Fig. 4.1
Integration of afferent input and differential efferent sympathetic nerve output
In animals SNA can be assessed by using electrodes to measure nerve firing or by examining the physiological responses of specific organs, including: kidneys [49], heart [50], lungs [51] and liver [52]. Such measurements are often enhanced by use of specific adrenergic antagonists or by use of genetically altered animals, which provide insight into the receptors activated. In humans quantification of SNA is more difficult. In the next section, we will discuss the currently employed approaches and discuss advantages and disadvantages of each.
Measurement of plasma or urinary noradrenaline is sometimes used as a surrogate of total sympathetic outflow but identifies only that portion of noradrenaline escaping reuptake and entering the systemic circulation. Moreover, plasma noradrenaline levels inherently fails to asses regional SNA [53, 54]. Radiotracer-based measurements of noradrenaline spill over from specific organs into the draining venous bed is accepted as a gold standard, but is complicated, requires arterial and venous cannulation and is performed in selective expert centers. Direct recording of sympathetic firing of the peroneal nerve is a elegant method to record muscle sympathetic nerve activity, but again is only performed in specific centers. These methods capture specific elements of sympathetic stimulation, when taken alone, they potentially fail to characterize differential sympathetic innervation of separate organs, the whole body sympathetic activity and contribution of peripheral sites to the whole body SNA [55].
Noradrenaline Spillover
Noradrenaline spill over uses radiotracer technology, is one of the most reliable to study whole body and regional noradrenaline kinetics in humans [56]. Radiolabelled norepinephrine (most commonly [3]) is infused intravenously and regional samples of blood are obtained from veins draining from specific organs. The variable measured is the release of noradrenaline into plasma. The difference in arteriovenous noradrenaline as measured by isotope dilution provides an estimate of regional/organ specific SNA [57, 58]. A limitation of this method is that it measures regional SNA at a single time point and does not allow continuous tracing. This method has proven very powerful, and has been used to document alterations in sympathetic innervation of the heart, the kidneys and skeletal muscle. Recent evidence suggests that at a high rate of nerve discharges there is no longer a linear correlation with the rate of noradrenaline spillover since noradrenaline released from the nerve terminals eventually reaches a plateau [59].
Microneurography
In 1968 Hagbarth and Valibo were the first to report on efficacy of microneurography to measure efferent multi-fiber sympathetic nerve activity (MSNA) [60]. Direct recordings (commonly from the peroneal nerve) reveal burst of nerve activity, synchronous with the heart beat. Observations show while repeat measurements within one subject are highly consistent, there is an up to tenfold variation of SNA from normotensive subject to the next [61–63]. This between individual variability appears to be due physiological variability, rather than variations in recording techniques. Recent refinements have led to single-fiber sympathetic nerve recording in humans [64, 65]. The results from single-fiber recording are supportive of recordings from the whole nerve [66, 67]. Similar to multi-fiber recordings, single-fiber firing is low in humans at baseline, however physiological stimuli and cardiovascular diseases increases single unit discharge rates [68, 69]. MSNA is commonly suggested to be a surrogate of global SNA. While MSNA correlates with noradrenaline spillover, it measures only the patterns of MSNA of the sampled nerve. As discussed above regional differences in sympathetic tone do not necessarily allow conclusions from one organ region to another. There is some evidence that in patients with HF MSNA correlates with mortality [70]. MSNA recording, while a very valuable tool, requires a dedicated laboratory, specialized equipment and skilled personal. For these reasons, it is not used in the clinical setting.
Heart Rate and Blood Pressure Variability
Another commonly employed method is power spectral analysis of heart rate and blood pressure variability. This identifies oscillations in heart rate and blood pressure that are modulated by inputs from the renin-angiotensin system, sympathetic and parasympathetic neurons and by locally released vasoactive factors such as nitric oxide. Obviously, assessment of heart rate variability requires continuous ECG or pulse monitoring, and assessment of blood pressure generally requires constant measurements using arterial cannulation. Fourier analysis and similar techniques allow one to differentiate between the influence of the sympathetic and parasympathetic impact on the heart rate. The analysis is based on the fact that the SNS and parasympathetic nervous system (PNS) operate in different frequency bands. Sympathetic outflow modulates low frequency oscillations, while parasympathetic tone affects both low and high oscillations of heart rate. Thus the ratio of low to high frequency heart rate variability reflects sympathetic cardiovascular modulation. Another approach is to measure baroreflex and chemoreflex sensitivity [71–73]. Patients with HF have increased heart rate and decreased heart rate variability, both of which are associated with increased mortality [74–76]. A major limitation of this method is that no individual heart rate spectrum is selective to the SNA. Factors like age, gender, respiration and baroreflex signals interfere with use of heart rate variability [75, 77]. Similar to the heart rate variability, variability in blood pressure is increased in conditions with increased sympathetic tone and correlates with a heightened MSNA [78]. Absolute values of low frequency blood pressure oscillations provide indirect assessment of sympathetic outflow. Moreover, increased blood pressure variability is associated with higher rates of cardiovascular mortality. However high frequency fluctuations depend on mechanical impact of respiration, whereas low frequency spectral powers are largely due to the complex interaction between vasomotor tone, vascular resistance, humeral and neural factors. Similar to the heart rate variability the use of blood pressure spectral powers are limited by its dependence on various factors, lowering its specificity thus limiting its use in clinical practice [71, 79].
Baroreflex Sensitivity
SNS hyperactivity suppresses the arterial baroreceptor reflex. Consequently the carotid baroreflex is blunted in animals and humans with cardiovascular disease like HTN and HF [80, 81]. At the same time resolution of those morbidities improves the baroreflex sensitivity (BRS). BRS is defined as the change in interbeat interval (IBI) in milliseconds per unit change in BP. This is most often quantified as a slope or gain function [82]. Initially the BRS was assessed with vasoconstrictive agents that increase BP and reflexly decrease heart rate affecting the IBI. New are non-invasive methods to measure the BRS. Here the spontaneous heart rate variability and BP variability are obtained continuously using noninvasive arterial pressure monitoring devices. A major limitation of this method includes that BRS assessment requires a sinus rhythm since small amounts of noise easily disrupts the analysis.
Chemoreflex Sensitivity
Preclinical animal and surgical human studies have outlined the significance of the peripheral chemoreflex in mediating sympathetic hyperactivity, heightened RSNA, and baroreflex inhibition [18, 19, 83–85]. Peripheral chemosensitivity is clinically assessed by measuring the ventilatory response and changes in heart rate or blood pressure in response to either inhibition or stimulation of the peripheral chemoreceptor by manipulating inhaled gas mixtures or applying chemoreceptor stimulating or inhibiting drugs [86–88]. Increased sensitivity of the chemoreflex reflects dysfunction of autonomic cardiovascular control and is linked to development and/or progression of diseases like HF and HTN. Again, give some examples and discuss pluses and minuses.
Identifying Patients in Which Renal Denervation Is Likely to Be Successful
The ability to identify patients with elevated renal sympathetic efferent or afferent nerve activity may help identify optimal candidates for denervation. To guarantee success of RDN, ideal inclusion criteria are: sympathetically mediated disease with heightened sympathetic tone, originating from the renal afferent nerves. Current trials have focused on the broad spectrum of treatment resistant hypertensive patients. Present patient selection focuses to identify truly resistant hypertensive patients. Inclusion criteria for the Symplicity trials are a baseline systolic blood pressure of 160 mmHg or more (≥150 mmHg for patients with type 2 diabetes), despite taking three or more antihypertensive drugs (including a diuretic). However since RDN is a rapidly evolving field newer trials started applying the novel technology in patients with moderate resistant HTN [89].
So far there are at least two screening tests that predict the outcome RDN in patients with resistant HTN. A screening tool that is currently applied by most centers includes the use of ambulatory BP monitoring (24–72 h) to identify patients with truly resistant HTN. This technique also allows to exclude patients with pseudo-resistant HTN [90]. Not surprisingly higher baseline BP predicted more pronounced BP reduction following the procedure. More interestingly impaired cardiac baroreflex sensitivity identified patients with resistant HTN who respond to RDN [91]. In a study by Zuern et al. baroreceptor sensitivity was identified to be the strongest predictor of a patient’s BP response to RDN. Similarly other preprocedural measures of SNA could provide important insight into effects of RDN on patients and increase rate of procedural success. Central sympatholytic agents like clonidine could additionally be used to distinguish responders from non-responders. This is supported by the idea that RDN is more likely to be effective in patients with treatment resistant HTN with a significant component of sympathetic hyperactivation. A marked reduction in blood pressure following the use of a sympatholytic agent is more likely to result successful response to RDN [92].
More efforts are required to preselect patients for RDN in order to maximize response to the novel technology. A selection trying to quantify activity of the SNS rather than disease activity as shown by Zuern et al. opens new ways for effective and targeted screening. Further development of screening tools requires the understanding of how to measure response and how to define success of RDN. Since RDN proves itself to be successful for sympathetically mediated diseases other than HTN, novel tools need to be developed to identify responders for a broad variety of diseases including DM, HF etc..
How to Confirm Technical Success? Measuring Afferent and Efferent Denervation
In humans there are no reports of direct intraprocedural measures of technical success, neither on direct assessment of renal nerve activity during or after the intervention. A more viable and accessible option to measure activity of renal nerves may arise with the use of biomarkers of renal specific nerve activity. Since many of these biomarkers are modifiable they provide targeted measures of risk reduction following therapeutic interventions. Table 4.1 shows a list of potential biomarkers that can be used to confirm technical success.
Table 4.1
A list of biomarkers which have been used in RDN studies or could potentially be applied to measure technical success
Outcome measurement | Biomarker |
---|---|
Efferent deinnervation | Renin, angiotensin and aldosteron levels |
Natriuresis, diuresis, renal vascular resistance and renal blood flow | |
Renal specific noradrenaline spillover | |
Systemic response to renal chemo, or mechanical sensory stimulation | |
Afferent deinnervation | Via interruption of neurogenic reflex loop |
Total body noradrenaline spillover | |
Heart rate/heart rate variability/blood pressure variability | |
Baroreflex sensitivity | |
Central angiotensin levels |
In today’s clinical world, technical success of RDN is defined solely by a reduction in blood pressure and quantified by the extent of BP reduction. It is important to point out that a reduction in sympathetic tone does not necessarily reflect itself in a reduction of BP. This is also true for various other surrogate markers that are clearly linked to the activity of the SNA. In the case of BP as a surrogate outcome, conceivable technical success of RDN does not have to be accompanied by immediate or any BP changes at all [93]. In parts this can be attributed to the complexity of pathophysiology of HTN and the variable role that SNA can play in it. The rate of ineffective reduction in BP following RDN ranges from 10 % to 50 % [94, 95]. Additionally, blood pressure cannot be used as a technical success measure in all populations, as it is not expected to fall following renal denervation in normotensive patients undergoing the procedure in hopes of reducing the renal contribution to elevated sympathetic tone, such as patients with HF, or sympathetically mediated tachyarrhythmias. Since technical success and BP reduction do not have sufficient overlap, other biomarkers and surrogate markers or a combination of both has to be applied to define technical success. Most effective would be a combined assessment of afferent and efferent nerve activity as outlined further below in this chapter.
Unlike in animals intraprocedural and postprocedural direct nerve recordings in humans are limited by the simple fact of inaccessibility of the renal nerves and invasiveness of the procedure. Chinushi et al. first recorded renal nerve activity in animals during RDN [96]. Renal nerve stimulation prior to the ablation increased systemic BP, serum catecholamine and heart rate variability. Renal nerve ablation attenuated BP and HR responses following nerve stimulation. Thus the attenuation or elimination of the different components of the response to renal nerve stimulation following RDN could be the evidence of successful damage to renal fibers and serve as technical success of the intervention. Translated to humans this could mean that technical success eventually could require demonstration of blunted efferent and afferent response to proper stimuli (e.g. exposure to chemosensitive agent fails to trigger afferent signals).
Efferent
In the case of RDN there are various potential biomarkers which arise from direct and indirect effects of efferent deinnnervation of the kidney. Renal efferent nerves regulate circulating volume and sodium as well as fluid homeostasis. Several acute and chronic renal diseases like renal ischemia, renal vascular disease, and end stage renal disease are associated with excessive renal sympathetic efferent activity. Reduction of renal sympathetic efferent signaling suppresses albuminuria, and podocyte injury in aortic insufficiency [97], preventing glomerular hyperfiltration in diabetic rats [98], prevention of angiotensin receptor over expression in HF [99], as well as in acute diseases where renal denervation prevented experimental glomerular nephritis [100] and endotoxemic associated renal injury in mice [101]. Moreover, renal sympathetic nerve traffic may mediate renal ischemic injury [102]. Thus, the renal nerves may mediate several pathologic process linked together by over activity of renal sympathetic signaling. This supports the use renal markers of disease to identify renal hyperactivity and successful thera-peutic renal denervation.
Primary biomarkers of efferent deinnervation include a decreased RSNA with decreased release of noradrenaline and its co-transmitters from renal nerve endings. Since renal efferent nerves innervate all major structures of the kidney, loss of the nerves would explain a decrease in renin release from the juxtaglomerular granular cells, decrease in renal arterial vascular resistance, increase in glomerular filtration rate and increase in renal sodium excretion [103]. Changes in levels of locally secreted neurotransmitters at the terminal endings of efferent renal nerves and levels of RAS components like renin could serve as additional markers of SNA. Several small scale human studies have used noradrenaline spillover technique and renin levels to quantify the remaining renal nerve activity following RDN. In a preliminary study using radiofrequency renal nerve ablation Schlaich et al. demonstrated a reduction of renal noradrenaline spillover (left kidney −48 % and right kidney −75 %), a 50 % reduction in renin activity and increased renal blood flow at 1 month after device based denervation [104]. In a study with ten resistant hypertensive patients, device based renal denervation led to a reduction in noradrenaline spillover of 47 %, 15–30 days after the procedure [95]. In two other small scale studies RDN decreased plasma noradrenaline or catecholamine metabolites but not renin levels [105, 106]. The absent decrease in renin activity was linked to insufficient ablation of renal nerves in order to see a meaningful response. Comparable outcomes were described in patients with resistant HTN and end-stage renal disease [107]. This data shows that primary biomarkers of efferent renal denervation like renin activity and noradrenaline spillover are useful methods to document a decreased sympathetic tone following RDN. While radiotracer dependent noradrenaline spillover technique is not readily available in clinic practice nor is it practicable outside of a research environment, intra or post procedural renal venous blood sampling could be used to measure the concentration of neurotransmitters.
Secondary biomarkers of efferent denervation would include an inhibition of the renin-angiotensin system (RAS), beyond a pure decrease in renin activity. Decreased renin activity reduces angiotensin II and aldosteron levels, resulting in a number of positive effects including decreased global sympathetic tone, vasodilation, improved heart function etc. [108, 109]. Surgical renal denervation in various animal models demonstrated increased natriuresis and diuresis as well as a better response to diuretic neurohormones like ANP, which can be understood as a direct effect of efferent denervation as well as indirect effect of RAS inhibition [110–113]. Human studies on bilateral nephrectomy in renal transplant patients and device based renal denervation in treatment resistant hypertensive patients resulted in not only decreased renin but also angiotensin levels [104, 114–116].
Afferent
Unlike the effects of renal efferent nerve denervation, the effects of afferent denervation cannot be explained by a pure loss of the afferent limb of renal nerves. The renal afferent nerves are part of a neurogenic reflex which regulates the autonomic balance by alteration of the sympathetic tone. In chronic diseases states this reflex is pathologically hyperactive, resulting in over activation of sympathetic tone. These pathophysiological processes do not necessary have to involve the kidney. However CKD serves as a good example to explain the relevance of the renal afferents for this reflex. In CKD, local ischemic and inflammatory changes could increase discharge of local chemoreceptors [32, 100, 117]. As a consequence sensory output from the kidney to the brain is elevated resulting in an increased discharge and potentially also new set point for SNA. Further, successful renal transplantation restores renal function however SNA does not decrease as long as native kidneys remain in place. Removal of bilateral native kidneys restores central sympathetic outflow represented by a decrease in whole body noradrenaline spillover [30, 118]. This speaks most likely for the persistent activity of sensory fibers if native kidneys remain in situ.
Apart from direct nerve traffic recordings, biomarkers of successful renal afferent nerve denervation are related to the interruption of the reflex arc. This includes assessments of the sympathetic nervous system with methods like MSNA, noradrenaline spillover (regional and whole body), baroreflex sensitivity and heart rate variability. There are multiple studies showing a reduction of MSNA in patients who underwent a device based renal denervation when compared with controls. In a study with 20 patients there was a significant reduction in MSNA 3 months following renal denervation procedure [119]. Schlaich et al. measured a reduced whole body noradrenaline spillover and MSNA in patients with CKD undergoing renal denervation [107].
Apart from multi-unit MSNA there is emerging evidence for the use of single-unit MSNA to measure technical success following RDN. Single-unit MSNA recording is technically more challenging, however appears to be more specific and quantitative in patients with cardiovascular disease including essential HTN than multi-unit MSNA [66, 120]. Hering et al. were able to show a reduction in single- unit and multi-unit MSNA in 25 patients 3 months following RDN [65].
While the responder rate to RDN (defined as a reduction in systolic BP of at least 10 mmHg) was up to 100 % in the first landmark trial (Symplicity HTN-1) within the first 12 months following the procedure, the magnitude of blood pressure reduction was more distinct in the time from 6 to 12 months. This finding could potentially be explained by the delayed integration of afferent renal nerve input to the CNS.
Several clinical trials have studied the effects of RDN on the SNA with methods other than MSNA and noradrenaline spillover. Animal studies RDN resulted in an improvement in cardiac and sympathetic baroreflex sensitivity [94, 121, 122]. Interestingly even unilateral RDN was beneficial on the baroreflex function [123]. So far only few studies have studied the effects of RDN on baroreflex sensitivity in humans. Comparable to animal experiments Hart et al. demonstrated improvements in the cardiac and sympathetic baroreflex sensitivity in resistant hypertensive patients [94]. Interestingly these changes were also observed in patients who had no BP response to RDN. Beyond its use as a potential biomarker of successful denervation, impaired baroreflex sensitivity was also able to predict response to therapy [91]. In contrast Brinkman et al. demonstrated no changes in baroreflex function [124]. Notably RDN did not result in any blood pressure changes in this study population.
With an increasing number of clinical trials studying the effects of RDN on the SNA, some studies have not show a reduction in SNA in patients with resistant hypertensive patients following RDN. In a study by Brinkmann et al. RDN did not alter the MSNA, heart rate, blood pressure variability or baroreceptor activity 3–6 months following RDN. Interestingly only 3/10 patients had a reduction in their office blood pressure without any relation to MSNA changes [124]. Similar results on blood pressure and MSNA in humans were demonstrated by Hart et al. 1 and 6 months following RDN. Unlike spontaneously hypertensive rats in a sample size of eight patients only four human subjects had a response in blood pressure to RDN (10 % reduction) which did not necessarily correlate with MSNA [94]. It is possible that response of MSNA to RDN is dependent on baseline MSNA which was lower in these two studies when compared to previously presented studies showing improvement in MSNA [104, 119].
Further biomarkers of successful RDN could be found in the CNS. Today we know that central RAS plays a significant role in controlling systemic sympathetic activity and central integration of the afferent limb of the neurogenic reflex loop originating from the kidney [125]. Central angiotensin II has been implicated to promote development and maintenance of HTN [126] while central infusion of angiotensin II inhibitors attenuated the increases in RSNA and BP in response to renal afferent nerve stimulation [125]. This data suggest central RAS to be a key mechanism for several effects of RDN in humans. Measuring the physiologic response to administration of central angiotensin or measuring central levels of angiotensin are all potential methods to assess the effects of RDN in animals [127]. So far there are no comparable studies in humans advocating the use of new biomarkers or surrogate outcomes.
Despite the limited number of published data a majority of studies point towards a beneficial effect of RDN on SNA. A number of biomarkers provide evidence for a successful reduction in local and general sympathetic tone in animal disease models as well as humans with resistant HTN. As expected for a new technology in its early clinical phase the effects on biomarkers are heterogeneous. Discrepancies between animal and human data can potentially be explained by the difference in pathophysiology of HTN, renal disease as well as the role of SNS for disease development and progression between animal models and humans. An explanation for varying results in humans could be based in the inconsistent selection criteria of resistant hypertensive patients.
Role of Surrogate Markers in Renal Denervation
The initial safety and durability experience for therapeutic RDN document infrequent serious adverse events and durable treatment benefits in patients with elevated blood pressure and drug resistant HTN [128]. Besides a reduction in ambulatory and office blood pressure, animal and human studies provide evidence for a variety of other beneficial effects on cardiovascular physiology and concomitant diseases. Some of the effects are an improvement in reduced heart rate [129, 130], insulin resistance [131, 132], improved exercise tolerance [133] and renal function [134, 135], with attenuation of HF [136–138] as well as improved sleep apnea and cardiac arrhythmia [139–142]. Developing a vision of endpoints and surrogates of treatment efficacy becomes a critical next step in the development and application of RDN. Measuring the success of RDN requires careful differentiation of the technical success (biomarker) from the two measures of successful clinical outcome; surrogate endpoint and clinical endpoint.
Selection and validation of a biomarker and successive advancement to a surrogate endpoint are key processes in the development of new drug and device therapies as shown in Fig. 4.2. Streamlining the use of biomarkers over surrogate outcomes to clinical outcomes requires deep understanding of the pathophysiology of the underlying disease. Thoughtful selection, validation, and application of surrogate endpoints of clinical outcomes offers the potential to bring valuable interventions to clinical care expeditiously. Reduction in sample size and trial duration are important motivations for the use of surrogate endpoints that replace rare or distal endpoints by more frequent or proximate ones.


Fig. 4.2
Effective denervation by the RDN procedure can be evaluated intra- or postprocedural (technical success/biomarkers). The clinical effects on outcome can be evaluated with surrogate outcomes or clinical outcomes
It is obvious that long term endpoints such as mortality are the ultimately desired endpoints of every clinical study. However no study has studied the effects of RDN on hard clinical outcomes. Prudent consideration of clinical surrogate standards and biomarkers of desired outcomes may accelerate appropriate applications of therapeutic RDN. It is unrealistic to demand mortality endpoints for all new promising therapies especially if the procedure like RDN continues to show low serious adverse events. Moreover, some clinical endpoints, such as blood pressure, as a surrogate for mortality and morbidity in HTN, have attained an indisputable status, and several biomarkers convey confidence about prognosis. Table 4.2 summarizes surrogate and clinical outcomes that have been used or can be used for past and future RDN studies.
Table 4.2
A list of surrogate outcomes and clinical outcomes which have been used in RDN studies or could potentially be applied
Outcome measurement | Surrogate outcome | Clinical outcome |
---|---|---|
Efferent deinnervation | Blood pressure (ambulatory, office, blood pressure variability) | All cause mortality, cardiovascular mortality, stroke, MI, angina |
Renal function (GFR, proteinuria, albuminuria etc.) | ||
Afferent deinnervation | Via interruption of neurogenic reflex loop | Via interruption of neurogenic reflex loop |
Blood pressure (ambulatory, office, blood pressure variability) | All cause mortality, cardiovascular mortality, stroke, MI, angina | |
Endothelial dysfunction | ||
Heart rate | ||
Insulin resistance | ||
Renal function (GFR, albuminuria, proteinuria etc.) | ||
Left ventricular mass by ECHO or MRI | ||
EKG LVH with repolarization abnormalities | ||
Tachycardic arrhythmias | ||
Endothelial dysfunction |
Disease Specific Outcomes
In the following part of this chapter we would like to identify disease specific measures which can be used to assess the effect of RDN on a particular disease. We will focus on surrogate outcomes as well as biomarkers which could be used to describe qualitative and quantitative result of RDN. A variety of measures can be used to characterize disease activity however not all of them can necessarily be used to capture the immediate or short-term effects of RDN on a particular organ or organ system.
Cardiovascular
Blood Pressure
Surrogate Outcomes: Ambulatory BP, Office BP, BP Variability, Night Time BP
BP reduction is the most commonly studied surrogate outcome in clinical trials and clinical practice. The first major RDN trials used changes in BP as a surrogate of successful RDN. According to the Symplicity trials patients with an office systolic BP drop of more than 10 mmHg were considered to be responders to the intervention [143]. The positive effect of RDN on arterial BP are most likely a combined result of efferent renal denervation and decreased SNA following afferent kidney denervation. There are several reasons why BP is primarily chosen as surrogate outcome. Control of arterial HTN is a well-accepted risk reduction intervention for primary and secondary prevention of cardiovascular events. There is a well-established continuous and independent relationship between (office and ambulatory) BP and cardiovascular risk. Interventions leading to a reduction of BP have consistently been demonstrated to improve clinical outcome [144]. Based on this relationship it is assumed that successful reduction in BP following RDN will eventually also result in better clinical outcomes. Additional benefit of BP as a surrogate outcome is its universal availability and comparability of results. Ambulatory blood pressure monitoring (ABPM) is more sensitive and specific for cardiovascular risk stratification than office BP measurements [145, 146]. Also, 24 h ABPM correlates better with hypertensive and diabetic end-organ damage than office BP measurements do [147, 148]. Night time BP is related to sympathetic hyperactivation and more indicative of cardiovascular morbidity and mortally [149, 150]. The Dublin Outcome Study studied over 5,000 hypertensive patients and showed that an increase of 10 mmHg in mean night time systolic ABPM increased the cardiovascular mortality by 21 % [151]. Further ABPM is recommended in resistant hypertensive patients to rule out pseudoresistance [152]. Today we know that reduction in BP does not equally affect the outcome, rather the mode of BP control seems to play an important role [153, 154]. It is important to keep in mind that BP alteration and changes in SNA (e.g. MSNA) are not mutually inclusive. While HTN is clearly linked to the activity of the SNA, technical success of RDN does not have to be accompanied by BP changes [93].
RDN trials have investigated both the ambulatory and office blood pressure. While RDN significantly reduced ambulatory and office blood pressure, office BP reductions were more distinct than reductions in ABPM [90, 143]. The landmark trial Symplicity HTN-2 demonstrated a reduction in office BP of 32/12 mm Hg and 11/7 mm Hg in ambulatory 24-h BP [143]. The relative lower change in ABPM may be explained in part by selection criteria for the HTN 1 and 2 trials that did not exclude white coat HTN, thus inherently limiting the opportunity to realize reductions in the post procedure ABPM. Just as entry blood pressure predicts the outcome of renal denervation, entry ABPM is likely a major determinate in its response to the therapy. This relationship is consistent with results from drug treatment trials [155]. Office blood pressure lowering effects have been observed for a period of at least 2 years [128]. Beyond changes in mean arterial pressure RDN also reduces 24-h BP variability [156]. The clinical relevance of standard deviation of systolic BP has been demonstrated by Parati et al. in 109 hypertensive patients, where higher BP variability led to more end organ damage independent from the mean systolic BP [157]. Moreover it appears that visit to visit or day to day BP variability is a similar negative prognostic factor for cardiovascular mortality, stroke and overall mortality [158–160]. Taken together parameters like office and ambulatory BP, as well as its characteristics like BP variability and the pattern/time-dependence of BP reduction are all well suited surrogate outcomes for RDN. Further, risk factors like endothelial dysfunction are clearly linked to increased cardiovascular disease like essential HTN and could be used as accessory markers to measure effects of RDN [161]. Whilst HTN control is the current gold standard in the assessment of technical success in RDN, hard clinical outcomes like mortality may be impacted without BP changes. This urges for additional clinical surrogates in future clinical trials.
Left Ventricular Mass
Surrogate Outcomes: LV Mass by Echocardiogram, ECG or MRI, LV Diastolic and Systolic Heart Function
Left ventricular hypertrophy (LVH) documented by ECHO, ECG and MRI is a common finding in patients with HTN and HF with preserved and reduced ejection fraction. LVH, diastolic and systolic dysfunction are associated with an increased cardiovascular morbidity and mortality [162, 163]. Reduction in LVH by any metric reduces cardiovascular risk, improve mortality in interventional pharmaceutical trials which qualifies it as a surrogate outcome [164–166]. Perhaps of most interest are the well documented associations of ECG repolarization changes and both all-cause and cardiovascular mortality, and the improvements in the mortality following HTN therapy associated with improvements in ECG findings [167, 168]. Herein rests a surrogate, LVH with repolarization abnormalities that might more closely link the therapy with morbidity and mortality changes, however it fails to have utility in predicting response to intervention or guiding intraprocedural therapy. There is also evidence suggesting that LVH regression influences cardiovascular outcomes in HTN independent of blood pressure changes and more strongly than any other risk factor except age [169]. Multiple retrospective and observational ECG and echocardiography studies shown that cardiovascular outcome is better in individuals in whom LVH regresses rather than progresses. This is indicative of the fact that reversal of LVH improves outcome beyond pure blood pressure treatment and reduction [170]. Using this information left ventricular mass can serve as a surrogate of disease progression and its control. This is supported by ECG trial data from the HOPE [168] and the LIFE [171, 172]. Further echocardiographic left ventricular mass predicts independently complications in the general population [173, 174] and patients with coronary artery disease (CAD) [175–177].
Brandt et al. studied the effect of RDN on left ventricular hypertrophy, systolic and diastolic function. In a group of 46 patients with resistant HTN, RDN significantly reduced left ventricular mass and improved systolic as well as diastolic heart function when compared to control [137]. Notably LV mass reduction was also observed in patients who did not respond to RDN with BP reduction, suggesting multiple pathways like fibrosis in which the SNA might contribute to LVH.
Exercise Capacity
Surrogate Outcomes: Exercise Capacity, Chronotropic Competence, Peak Exercise BP and HR
Decreased exercise capacity as well as excessive increases in systolic BP and heart rate during exercise are associated with increased cardiovascular morbidity and mortality [178, 179]. Meanwhile improvement in exercise tolerance is associated with an improved outcome. RDN improves exercise capacity with attenuated blood pressure at peak exercise (21 mmHg) without compromising chronotropic competence [133]. So far there is only one study targeting the HF population directly. In the REACH pilot study seven patients with HF with reduced ejection fraction underwent RDN. This resulted in a trend toward improved 6 min walk test and reduced diuretic therapy over a 6 months follow up period [138]. Unfortunately, the association of exercise endpoints and mortality in HTN is limited, and hardly serves as a convenient surrogate for diseases other than HF.
Arterial Stiffness
Surrogate Outcomes: Arterial Stiffness, Arterial Wall Inflammation?
Arterial stiffness is an established risk factor and independent predictor of cardiovascular morbidity and mortality [180–182]. Decreased aortic distensibility has been linked to worse cardiovascular outcome especially in the hypertensive population [183]. Arterial stiffness can be assessed with the so called augmentation index which shows good correlation with coronary artery disease, all-cause and cardiovascular mortality [184, 185]. Hering et al. measured the arterial stiffness using finger tonometry-derived augmentation index in 40 patients who underwent catheter based RDN which resulted in a significant and rapid reduction of augmentation index [65]. Effects on arterial stiffness were independent from BP and MSNA changes. Interestingly drug studies with RAS blockade reduced arterial stiffness independent from BP changes which could speak for the fact that arterial stiffness is due to structural changes in the vessel wall rather than hemodynamic changes only [186–188]. Similar to EKG and repolarization abnormalities, the several metrics of arterial stiffness may closely associate to reductions of mortality and end organ disability, but will fail to provide guidance in screening patients or making intraprocedural therapy.
Heart Rate
Surrogate Outcomes: Resting HR
Elevated resting heart rate is considered a risk factor for arterial HTN [189], CAD [190], HF [191] and is a well established surrogate outcome of cardiovascular mortality [192, 193]. Pharmacological suppression of heart rate with ivabradine decreased composite outcome of cardiovascular death and hospitalization in a HF population [194]. In a sub population of patients with a resting heart rate >75 ivabradine reduced cardiovascular and all-cause mortality [195]. These data make heart rate a potential surrogate outcome and target for RDN. Repeatedly RDN trials were able to demonstrate a reduction in heart rate [89, 129]. The effect was more pronounced with higher baseline heart rate at baseline. Theoretical candidates, such as changes in heart rate and alterations of heart rate variability are complicated by the concurrent requirements for analgesia and anxiolytics during the procedure. Unfortunately, while reducing heart rate may associate with long term benefits of therapeutic renal denervation, it cannot be valuable in guiding patient selection or intraprocedural therapy.
Electrophysiology
Surrogate Outcomes: Freedom of Atrial Fibrillation and Ventricular Tachycardia and Fibrillation, Proarrhythmia, Ventricular Rate, Premature Ventricular Complex, ST-T Abnormalities
The SNA affects important electrophysiological properties of the heart including chronotropy and dromotropy. Consequently SNA plays a significant role in the development and persistence of atrial and ventricular tachyarrhythmias [196, 197]. An increased sympathetic tone becomes particularly relevant in context of existing co-morbidities like HTN, HF, DM, CAD and SDB. Atrial and ventricular tachyarrhythmias are clearly linked to an increased risk of mortality, making tachyarrhythmias a potential surrogate outcome for RDN [198, 199]. In animal models of tachyarrhythmias RDN resulted in negative chronotropic and dromotropic effects on the heart, leading to an improved rate control during induced atrial fibrillation (AF) and decreased AF susceptibility [140, 141]. In humans RDN improved ventricular rate in AF and decreased inducibility of AF in patients undergoing pulmonary vein isolation (PVI) vs. PVI alone [142]. Potential benefit of RDN on tachyarrhythmias in humans might also be explained by the effects of RDN on comorbidities which are associated with an increased SNA and tachyarrhythmias. Guidelines for clinical trials on atrial fibrillation suggest a variety of outcome measures to validate therapy effects of new drugs and technologies. Suggested clinical outcomes include mortality, stroke, AF related life quality as well as ECG based outcomes like freedom from AF, change in AF pattern, proarrhythmia (ventricular tachycardia, torsades de pointes, atrial flutter, bradycardia, AV nodal block), ventricular rate during AF at rest and during exercise [200].
Comparable to supraventricular arrhythmias RDN was studied in ventricular arrhythmias. In an animal model of myocardial ischemia induced ventricular arrhythmias/fibrillation RDN reduced the occurrence of ventricular arrhythmias/fibrillation [201]. First human studies on RDN and ventricular arrhythmias are restricted to a case series of two patients with HF and electrical storm. The off-label use of RDN decreased incidence of ventricular arrhythmias [202]. Likewise to guidelines for atrial fibrillation the use of hard clinical outcomes and ECG based outcomes can be used to investigate the effect of RDN on ventricular arrhythmias.
Lastly electrocardiographical ST-T wave changes could be used as a surrogate outcome. While a clear relationship with the SNA has not been established, ST-T patterns represented by ST-T wave abnormalities are clearly linked to a higher cardiovascular mortality in patient with underlying cardiovascular disease like HTN, coronary disease etc. [203].
Atherosclerosis and Cardiovascular Events
Surrogate Outcomes: Intima Media Thickness, Inflammatory Markers
Reduction in sympathetic tone could also benefit atherosclerosis and future cardiovascular events. There is an established link between sympathetic hyperactivation and cardiac events like myocardial infarction and angina. Although RDN trials have not yet looked into potential benefits, future trials could use established surrogate outcomes of CAD like carotid intima media thickness and inflammatory markers like CRP [204, 205].
Renal
Surrogate Outcomes: GFR, Proteinuria, Albuminuria, Renal Noradrenaline Levels, Renal Vascular Resistance and Renal Arterial Flow
Increased SNA correlates with worsening renal function [30, 118, 206]. Levels of noradrenaline predict mortality and cardiovascular events in patients with chronic kidney disease (CKD). CKD is an established independent risk factor for cardiovascular and all-cause mortality in the general population [207–209]. Besides the traditional estimate of renal function with the glomerular filtration function (GFR), albuminuria and proteinuria have been established as independent risk factors for all-cause mortality [209, 210]. Thus GFR, albuminuria and proteinuria can potentially serve as surrogate outcomes for RDN. Surgical RDN has favourable effects on acute kidney injury and CKD in a number of different animal models [211]. RDN resulted in improvement of renal hemodynamic and filtration dysfunction caused by chemical toxic or ischemic damage to the kidney. In humans there are currently only several small scale studies looking into potential effects of RDN on renal function. Human trials have studied the effects of RDN on normal and deceased kidneys in resistant hypertensives. Initial landmark studies have used decreased GFR as an exclusion criterion for RDN. Effects of RDN on the kidney function include biomarkers of successful renal denervation like decreased renin secretion and increased RBF [104]. Further Krum et al. demonstrated in a single-arm study an improved GFR in 24 % of patients with resistant HTN and a normal renal function over a 12 months period. In a comparable study with a control group RDN reduced the incidence of microalbuminuria while GFR remained unchanged [134]. Denervation resulted in a decreased proportion of patients with detectable urinary albumin excretion 6 months after the procedure. Other renal vascular hemodynamics could be additional biomarkers of efferent and afferent denervation. Renal vascular resistance and renal blood flow could reflect a reduction in SNA of renal nerves. However despite improved central and peripheral blood pressure, decreased renal vascular resistance, the renal function (GFR) and renal perfusion remain unchanged after the procedure [95, 212].
Subsequent studies investigated the safety and potential benefit of RDN in CKD. Hering et al. studied the short term effects of RDN in CKD three to four patients. RDN reduced the BP however did not result in significant changes of GFR, proteinuria or albuminuria [213]. Three additional studies investigated the effects of RDN in end stage renal disease (ESRD) for up to 12 months [107, 116, 214]. These studies confirm the anti-hypertensive effect of RDN in ESRD without a change in GFR which could suggest a protective effect of this intervention on renal function. Since most studies lack a control group the potentially beneficial effect and its role as a surrogate outcome need to be further investigated in randomized controlled trials.
Metabolic
Diabetes
Surrogate Outcomes: Insulin Level, Insulin Resistance, Fasting Glucose and Glucose Tolerance Test
There is a clear link between activation of the SNS, hyperinsulinemia and development of diabetes mellitus type 2 (DM) [215–217]. Moreover SNA is associated with pathophysiology of metabolic syndrome, obesity and resistant HTN [217]. At the same time the metabolic syndrome and DM are clearly linked to an increased cardiovascular morbidity and mortality [218]. Increased fasting glucose, impaired glucose tolerance and increased insulin levels have been established as surrogate outcomes for the metabolic syndrome and DM.
In animals models of insulin induced HTN, renal denervation improved BP [131]. Mahfoud et al. studied the effects of RDN in humans with resistant HTN [132]. In a population of patients with resistant HTN and metabolic syndrome, 40 % of whom had a confirmed diagnosis of DM2, RDN decreased fasting glucose from 118 to 108 mg/dL, insulin levels from 20.8 to 9.3 μIU/mL and C-peptide levels from 5.3 to 3.0 ng/mL. Further the insulin resistance index as well as a postprandial glucose tolerance test improved by 30–40 % following 3 months after the procedure. Moreover oral glucose tolerance tests improved in the studied population resulting in decreased number of patients with impaired fasting hyperglycemia and impaired glucose tolerance test.
While the association between renal sympathetic denervation in resistant HTN has been documented, neither insulin resistance by HOMA or reduction of insulin levels themselves have been documented as mortality surrogates.
Conclusion
1.
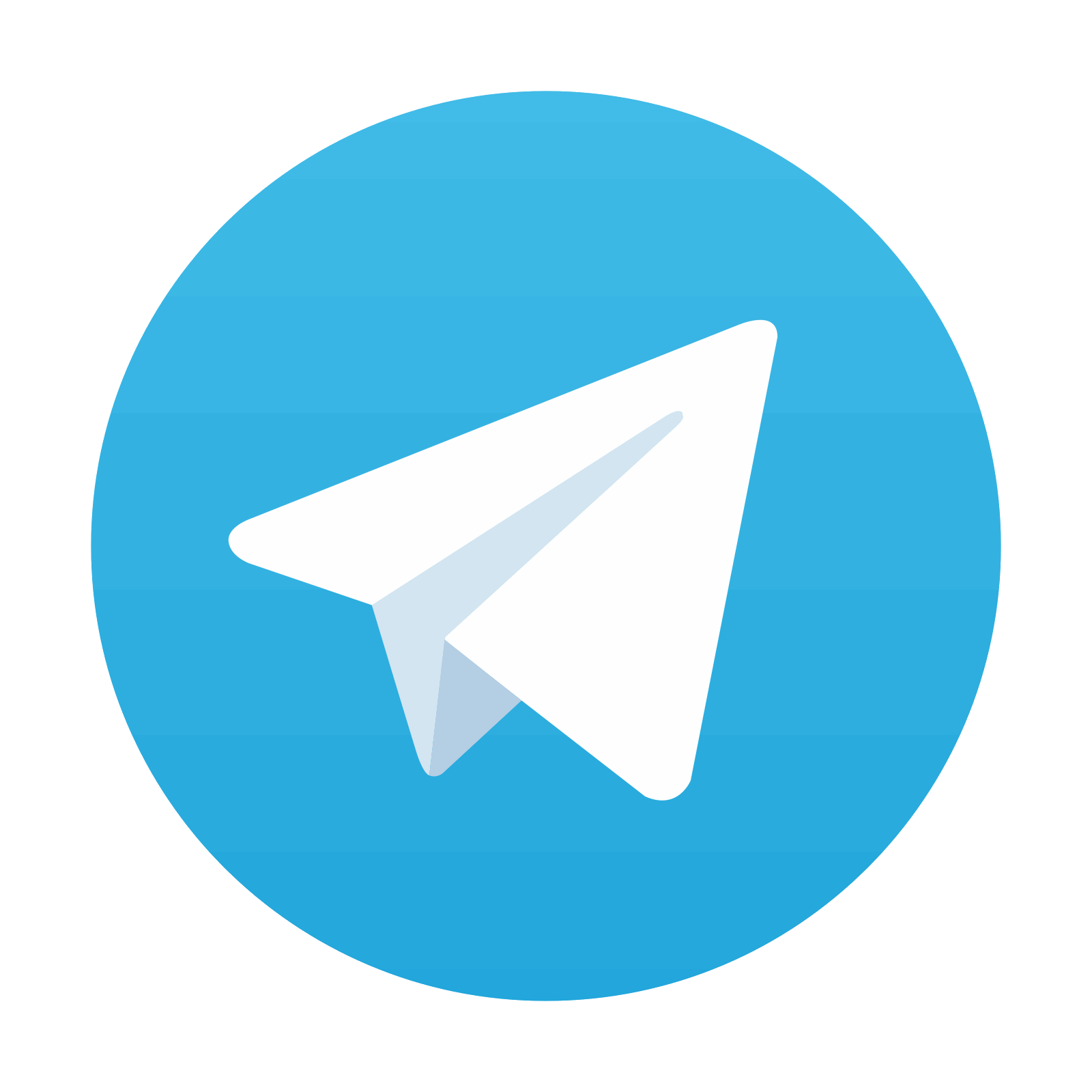
There is no current intraprocedural test of technical success to assist in titration of therapy that both is sensitive and specific as well as provide immediate results to the interventional clinician. The low complication rates and cost of therapeutic renal denervation for treatment resistant HTN render the value for these tools low. However, if more aggressive or potentially toxic interventions or the titration of ablation therapy is associated with renal or vascular toxicity the need for measures of technical success will be more obvious.
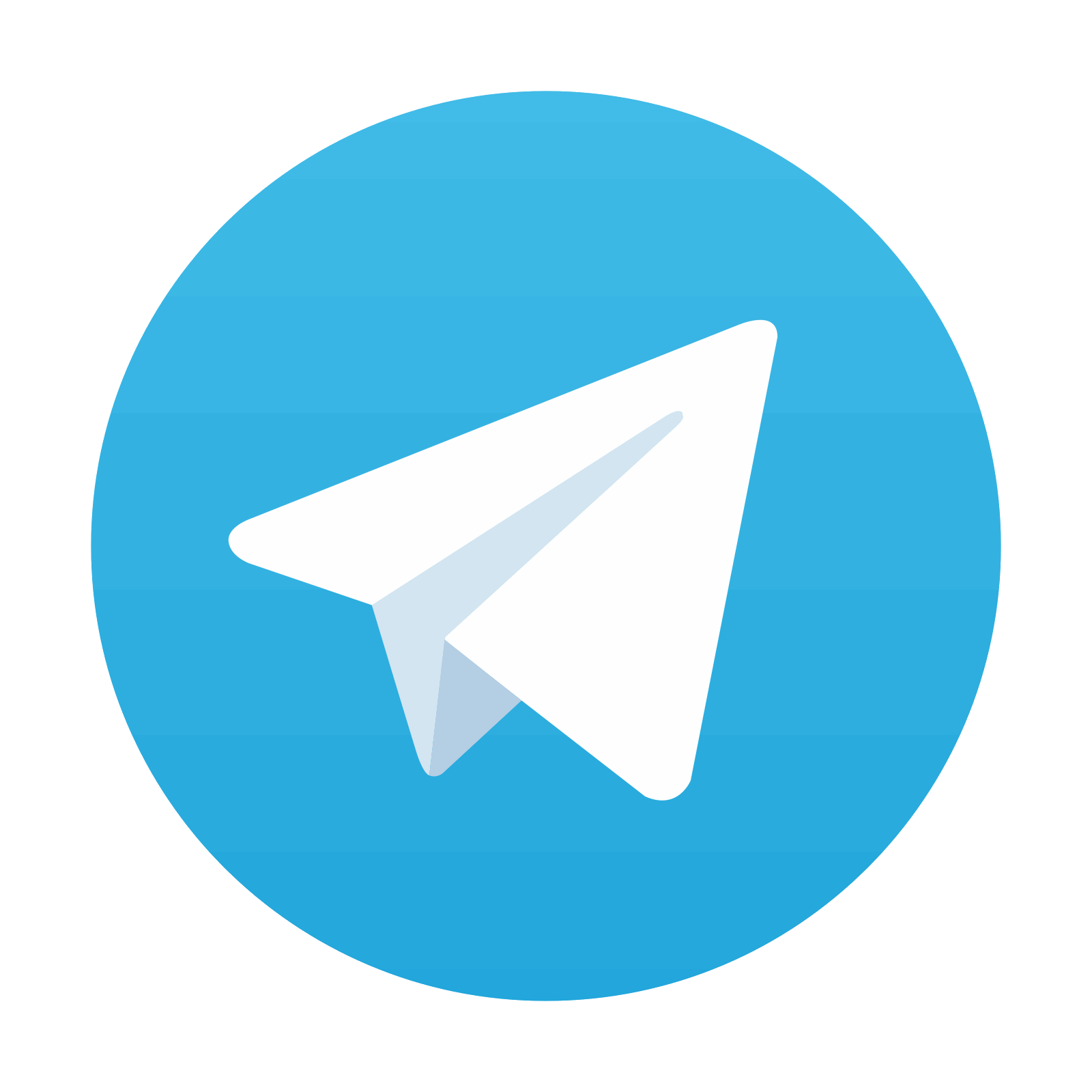
Stay updated, free articles. Join our Telegram channel

Full access? Get Clinical Tree
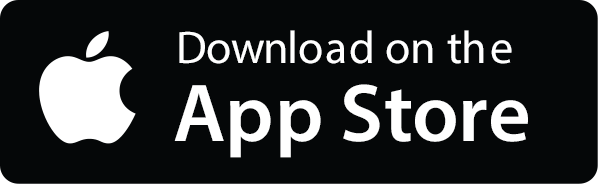
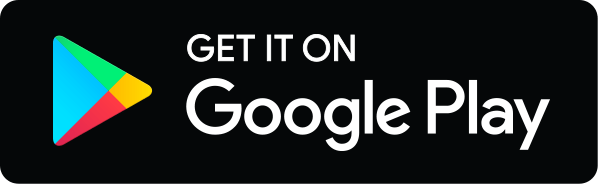
