration of CCK not only has effects to decrease meal size and inhibit ongoing feeding but will also induce the sequence of behavioral changes associated with satiation, including a decrease in activity and in rodents, the onset of grooming behaviors. This supports the idea of CCK as being a physiological satiety factor. In humans, administration of CCK can induce the feeling of satiation and decrease meal size. Administration of CCK1R antagonists in humans increases hunger scores, meal size, and caloric intake.
The use of naturally occurring genetic mutations and the generation of transgenic animals have also helped define a physiological role of CCK in the control of food intake. Experiments in mice with a CCK1R deletion have shown that these mice ingest larger and longer meals. However, there is little change in overall daily food consumption or change in body weight in these mice, even in the long term, although a potential confounder in interpretation is that CCK1R-null mice were generated on an obese-resistant mouse strain. Similarly, CCK-null mice have normal food intake, body weight, and adiposity, although individual meals are larger. A spontaneously occurring 64 kb genetic deletion in CCKA receptors in a strain of rats results in a different phenotype; Otsuka Long Evans Tokushima Fatty (OLETF) rats were originally described as developing obesity and diabetes on regular laboratory chow diet and were selectively bred to provide a consistent phenotype of obesity, hyperglycemia, and late-onset insulin resistance. These rats are insensitive to the ability of exogenous CCK to inhibit food intake. Moreover, these rats eat longer and larger meals, and unlike CCK1R-knockout mice, do become hyperphagic, and gain body weight even when ingesting regular low fat laboratory chow. When OLETF rats were pair-fed to their lean counterparts, they remained lean, suggesting that CCK and the CCK1R may indeed play a role in the long-term regulation of body weight. Loss of CCK1R expression in hypothalamus in rats drives overexpression of neuropeptide Y (NPY) in dorsal medial hypothalamus (DMH). In support of a role for central CCK1R are data from experiments where direct injection of CCK into the hypothalamus decreases food intake in rats.
Plasma levels of CCK rise after a meal or following perfusion of either lipid or protein-rich solutions into the proximal small intestine in humans and experimental animals. However, although CCK may have humoral actions to regulate the gallbladder and pancreas, for example, its action to decrease food intake and stop eating are mediated via extrinsic, vagal afferent pathway. In rats, either systemic capsaicin pretreatment or perivagal capsaicin treatment to functionally ablate vagal afferents blunts the effect of exogenous and endogenous CCK to inhibit feeding. Vagal afferents innervating the abdominal organs express CCK1Rs and CCK induces action potentials in vagal afferent neurons both in vivo and in vitro. Similarly, activation of vagal afferent neurons by lipid is abolished by CCK1R antagonist, suggesting that endogenous CCK also acts to increase vagal afferent firing. Consistent with these observations, CCK activates second-order neurons in brainstem, the initial central nervous system site of integration of signals arising from the GI tract.
62.1.2.2
PYY
Peptide YY (so denoted because of the tyrosine residue at both terminals) (PYY) is secreted from EECs located in the proximal and distal small intestine, and the proximal colon; PYY is released as a 39 amino acid peptide in response to either lipids or carbohydrate in the intestinal lumen. PYY is also released in response to short-chain fatty acids generated by the gut microflora in the colon. The intact PYY molecule is rapidly broken down to produce PYY3-36, the form that inhibits food intake. In rodents, administration of PYY3-36 decreases food intake, and in humans, peripheral infusion of PYY3-36 decreases hunger and 24 h caloric intake. Initial reports on the effects of PYY3-36 to inhibit food intake were difficult to reproduce in some laboratories; however, it seems that the expression of its effects are sensitive to environmental stressors and it is now generally agreed that exogenous administration of PYY3-36 inhibits food intake. Thus, the acute anorectic effects of PYY3-36 have been reproduced in several species including rodents, nonhuman primates, and humans. Like CCK, the predominant effect of PYY3-36 is to decrease the size and duration of meals.
Receptors for PYY belong to the NPY family of receptors; PYY3-36 is a potent agonist for the Y2 receptor subtype and inhibits food intake via the Y2 receptor subtype. PYY3-36 is without effect on food intake in the Y2 receptor null mouse, and administration of Y2 receptor antagonists blocks the action of PYY3-36. The precise mechanism and location of action of PYY3-36 to influence eating behavior are not fully elucidated. PYY3-36 can cross the blood-brain barrier and will, therefore, have access to central Y2 receptor. There is evidence to suggest that PYY3-36 acts on Y2 receptors in the arcuate nucleus of the hypothalamus, a region of the brain crucial in the regulation of food intake and body weight. PYY3-36 directly administered into the arcuate nucleus inhibited food intake and also activates arcuate neurons, as measured by expression of the early gene c-fos. It is thought that the mode of action is on proopiomelanocortin (POMC)-expressing neurons to reduce NPY expression and release, which will in turn increase activity in POMC neurons and reduce appetite.
However, similar to CCK, there are Y2 receptors expressed by vagal afferent neurons innervating the GI tract, and it is possible that this pool of receptor plays a role in mediating the effects of PYY to inhibit food intake. At least two separate laboratories have shown that the anorectic effect of PYY3-36 is absent in vagotomized rats ; however, neither vagotomy nor capsaicin treatment attenuated the effects in mice.
Although there is considerable evidence that exogenous PYY can inhibit food intake, the physiological role of PYY has been harder to establish. Recently, the use of genetically manipulated mice has shed some light on the potential role of PYY. PYY −/− mice are hyperphagic and have an increased body weight and adiposity, even when ingesting a regular laboratory chow diet ; moreover, the phenotype of this knockout mouse can be rescued by administration of exogenous PYY3-36. However, other studies have found only minor changes in food intake and body weight in female PYY-null mice, and yet another study has found no change at all. Overexpression of PYY was without effect on either food intake or body weight in mice. Mice deficient in the Y2 receptor demonstrate a small but significant increase in body weight even when ingesting chow, an effect that was exacerbated when these mice were allowed to ingest a high-fat diet. Thus, the physiological role of PYY to inhibit food intake is not fully established at this time. However, there is considerable interest in the role of PYY released from the gut in mediating the beneficial effects of bariatric surgery on obesity; this will be considered later in this chapter.
62.1.2.3
GLP-1
Considerable attention has recently focused on the role of the incretin hormone, GLP-1, in the regulation of food intake and body weight. GLP-1 is expressed in EEC found throughout the small intestine and the proximal large intestine; in the small intestine, there is an increase in density from the proximal to the distal gut. GLP-1 is released by lumenal nutrients, such as glucose and long-chain triglyceride, and also by other factors including bile acids and short-chain fatty acids. Release of GLP-1 is more complex than some other gut hormones as it is directly regulated by contact of EEC with nutrients and also indirectly via release of GIP, another incretin hormone, from the proximal gut. The relative contribution of these two mechanisms is unclear and may depend on the size of the meal and thus nutrient spread to the distal small intestine.
Exogenous administration of GLP-1 decreases food intake in several species including humans, rats, and mice. The effect of GLP-1 is inhibited by a GLP-1 receptor antagonist and is absent in GLP1-R-null mice. However, the pathway by which GLP-1 mediates effects on food intake is unclear. GLP1-Rs are expressed on vagal afferent neurons, and there is compelling evidence to suggest that peripheral GLP-1, possibly including that released from EEC, acts on this pathway to inhibit food intake. There are numerous pieces of evidence to support this mechanism of action, including reduced effectiveness of exogenous GLP-1 or low doses of the long-acting agonist excendin-4 after capsaicin treatment or subdiaphragmatic afferent vagotomy in rodents. In addition, in humans with vagotomy in combination with pyloroplasty, intravenous GLP-1 has no effect on meal size. Perhaps the most compelling evidence for a role of GLP-1 Rs expressed on vagal afferents comes from experiments in rats where GLP-1Rs in vagal afferent neurons is knocked down using a lentiviral vector. After 3 weeks, the investigators reported an approximate 50% decrease in RNA and protein expression of the receptor, which was accompanied by an increase in meal size and duration in the dark phase, and a complete abolition of the effect of exogenous GLP-1 administration on food intake. Although GLP-1Rs are expressed in a number of areas of the CNS involved in the control of food intake, including the hypothalamus, limbic regions, and brainstem, there is little evidence to suggest that GLP-1 released from EEC influences these populations of receptors in the brain.
However, whether peripheral GLP-1 plays a role in long-term regulation of food intake or body weight is unclear. GLP-1R KO mice have overall normal daily food intake and maintain body weight. Therefore, the role of GLP-1 in long-term regulation of food intake unclear, but similar to CCK may be involved in short-term regulation of meal size and duration. Unlike CCK, long-acting agonists of GLP-1R do reduce body weight in models of increased body weight such as diet-induced obesity in rodents or obese humans, or in type 2 diabetes. Moreover, RYGB and VSG in type 2 diabetics and in experimental animals are accompanied by increase in plasma levels of GLP-1 and have been hypothesized to play a role in the beneficial effects of bariatric surgery on appetite, food intake, and glucose homeostasis.
62.1.2.4
Leptin
Although leptin is largely considered to be an adipocytes hormone, leptin is also found in endocrine cells within the gastric mucosa. As such, it can be considered to be anorexigenic gut hormone. However, the extent to which gastric leptin contributes to overall control of food intake or body weight is unclear. Exogenous administration of leptin inhibits food intake in humans and experimental animals; this effect is likely mediated at least in part via a direct action of leptin on neurons in the hypothalamus. However, vagal afferent neurons express ObR and leptin activate these neurons, as determined in electrophysiological studies and also in cell culture. Deletion of leptin receptors in vagal afferent neurons induces a mild-obese phenotype, changes meal patterns, and markedly reduces the ability of CCK to inhibit food intake. Taken together, the data support a role for gastric leptin and vagal afferent neurons in eating behavior and body weight.
62.1.3
Orexigenic Signals From the Gut
62.1.3.1
Ghrelin
Until 1999, all identified gut hormones produced an inhibition of food intake upon exogenous administration and led to the concept of intestinal feedback inhibition in response to ingested nutrients. However, in 1999, ghrelin was identified in EEC in the gastric mucosa. Ghrelin is an endogenous ligand for the growth hormone secretagogue receptors (GHSR). Ghrelin is secreted from EEC the gastric mucosa; these cells are very numerous, accounting for around 20% of the total EEC cell population in the gastric mucosa. It is interesting to note that these are of the closed type suggesting that they may not be directly regulated by luminal stimuli (in contrast to the majority of EEC found in the small intestine). Unlike other gut hormones that are released by the presence of food and initiate intestinal feedback inhibition of gut function and food intake, fasting elevates circulating levels of ghrelin and feeding decreases plasma levels of ghrelin. Indeed, plasma levels of ghrelin are highest right before the onset of a meal in humans and in rodents. Moreover, the postprandial decrease in plasma ghrelin does not seem to rely on exposure of the gastric or intestinal mucosa to nutrients, but rather is likely to be neurally mediated. Consistent with these observations, exogenous administration of ghrelin stimulates food intake by inducing meal initiation and also by increasing the number of meals, rather than any change in meal size or duration. The majority of the neural regulation of the oral and cephalic response to a meal, including stimulation of peptides from EECs the gastric mucosa, is mediated by the parasympathetic subdivision of the autonomic nervous system; however, it is interesting to note that the release of ghrelin seems to be regulated by the sympathetic innervation.
Ghrelin is a 28 amino acid peptide that undergoes considerable posttranslational modification. There is n-octanoylation (i.e., acylation) of the serine residue in position 3, but both the acylated and desacylated form of ghrelin seem to occur naturally. However, the biologically active form of ghrelin to inhibit food intake is only the acelyated form. In addition, there is evidence that other long-chain fatty acids can substitute for the octanoic acid. It is of interest to note that ghrelin is synthesized and stored not only in EECs in the gastric mucosa but also in neurons in the central nervous system, including those involved in the regulation of food intake and appetite in the brainstem and hypothalamus.
Ghrelin can have direct effects on neurons within the hypothalamus to initiate meals. Ghrelin receptors are expressed by NPY/AGRP neurons in the arcuate nucleus of the hypothalamus; injection of ghrelin into this region results in the onset of a meal, supporting this as a site of action of endogenous ghrelin. Ghrelin receptors (GHSR1A) are also expressed by vagal afferents, although the role of this pool of receptors in mediating the effects of ghrelin is highly controversial. Administration of ghrelin inhibits CCK signaling in vagal afferent neurons and subdiaphragmatic vagotomy or capsaicin treatment to ablate afferent neurons inhibits the influence of exogenous ghrelin on feeding. However, subdiaphragmatic vagal deafferentation had no effect on the ability of ghrelin to stimulate feeding. These data suggest that ghrelin activation of vagal afferent neurons may prevent meal termination, while central ghrelin receptors may initiate food intake.
It has been reported, however, that ghrelin −/− mice are of normal body weight and adiposity, and there is no alteration in food intake compared to wild-type mice. However, ghrelin-null mice are resistant to the obesigenic effects of a high-fat diet. Similarly, GHSR1a KO have normal food intake and show no overall change in body weight on chow but, like ghrelin-null mice, are protected from diet-induced obesity, in part due to a decrease in food intake. The location of the receptor mediating these effects is not clear, nor whether gastric ghrelin is playing a role in these phenotypic changes.
62.1.3.2
Endocannabinoids and Lipid Mediators
One of the newer class of mediators generated in the GI tract that alters food intake are lipid mediators, including the endocannabinoids, anandamide, and also oleoethanolamide (OEA). N -acylethanolamines are a group of fatty acids derivatives. Many of these act as agonists at cannabinoid receptors, although there is evidence that these lipid mediators also activate other receptors and ion channels.
There is evidence that lipid mediators can act both within the brain and the GI tract to alter food intake. It is interesting to note that in contrast to other anorexigenic gut mediators such as CCK and PYY that decrease food intake by decreasing meal size, endocannabinoids act via prolonging intermeal interval. Perhaps the best studied of this class of anorexigenic mediators is OEA. OEA is an endogenous fatty acid ethanolamine that is produced in the intestinal mucosa. Levels of synthesis are regulated by feeding, with increased levels in the fed state and a decreased level during a period of fasting. Exogenous administration of OEA inhibits feeding in rodents and also can decrease body weight following long-term administration, at least in rodents.
The evidence suggests that OEA acts via a vagal afferent pathway to inhibit food intake; the decrease in food intake in response to exogenous OEA is abolished by perivagal capsaicin treatment, and exogenous administration induces an increase in neuronal activity as determined by immunochemical localization of fos protein in the nucleus of the solitary tract (NTS), the site of termination of vagal afferent neurons. Moreover, central administration of OEA has no effect on food intake. In short-term culture, OEA can activate vagal afferents and this seems to be mediated via a TRPV1 pathway since it is not present in nodose neurons from TRPV1-null mice and vagal afferent neuronal responses to OEA were temperature-sensitive and blocked by a TRPV1 channel blocker. However, OEA can activate a number of different receptors in addition to TRPV1, including CB1Rs, PPAR, and GPR119, all of which are expressed in the gut and have been shown to be involved in control of feeding.
It is interesting to note that is has been recognized that all effects of intestinal lipid are not mediated by CCK1Rs; infusion of lipid into the small intestine or ingestion of lipid inhibits food intake that is markedly reduced but not abolished be treatment with specific CCK1R antagonists or also in CCK1R-null mice. It is possible that other ligands and receptors, such as lipid mediators and PPAR agonists, may be involved in the pathway by which lipid inhibits food intake. For example, in either CD36 or PPAR-null mice, there is no inhibition of food intake in response to fat. This requires further study but illustrates the complicated nature and possible redundancy of the pathways and mediators involved in intestinal feedback regulation of food intake.
62.2
Vagal Afferent Neurons
Vagal afferent neurons, the cell bodies of which are located in the nodose ganglia, are most densely distributed in the proximal GI tract (esophagus, stomach, and small intestine), although they are located throughout the length of the gut. These nerve terminals are mechanically sensitive and express a plethora of receptors enabling them to sense nutrients, hormones, and other chemical stimuli. The central projection of vagal afferent neurons projects to the NTS with a viscerotopic representation of the gut. These signals are integrated and passed to neurons of the NTS. The NTS, in turn, projects to efferent vagal neurons in the dorsal motor nucleus of the vagus (DMN). Direct vagal afferent-DMN connections have also been described, although their functional significance has yet to be determined. Vagal afferent neurons convey information from GI receptors to the NTS and initiate vagovagal reflexes affecting gut motility and secretion. The vagal efferent innervation of the GI tract originates from two nuclei of the medulla, the DMN, and the nucleus ambiguus (NAmb). The DMN contains preganglionic neurons that innervate the neurons of the myenteric and submucosal plexus of the ENS.
Vagal afferent neurons innervate the smooth muscle and the mucosa of the gastric wall. Meal-related distention of the gastric wall activates mechanosensitive vagal afferent neurons; these neurons are activated by low-pressure distention or stretch, such as would occur as the stomach fills during food ingestion. There is good evidence from studies in rodents and in humans that activation of the vagal afferent pathway by gastric distension will decrease meal size. It is of interest to note that even though EECs of the gastric mucosa do not secrete the hormone CCK, there is evidence from electrophysiological recording of vagal afferent activity that CCK can active gastric mechanoreceptors. It is possible that this sensitizes neurons to gastric distension thus producing even greater inhibition of food intake.
Vagal afferent terminals have been identified in the mucosa of the proximal small intestine. Fine varicose nerve fibers can be identified that terminate beneath the intestinal epithelium and the terminals do not penetrate into the intestinal lumen. Vagal afferent neurons are chemosensitive and respond to changes in pH and osmolality and can also respond directly to glucose. Vagal afferent nerve terminals also innervate the hepatic portal vein; these afferents respond directly to glucose and amino acids. However, it is likely that the majority of information with respect to nutrient signals activates vagal afferent neurons via the release of hormones and mediators from epithelial cells, including enterocytes, EECs, immune cells, and brush cells. For example, activity in vagal afferent neurons innervating the duodenum is increased in response to luminal protein hydrolysates and lipid, but the response is mediated, at least in large part, by release of peptide and other mediators, such as CCK, PYY, and 5-hydroxytryptamine.
An example of this pathway is the gut hormone CCK, which is released from enteroendocrine I-cells of the small intestine in response to lipids and protein-rich meals. CCK activates CCK1R located on vagal afferent terminals in the gut resulting in activation of NTS neurons. The inhibitory role of CCK on food intake is thought to occur as a result of NTS neurons transmitting the information to forebrain regions including the hypothalamus, limbic regions, and the hippocampus. Evidence from decerebrate rats, in which the connections between the forebrain and hindbrain are severed, suggests that the control of food intake can reside in the brainstem alone. In these studies, inhibition of food intake in response to exogenous CCK remained intact. Thus, activation of the vagal afferent pathway by CCK can not only directly inhibits food intake but also stimulates the efferent limb, thus forming the “vagovagal reflex.” This plays an important role in stimulating pancreatic enzyme secretion and inhibits gastric emptying. Collectively, these actions allow regulation of food intake as well as optimal digestion of fat and protein in the small intestine by balancing the capacity to secrete enzyme and bile salt with the delivery of nutrient substrates.
Vagal afferent neurons also express receptors for many other gut hormones, including GLP-1, ghrelin, and PYY, all of which have been shown to influence food intake in rodents and humans. Like CCK, GLP-1, and PYY can activate vagal afferent neuronal discharge, and when administered exogenously can inhibit food intake. However, their role in the physiological regulation of food intake or in mediating the effect of nutritive signals from the GI tract is not fully established. Ghrelin on the other hand activates vagal afferent neurons to stimulate food intake, inhibits pancreatic enzyme secretion and gallbladder contraction, and stimulates gastric emptying by activating the efferent pathway following inhibition of NTS neurons.
Anorectic hormones depolarize nodose neurons (afferent pathway) while orexigenic hormones hyperpolarize these cells. Thus, CCK and leptin directly and acutely induce membrane depolarization in a subpopulation of cultured nodose neurons, while ghrelin directly and acutely induces membrane hyperpolarization in cultured nodose neurons. When CCK was administered to rats after ghrelin treatment, the CCK-mediated depolarization of afferent activity was not observed, indicating that ghrelin can inhibit CCK activation of these neurons. There is also a certain amount of interaction, leptin markedly enhances vagal afferent responses to low doses of CCK.
62.2.1
Reversible Neurochemical Phenotypes
Vagal afferent neurons express a plethora of different receptors, for GI hormones (such as CCK, GLP-1, GLP-2, PYY, leptin, ghrelin), amines (5-HT), and other neuroactive agents (cannabinoids); many of these receptors, classed either as mediating anorexigenic or orexigenic effects, have been shown to be involved in the regulation of food intake. Moreover, vagal afferent neurons also express different neuropeptide transmitters, including glutamine, cocaine, and amphetamine regulated transcript (CART) or melanin concentrating hormone (MCH), which are released from terminals in the NTS to regulate food intake.
Vagal afferent neurons adapt to energy intake by adopting one of two neurochemical phenotypes. After a meal, vagal afferent neurons express a satiety phenotype, characterized by expression of anorexigenic mediators and receptors, including Y2 and CART ; whereas in the absence of food vagal afferent neurons express a hunger phenotype, similarly characterized by expression of orexigenic mediators and receptors, including MCH, MCH1 receptor, and cannabinoid 1 receptor. The phenotypic change is characterized by altered expression of both mediators and receptors, potentially changing the sensitivity of the neurons to circulating hormones and a change in signaling of information to central circuits.
There is good evidence that CCK acts as the “gatekeeper” that regulates the switch between these two phenotypes. The orexigenic phenotype, expressed in fasted animals, can be reversed by the administration of CCK, and similarly, the anorexigenic phenotype, expressed in fed animals, can be blocked by the use of a CCK-1 receptor antagonist. CCK binds to CCK-1 receptors on nerve terminals projecting to the gut initiates a signaling cascade of PKC and MAPkinase, which activates two transcription factors, CREB and EGR-1. These transcription factors are, at least in part, responsible for the synthesis of Y2 receptor and the anorectic neuropeptide transmitter, CART. The presence of CCK is also characterized by a reduced expression of the anandamide receptor, CB-1, and the MCH receptor, MCH-1 ; CCK activation of CREB also appears to play a part in the downregulation of the orexigenic neuropeptide transmitter MC. In the absence of CCK, CB1, MCH-1, and MCH are upregulated, while CART and Y2 are downregulated.
62.2.2
Neuropeptide Transmitters
62.2.2.1
CART
CCK regulates both the expression and the release of CART. CCK-induced CART expression is upregulated within 30 min and is maximal at 2 h following administration of CCK or feeding. It is unclear how quickly secretory vesicles travel from the cell body where CART is synthesized along the axon to the nerve endings feeding the brain stem. It has been reported that axonal transport can travel at a rate of 100–400 mm/day (1–5 μm/s), suggesting that vesicles made in the nodose ganglia could reach vagal afferent terminals innervating the NTS within 30 min of synthesis. This suggests that 1 h after a meal CCK-induced depolarization of VAN would release CART into the NTS, and is therefore likely to control intake of subsequent meals.
It is presumed that CART released from vagal afferent neurons in response to CCK activates neurons of the NTS. There is considerable evidence in favor of this hypothesis. Administration of CART ICV into the 4th ventricle inhibits food intake, inhibits gastric emptying, and potentiates the effects of peripheral CCK. However, injection of CART directly into the medial NTS of fasted rats did not significantly reduce sucrose drinking. Part of the problem with determining the role of CART is that the CART receptor has not been identified making it impossible to predict the sites of action of CART. However, using cultured vagal afferent neurons, it has been possible to demonstrate that CART acts as part of an autocrine-positive feedback loop in vagal afferent neurons. In cultured vagal afferent neurons, blocking CART expression or its release markedly reduced CCK-induced CART and Y2R expression indicating that CART mediates the effects of CCK. CART on its own stimulates the expression of Y2R and CART in vagal afferent neurons, without influencing the expression of CB1, MCH1, or MCH. The lack of effect on the orexigenic genes suggests that on its own, CART might not be particularly effective in influencing food intake after a prolonged fast; however, in combination with CCK (which downregulates the orexigenic genes), CART could prolong and enhance the satiety effects of CCK. This has been shown in lean rats, whereby after a prolonged fast, peripheral CART did not inhibit food intake; whereas after a short fast CART enhanced and prolonged the anorexic effects of CCK.
62.2.2.2
MCH
MCH expression is negatively regulated by CCK. In response to a meal, or in the presence of CCK, MCH expression is downregulated. Experiments using cultured vagal afferent neurons have demonstrated that CCK-induced phosphorylation of CREB inhibits MCH synthesis.
MCH has been localized to brain regions associated with feeding behavior. ICV injection of MCH increases the food consumption, and MCH1 receptor antagonist reduced the food intake. The role of MCH in the NTS has not currently been examined; however, MCH1 receptor has been localized to the NTS, suggesting MCH could play a role in stimulating food intake.
The implications of CART inhibiting food intake and that MCH stimulates food intake when secreted from VAN into the NTS suggest that the previous meal will predetermine the effect of vagal afferent signaling on food intake. After a prolonged fast, secretory vesicles at the synapse will be carrying MCH rather than CART. Therefore, the first meal after a fast will release MCH into the NTS rather than CART. This suggests one possible explanation for the increased duration of the first meal after a fast. By the next meal, MCH synthesis will have been inhibited, and CART secretory vesicles will be at the afferent terminals of the NTS, and action potentials generated by CCK will release of CART. It is possible that this is the reason for the shortened duration of meals following the first meal.
62.2.3
Receptors
62.2.3.1
Y2R
In the absence of food, the expression of Y2R on vagal afferent neurons is downregulated with a half-life of approximately 12 h. In the presence of food or administration of CCK to fasted rats stimulates the increase of Y2 expression in vagal afferent neurons within 2 h. Y2 is predominantly activated by PYY3-36, an anorectic peptide released from L cells in response to a meal that reaches a plateau in circulation after 1–2 h. PYY inhibits pancreatic enzyme secretion, influences gastric emptying, and inhibits food intake.
CCK only stimulates Y2R expression in vagal afferent neurons projecting to the stomach, and not those projecting to the ileum or colon. Since L-cells are found predominantly in the ileum and colon this suggests that CCK regulates the sensitivity of vagal afferent neurons to circulating PYY3-36 but has no effect in regulating the effects of PYY3-36 at its site of release.
62.2.3.2
CB1 and MCH1
sting. This indicates that orexigenic signaling can be amplified progressively as the duration of fasting increases. In response to a meal CB1 mRNA expression is downregulated within 2 h, whereas the abundance of MCH-1R was essentially unchanged 2 h after refeeding. By 5 h, both CB1 mRNA and MCH1R protein expression were abolished.
The presence of MCH and its receptor on VAN suggests the possibility of a positive feedback loop in response to a prolonged fast. However, the role of MCH on VAN has not yet been established. Two endogenous CB1 ligands have been identified, anandamide and 2-arachidonylglycerol (2-AG). Unlike classical neurotransmitters, or many other intercellular signaling molecules, which are stored in vesicles before release, anandamide and 2-AG are synthesized from the remodeling of membrane lipids. Basal levels of 2-AG in the gut are constitutively elevated suggesting that CB1 would continuously activated were its expression not regulated. Conversely, the availability of nutrients alters the levels of anandamide in the gut. After a 24-h fast, rats show increased levels in the small intestine, but not the stomach. Endogenous cannabinoids have been found to reduce GI motility, reduce gastric emptying, and inhibit food intake.
62.2.4
Hormones
62.2.4.1
Leptin
Vagal afferent neurons constitutively express the long form of the leptin receptor. It is unclear whether leptin receptors localized on vagal afferent neurons sense circulating leptin secreted from adipose tissue or the much greater concentration of leptin secreted locally from endocrine cells in the rat stomach. In contrast to adipose tissue that constitutively secretes leptin in response to an increase in adipose tissue mass, the gastric pool of leptin is secreted in response to feeding and administration of CCK-8. It is well established that leptin depolarizes vagal afferent neurons, but it also plays a crucial role in potentiating and prolonging the effects of CCK to regulate feeding behavior, as well as at the cellular level to regulate gene expression and cell activation.
Leptin interacts with CCK at the cellular level by regulating EGR1 abundance in the neuron. On its own, leptin does not stimulate nuclear translocation of EGR1, but it induces EGR1 expression, and potentiates the capacity of CCK to induce EGR1 translocation so that when combined with leptin 1000-fold lower concentrations of CCK are required for EGR1 redistribution to the nucleus. Overexpression of EGR1 in unstimulated cells was also associated with a remarkable potentiation of the action of CCK on both nuclear localization and induction of CART, consistent with the idea that the interaction between CCK and leptin is attributable to increased EGR1 abundance in response to the latter. It seems therefore that leptin acts to sensitize VAN to the effects of CCK.
62.2.4.2
Ghrelin
In addition to inhibiting depolarization of vagal afferent neurons, ghrelin inhibits translocation of transcription factors to the nucleus. Therefore, ghrelin inhibits CCK signaling via phosphoCREB and EGR1. The functional significance of these interactions is to prevent expression of anorectic peptides and prevents the inhibition of the orexigenic peptides, driving a hunger phenotype. In addition, ghrelin inhibits leptin-stimulated phosphoSTAT3 nuclear localization, thereby inhibiting EGR-1 synthesis. The functional significance of these interactions appears to be to desensitize the cells to circulating levels of CCK. The molecular mechanism by which ghrelin hyperpolarizes the cells or prevents nuclear translocation of transcription factors is unknown.
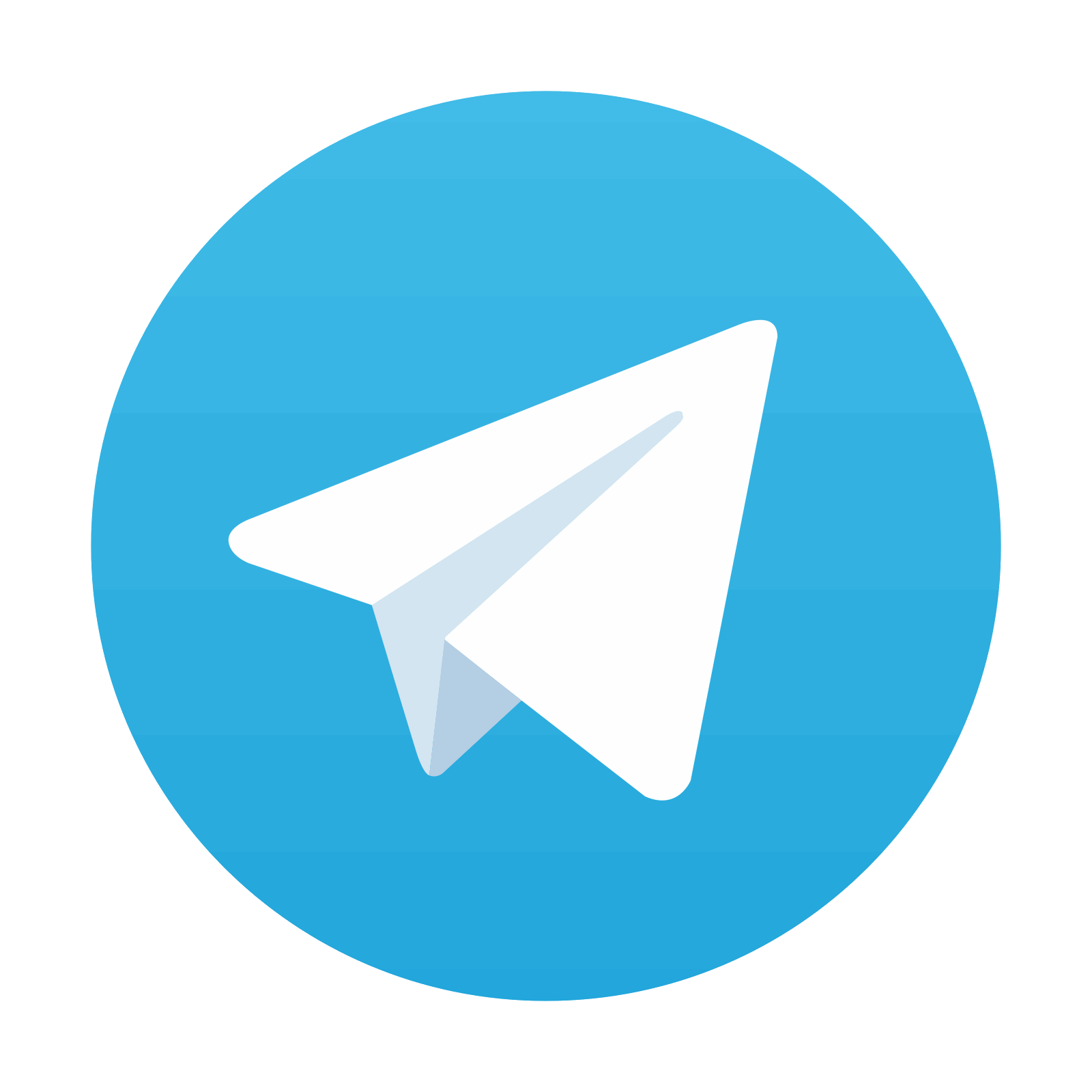
Stay updated, free articles. Join our Telegram channel

Full access? Get Clinical Tree
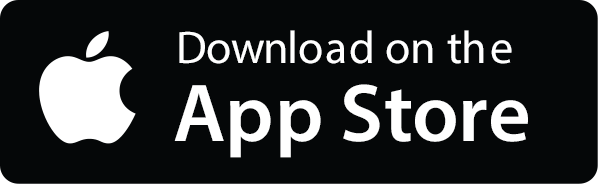
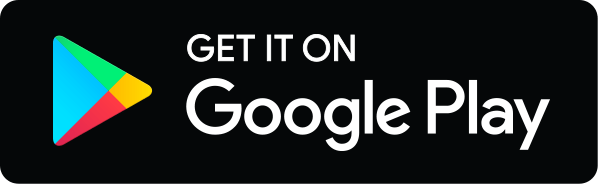