(1)
Department of Anatomy, Tokyo Medical University, Shinjyuku-ku, Tokyo, Japan
4.1 Introduction of Autoimmune Orchitis in Experimental Animals
Testicular autoimmunity in experimental animals can be divided into “spontaneous” and “experimental” autoimmune orchitis (EAO).
Spontaneous autoimmune orchitis was reported in the dog (Fritz et al. 1976; Davidson et al. 2015), the mink (Tung et al. 1981, 1984; Pelletier et al. 2009), the rat (Furbeth et al. 1989), and the mouse (Robinson et al. 1994). The pathology of the spontaneous orchitis involves lymphocytic infiltration, spermatogenic disturbance, and deposits of immune complexes in the basal lamina of seminiferous tubules with appearance of serum anti-TGC autoantibodies; however, its pathophysiology remains unclear. In the mink, there are two pathological patterns: one has massive granulomatous inflammatory lesion, and another has extensive TGC loss with little inflammation (Lustig et al. 2014). It is noted in the mink that there is an association of spontaneous autoimmune orchitis with abnormal hypothalamic-pituitary-testicular axis since correction of the hormonal defect prevented the orchitis (Tung et al. 1984). Pelletier et al. (2009) identified aberrations leading to spontaneous autoimmune orchitis, in which a malfunction of the clearance mechanisms for apoptotic cell debris arising from imbalance in phagocyte receptors or cytokines acting on Sertoli cells was observed with increased serum levels of TNF-alpha and IL-6 in the mink. Western blot analyses revealed that serum from minks with the orchitis reacted specifically to 23 and 50 kDa proteins of TGC. With the advance of the spontaneous autoimmune orchitis, expression of connexin 43, a gap junction protein, decreased (Pelletier et al. 2011).
On the other hand, EAO are experimentally induced by various protocols for immunization with testicular autoantigens or for alteration of the immune system without injecting testicular autoantigens. The first model of EAO was developed by Freund et al. (1953). The EAO lesions contained immunopathological evidence of granulomatous or non-granulomatous orchitis, testicular immune complexes, and epididymal granuloma of noninfectious origin. Similar changes have been often found in testicular biopsies from infertile men. In general, EAO is CD4+ T cell dependent and characterized by lymphocytic inflammation with damage to the seminiferous epithelium, that is, the shedding and apoptosis of TGC. The complete histopathological lesion is produced by both cellular and humoral immune responses. EAO has been investigated in guinea pigs, rats, mice, and other animals for more than 60 years, and, at present, the mouse has the distinct advantage of the availability of a wide range of immunological markers and a well-defined genetic background.
Chapters 4–7 review research on various EAO models. Its aim is to review and attempt to understand the regulatory mechanisms that normally prevent EAO from occurring, the events that overcome these control mechanisms, and, once initiated, the mechanisms that amplify the disease process (Fig. 4.1).


Fig. 4.1
Three main factors for induction or suppression of experimental autoimmune orchitis (EAO)
4.2 Various EAO Models by Immunization with Testicular Antigens Alone
4.2.1 EAO Induced by Immunizations with Syngeneic TGC Alone
4.2.1.1 Induction of Active EAO
Active EAO is produced in both C3H/He and A/J mice with as high as 100% incidence by two subcutaneous injections of 1 × 107 viable syngeneic TGC on days 0 and 14 without resorting to any adjuvants or other artificial immuno-potentiation (Fig. 4.2a) (Itoh et al. 1991a). Prepared TGC contained all kinds of spermatogenic cells including spermatogonia, spermatocytes, spermatids, and spermatozoa. This EAO develops fully within 40 days through three different phases: a preclinical phase (until day 20), the disease onset phase (from day 20 to day 25), and the disease developing phase (from day 25 to day 40) (Itoh et al. 1995a; Naito and Itoh 2008; Naito et al. 2012a, b). The autoimmune nature of this model may be evidenced by a steady occurrence of both antibody and DTH responses against syngeneic TGC (Hirai et al. 2013a; Qu et al. 2014). In particular, the level of DTH response to TGC protein was highly relevant to the pathology of EAO development. As this EAO model can be induced without the immune responses against adjuvants, it is simply autoimmune in nature. It is also noted that immunization with syngeneic epididymal spermatozoa failed to induce EAO (Fig. 4.2b).


Fig. 4.2
Comparison of autoimmune inflammation between TGC- and epididymal spermatozoa-immunized mice. a, mice immunized with TGC. b, mice immunized with epididymal spermatozoa. Dotted area indicates the presence of the lymphocytic inflammation. D, ductuli efferentes. E, epididymis. T, testis. V, vas deferens
4.2.1.2 Histopathology of Testicular Inflammation
In this EAO model, lymphocytes first appear around the tubuli recti, surround them, and then spread to the peripheral interstitium, resulting in induction of spermatogenic disturbance (Naito and Itoh 2008; Naito et al. 2009) (Fig. 4.3). Therefore, the pathologic lesions preferentially involve the tubuli recti where the BTB may be incomplete. A number of F4/80+ and class II MHC antigen+ cells were located in and around the tubuli recti of the TGC-immunized mice. This model is very unique in that epididymitis and vasitis are completely lacked (Itoh et al. 1991a, b) (Fig. 4.2). Therefore, the immune responses involved in this model may be mainly directed to testis-specific antigens on TGC but not to specific antigens on epididymal spermatozoa bearing late developing male antigens. The antigenic determinants of TGC, which diminish or disappear as the spermatogenic cells differentiate to mature sperm, may be chiefly responsible for the induction of this EAO model. Unexpectedly, subcutaneous injections of viable epididymal spermatozoa did not evoke any autoimmune inflammation in the epididymides (Fig. 4.2). Therefore, the testis rather than the epididymis may easily become an unprivileged organ as to autoimmunity in this experimental model. It may be that testicular but not epididymal haploid cells, spermatids but not spermatozoa, have critical autoantigens for this EAO.


Fig. 4.3
Histopathological stages of EAO. D, ductuli efferentes. R, rete testis. S, seminiferous tubules. T, tubuli recti. Dark–colored area indicates the presence of the lymphocytic inflammation
Both cellular (CD4+ T cells and CD8+ T cells) and humoral (B cells and plasma cells) immunity participate in immunohistochemical manifestation of the EAO lesion. T cells in the testes were as numerous as cytoplasmic Ig-bearing cells (approximately 30% of all inflammatory infiltrating cells). The T cells and cytoplasmic Ig-bearing cells were diffusely distributed through the interstitium, while B220+ cells formed focal collections and were predominantly located in the subcapsular lesion. Major phenotype of T cells in the lesion was CD4+ (approximately 85%) with the remainder (approximately 15%) being CD8+. Deposits of immunoglobulins and complements were identified on the basement membrane of the seminiferous tubular wall, interstitium between the seminiferous tubules, blood capillary endothelium, and degenerated TGC. Inflammatory cells (including macrophages, B cells, and, probably, some activated T cells) in the lesion were class II MHC antigens+ (Itoh et al. 1991b). However, Leydig cells, Sertoli cells, and TGC did not exhibit class II MHC antigens at all. In addition, after the active inflammation stage of this model, the seminiferous epithelium is damaged irreversibly, resembling the histopathology of human male idiopathic spermatogenic disturbance (Naito et al. 2012a).
During EAO, lymphangiogenesis occurred along the undersurface of the tunica albuginea but not into the interstitium proper between the seminiferous tubules (Hirai et al. 2012, 2013b). It was noted that some F4/80+ macrophages expressed lymphatic vessel endothelial hyaluronan receptor (LYVE)-1, a lymphatic endothelial cell marker, at the undersurface of the tunica albuginea and also in the testicular interstitium proper between the seminiferous tubules, indicating that activated macrophages play an important role in the process of testicular lymphangiogenesis. All LYVE-1+ cells between the seminiferous tubules are positive for F4/80 and negative for CD31, an endothelial marker. There is no LYVE-1+ cell in normal testicular interstitium proper between the seminiferous tubules (Hirai et al. 2010, 2012). These data suggested that LYVE-1+ cells in the testicular interstitium proper in testicular inflammation are mature macrophages but not endothelia of lymphatic capillary. LYVE-1+ cells in EAO were frequently detected around blood capillaries but did not form capillary structure in the testicular interstitium proper. However, the interstitium under the tunica albuginea, the lymphatic capillary structure, was identified, and some LYVE-1+ macrophages were incorporated into the wall of lymphatic capillaries. This suggests that some F4/80+ mature macrophages expressing LYVE-1 migrate and are incorporated into the lymphatic capillaries for lymphangiogenesis during inflammatory testis. Real-time RT-PCR analysis revealed that the expression of vascular endothelial growth factor (VEGF)-A, VEGF-D, and TNF-alpha were significantly increased but that of VEGF-C remained unchanged in the inflammatory testes. The interstitial area in EAO was expanded on active inflammatory condition and then reduced with resolution of lymphocytic inflammation under post-inflammatory condition (Naito et al. 2012a). In EAO, lymphatic capillaries extend along the undersurface of the tunica albuginea, indicating that increased lymphatic capillaries preferentially surround inflammatory lesions and play important roles in draining both the increased interstitial fluid including the infiltrating lymphocytes effectively (Hirai et al. 2012, 2013b).
4.2.1.3 Histopathology of the Spermatogenic Disturbance
A significant spermatogenic disturbance was consistently induced after the appearance of inflammatory cell responses around the tubuli recti (Itoh et al. 1995a, b). The histopathology of the seminiferous tubules in the early phase ranged from partial degeneration and depletion of all kinds of TGC to complete loss of spermatocytes, spermatids, and spermatozoa, with some remaining spermatogonia, while both Sertoli cells and the basal lamina of the tubules appeared relatively intact. The disturbance of spermatogenesis which had started near the tubuli recti region spread to the peripheral area in spite of the fact that the inflammatory cell infiltration was still limited to the tubuli recti region. Thus, the damage to seminiferous epithelium was not dependent on a direct contact of the inflammatory cells with the epithelium. In the late phase, loss of contact between TGC, appearance of some round-shaped degenerating ones, depletion of some Sertoli cells with detachment of the cells from the basal membrane, distortion and dissociation of the tubular wall layers, appearance of malformed spermatids with signet ring nuclei, depletion of immature TGC with remaining elongated spermatids, or complete loss of the seminiferous epithelium were observed in addition to the early histopathological features. Some Sertoli cells and a few spermatogonia were sometimes remained even when the tubules were infiltrated by inflammatory cells, showing that they, especially Sertoli cells, are relatively resistant to this autoimmunity. In the most severely damaged tubules, the whole tubular architecture was destroyed by the invading inflammatory cells and replaced by granulomatous tissue. Considering that this EAO model is induced by spermatid-specific autoimmunity, the histological feature of the seminiferous tubules in the lesions apparently shows depletion of elements of the seminiferous epithelium in an antigen-nonspecific fashion. These features support the theory that a disturbance of TGC development rather than a specifically immunological depletion of TGC may be critical for producing such lesions (Fig. 4.4).


Fig. 4.4
Modes of lymphocytic inflammation and the following spermatogenic disturbance during EAO
Previously, the pathogenesis for TGC depletion in EAO-affected seminiferous tubules was often attributed to an influx of specific autoantibodies into the germ cell ducts with a leaky BTB. However, this theory is not suitable for the antigen-nonspecific TGC depletion in EAO. At least three causes can be considered for the disturbance of spermatogenesis. The first is that the presence of the testicular inflammation induces an impairment of the local circulation of blood and lymph in the testis, leading to an inhibition of TGC development. The circulatory impairment in the testis is evidenced by the presence of congestion and widened lymphatic space in the lesion. The circulatory impairment may also inhibit transport of TGC by peritubular myoid cell contraction. The local congestion may also elevate intra-testicular temperature, with the resultant physical damage to the seminiferous epithelium. The second is that intense inflammation surrounding the tubuli recti may cause dysfunction of the valve of the modified Sertoli cells at tubuli recti for regulation of intra-seminiferous tubular pressure. The valve dysfunction may evoke increase in the intratubular pressure, resulting in damage to the seminiferous epithelium. The third is that the inflammatory cells in EAO lesion locally secrete various cytokines (such as IL-1, IL-2, IFN-gamma, and TNF-alpha), which may be harmful to the TGC development (Fig. 4.4).
It has been demonstrated that Fas/Fas ligand- and Bax/Bcl-2-mediated apoptoses are two of the main causes of TGC death under various conditions, including cryptorchidism, vasectomized testis, and testicular torsion (Koji 2001; Koji et al. 2001; Kuerban et al. 2012). In TGC-induced EAO, some TGC still exist in the seminiferous tubules when lymphocytic infiltration has finally resolved (Naito et al. 2012a, b). It was also found that both the proliferation and death of TGC occurred simultaneously in the seminiferous tubules during the post-active inflammation stage of TGC-induced EAO. To get more detailed pathogenic information on TGC death during EAO, involvements of Fas/Fas ligand and Bax/Bcl-2 systems in TGC death following immunization with TGC were studied (Kuerban et al. 2012). Many TUNEL-stained TGC were present in the seminiferous tubules during the active inflammation stage, and these cells were persistently observed in the seminiferous epithelium until the post-active inflammation stage. Intra-testicular mRNA expression levels of both Fas and Bax were increased during the active inflammation stage and were dramatically decreased during the post-active inflammation stage. In contrast, the intra-testicular mRNA expression levels of both Fas ligand and Bcl-2 did not show significant changes during the active inflammation stage. Immunohistochemically, some Fas- and Bax-positive germ cells were detected during the active inflammation stage, but these were hardly found during the post-active inflammation stage. In contrast, some Fas ligand- and Bcl-2-positive TGC were immunohistochemically found during the active inflammation stage, and many of these were also observed during the post-active inflammation stage. These results indicate that TGC death during TGC-induced EAO is mediated by the Fas/Fas ligand and Bax/Bcl-2 systems during the active inflammation stage but not the post-active inflammation stage. Therefore, TGC death during the post-active inflammation stage should occur through mechanisms other than the Fas/Fas ligand or Bax/Bcl-2 system in TGC-induced EAO. The intra-testicular mRNA expression levels of caspase 3 and caspase 8 increased during the active inflammation stage; however, the expression level of caspase 9 decreased during this stage. This implies that TGC apoptosis during the active inflammation stage is primarily mediated by the Fas/Fas ligand system rather than the Bax/Bcl-2 system. During the post-active inflammation stage, the intra-testicular mRNA expression levels of caspase 3 and caspase 8 did not change significantly, whereas the expression level of caspase 9 decreased. Considering that TUNEL-positive TGC were detected at higher levels than those of normal mice during the post-active inflammation stage, most TGC deaths are probably non-apoptotic during the post-active inflammation stage. In general, cells that show increased resistance to stress require higher stimulus levels to induce cell death. The ability to evade apoptosis is induced by a range of different alterations, inducing physiological changes such as the activation/upregulation of mitogenic signaling pathways, inactivation/downregulation of certain apoptotic molecules, and upregulation of a number of anti-apoptotic genes. In normal condition, Fas- and/or Bax-positive TGC and Fas- and Bax-negative TGC exist simultaneously; however, Fas- and/or Bax-positive TGC were increased during the active inflammation stage, but Fas- and Bax-negative TGC remained alive during the post-active inflammation stage. There is a possibility that the upregulation of Fas ligand and Bcl-2 during the post-active inflammation stage is a reaction against the inactivation of Fas- and the Bax-mediated TGC death, respectively (Kuerban et al. 2012).
4.2.1.4 Passive EAO by Adoptive Transfer of Lymphocytes
For analysis of immunological events at the beginning and the course of this autoimmune reaction, the adoptive transfer system offers an important approach. In a simple protocol for adoptive transfer of EAO, cell donors were mice that received subcutaneous injections twice with TGC alone (Itoh et al. 1991c). Spleen cells from the donor mice were stimulated in vitro with TGC, propagated in IL-2-containing medium, and then injected intraperitoneally or intravenously to naive recipient mice. This procedure induced severe orchitis and spermatogenic disturbance in the recipients at high incidence, with often involvement of inflammation in the epididymis and the vas deferens. Elimination of all T cells or CD4+ T cells before the transfer produced no histopathological signs in the recipients, whereas that of the CD8+ T cells or B cells had no inhibitory effects on the disease transfer, indicating that the effector cells are CD4+ T cells (Itoh et al. 1991c). Therefore, the CD4+ T cells are critical cells for EAO induction and the CD8+ cells (approximately 15% of infiltrating leukocytes in EAO lesion), B cells, and plasma cells (approximately 50% of infiltrating leukocytes) may be involved in further development of EAO lesion (Itoh et al. 1991b). It is known that CD4+ T cells recognize peptides in association with class II MHC molecules, although MHC class II-positive cells in normal mouse testis are quite sparse.
In the experimental system using mice immunized with TGC alone, it is also noted that mode of inflammatory cell infiltration in adoptively transferred EAO to recipients (passive EAO) differs from that in EAO induced by subcutaneous injections with TGC (active EAO). Although the orchitis affecting the tubuli recti and seminiferous tubules at various sites was common between passive and active EAO, epididymitis and vasitis were accompanied only in the passive EAO (Itoh et al. 1991a, b, c). The reason for producing the different histopathology is unknown. Differences in migration property of lymphocytes to the target organ may exist between the transferred lymphocytes stimulated in vitro in passive EAO and lymphocytes stimulated in vivo in active EAO. At present, the precise mechanism of migration of the transferred TGC-specific lymphocytes into the male reproductive tissues is still unanswerable. The donor lymphocytes introduced into naive recipients were able to home to the testis and induce EAO without depending on artificial perturbation of the BTB. This indicates that a part of TGC autoantigens are not immunologically sequestered by the BTB and accessible by exogenously administered TGC-specific CD4+ T cells in normal recipient mice.
Subsequently, a CD4+ T cell line was derived from mice immunized with TGC alone by subsequent repeated selection of the lymphocytes in vitro with soluble testicular antigens (Itoh et al. 1992a). Intraperitoneal or intravenous inoculation of as few as 1 × 105 lined cells that had been stimulated in vitro with testicular antigens before inoculation was capable of transferring EAO to naive recipients. The transferred lesion was characterized by infiltration of inflammatory cells into the epididymis and rete testis (epididymo-orchitis) and the following widespread spermatogenic disturbance in the testis. Therefore, this passive EAO by CD4+T cell line is quite different from active EAO (orchitis without epididymitis) in regard to mode of inflammatory cell infiltration. In the T cell line-induced EAO, lymphocytic infiltration consistently started in the epididymis before spreading to the rete testis (Fig. 4.5). In vitro characterization of the biological activity of the lined cells revealed that the cells had no cytolytic or cytotoxic activity against TGC, but the culture supernatant had macrophage migration inhibitory activity involved in the DTH response (Itoh et al. 1993). Therefore, the DTH responsiveness transferred by the T cell line was found to correlate with their EAO-inducing capacity. It is also important to stress that the transferred T cell line must be stimulated with testicular antigens before the cell transfer for the successful induction of EAO. The lined T cells cultured in the absence of stimulation with testicular antigen revert to the resting state and lose their EAO-inducing capacity. Although it is supposed that the activated cells may home in on the testis and epididymis more efficiently than the resting cells, it is not yet clear what differences exist at the molecular level between the activated and resting lined cells in this EAO transfer model. In the relevant experiments, when the lined orchitogenic CD4+ T cells were seeded onto cultured monolayers of syngeneic Sertoli cells and dermal fibroblasts, they immediately induced detachment of the both monolayers in an antigen-nonspecific manner (Itoh et al. 1993). The disease transfer was antigen specific, because no inflammatory lesion was found in any organs other than epididymis and testis by intraperitoneal transfer of the T cell line. Therefore, activated T cell line might specifically home in on the epididymal and testicular vasculatures, migrate into their interstitium, and then break the integrity of the blood-epididymal barrier and the BTB, in an antigen-nonspecific fashion through the secretion of some cytokines or direct cell contact. Furthermore, with loss of the blood-epididymal barrier and the BTB, more germ cell autoantigens might leak from the epididymal ducts and the seminiferous tubules, and a continuous source of the autoantigens for immune stimulation might be provided. Indeed, the culture supernatant of the T cell line induced nonspecific local inflammation when injected into subdermal tissue of normal syngeneic mice. Therefore, the lined T cells were shown to devastate a tissue integrity and cause attraction and activation of inflammatory cells of the recipient origin in a local environment. The injection of culture supernatant from the lined T cells with irradiated spleen cells caused more intense subdermal inflammation compared to that of the culture supernatant alone. These results suggest that the transferred lined CD4+ T cells will specifically home in to the testis and epididymis of recipients, but the following devastation of seminiferous tubules and epididymal ducts might be nonspecifically produced by coexistence of the donor CD4+ T cells and other inflammatory cells of recipient origin in the lesion.


Fig. 4.5
Autoimmune orchitis, epididymitis, and vasitis by TGC-specific lymphocytes
4.2.1.5 Humoral Immunity in EAO
Serum autoantibody titers against TGC and epididymal spermatozoa gradually increased with the development of EAO (Hirai et al. 2013a). At the initial stage of EAO, serum autoantibodies reacted with only a few antigens of TGC and epididymal spermatozoa, while in the later stage, the reactions with various antigens of TGC and epididymal spermatozoa occurred (Itoh et al. 1994; Qu et al. 2010; Musha et al. 2013). However, the autoantibodies were specific to the testis and epididymis but not to other organs (seminal vesicle, brain, heart, lung, thymus, salivary gland, small intestine, liver, pancreas, kidney, muscle, and ovary) in enzyme-linked immunosorbent assay (Itoh et al. 1989; Hirai et al. 2013a). In active EAO of which induction is CD4+ T cell-dependent, B cell lineage (B cells and plasma cells) was found to be approximately 50% of all infiltrating cells in the testis lesion (Itoh et al. 1991b). Although the disease was transferrable to naive recipients only by CD4+ T cells from TGC-immunized donor mice, a significant infiltration of B cells and plasma cells in EAO lesion suggests that the local humoral immunity also might affect the integrity of seminiferous epithelium and the supporting tissue in the testis. Many plasma cells preferentially infiltrated around the tubuli recti already from the early stage of EAO (Naito et al. 2009, 2012b). A number of these cytoplasmic Ig-bearing cells contained IgG or IgA. It is noted that there was a good correlation between the serum level of anti-TGC antibodies of IgG class and the intensity of IgG deposition in the testis lesion, but serum IgA autoantibodies were not detected in spite of evidence of IgA deposition on degenerated cells inside the epididymal ducts. There were no cytoplasmic IgA-bearing cells in any portion of the epididymal interstitium in TGC-induced active EAO. It appears most likely that the IgA deposits on degenerated cells inside the epididymal ducts were derived from TGC reacted with intra-seminiferous tubular-specific IgA antibodies secreted by a number of IgA-producing cells residing in the testis lesion. There is another possibility that degenerated IgA-producing plasma cells themselves which had infiltrated into the seminiferous tubules were finally flowed to the epididymal ducts. The circulating antibodies of IgG class reacted strongly with the acrosomal portion of round and elongating spermatids but only weakly with that of mature spermatozoa (Itoh et al. 1991b, 1994). Although the IgM antibodies reactive with the acrosomal area of immature TGC were also identified, the same class of antibodies reactive with the acrosome of mature spermatozoa was undetectable (Itoh et al. 1991b). It seems therefore that this testicular autoimmunity may be primarily directed to immature acrosomal proteins of developing haploid cells which diminish as the spermatids differentiate to mature sperm, with the resultant orchitis but not epididymo-vasitis (Fig. 4.2). Anti-TGC antisera primarily reacted with the acrosomal and proacrosomal portion of spermatids of mice as early as 3 weeks (Soramoto et al. 1993; Itoh et al. 1994). As shown in enzyme-linked immunosorbent assay, immunohistochemical stainings obtained by reaction of the immune sera with liver, kidney, and ovary sections were also negative. Therefore, the results indicate that the circulating antibodies are quite organ specific (Itoh et al. 1994; Hirai et al. 2013a). However, it cannot be excluded that circulating autoantibodies against spermatogonia, spermatocytes, Sertoli cells, the basal lamina of the seminiferous tubules, and the Leydig cells are also produced in TGC-immunized mice but deposited in the testicular tissue of the host, resulting in absorption of the autoantibodies against testicular cells other than the spermatids and spermatozoa from the blood circulation, because the testicular tissues outside the BTB formed by inter-Sertoli cell junction apparently has free access to the products delivered from the blood circulatory system. In fact, the immune deposits had been detected on outside the BTB in the lesion of this EAO (Itoh et al. 1991b; Naito et al. 2012a, b). In this way the autoantibodies would easily react with the testicular tissue of the host and disappear from the serum samples. To test this possibility, an immunohistochemical examination was performed by using the immune sera from mice that were castrated before receiving the first TGC injection 7 days later. However, even in this case, the serum autoantibodies were against round, oval, and elongated-shaped spermatids but never on less mature TGC resting on the basal lamina, Sertoli cells, basal lamina, or testicular interstitial cells. Therefore, intra-testicular plasma cells may secrete autoantibodies against spermatids and spermatozoa. Furthermore, the preferential involvement of the tubuli recti might be due to that infiltrating class II MHC-positive B cells around the tubuli recti function as antigen-presenting cells and attract more lymphocytes (Naito et al. 2009). Furthermore, antibodies secreted from plasma cells in situ may cause further damage to basal lamina of the tubuli recti and the seminiferous tubules.
4.2.1.6 Developmental Autoimmunogenicity of TGC
Trials to induce EAO by immunization with TGC obtained from various aged mice revealed that TGC from donor mice younger than 3 weeks of age did not exhibit the capability to induce the disease; however, TGC from donor mice older than 4 weeks of age showed the EAO-inducing capability (Soramoto et al. 1993). Developmental studies using various aged mice demonstrated that TGC from 6- rather than 5-week-old mice had more strong capability of inducing EAO (orchitogenicity) (Fig. 4.6). Both DTH-inducing and anti-TGC antibody-eliciting capabilities were observed in mice immunized with TGC from donor mice older than 4 weeks of age. Therefore, this implies that the testis becomes vulnerable to localized autoimmunity as early as 4 weeks of age when spermatids first start to differentiate in the seminiferous tubules. To assess the cytokine release from specific lymphocytes stimulated with syngeneic TGC derived from mice at various ages, levels of IL-2 and IFN-gamma in the supernatants obtained from co-culture of lymphocytes with each TGC were determined. The spleen cells did not secrete detectable levels of IL-2 and IFN-gamma in response to TGC from 3-week-old mice but did upon stimulation with TGC from 4 weeks or older (Soramoto et al. 1993).


Fig. 4.6
Ontogeny of TGC autoimmunogenicity for EAO induction. Immunization with TGC obtained from donor mice older than 4 weeks of age has a sensitizing capability to induce EAO in the recipients
In biochemical studies for detection of autoantigens of TGC, the fragility of TGC plasma membrane following exposure to chemical treatment must be kept in mind. By using SDS-polyacrylamide gel electrophoresis and immunoblotting in reaction of normal sera with normal murine testicular homogenates, two immunoreactive bands corresponding to approximately 45 and 100 kDa were detected (Qu et al. 2010; Musha et al. 2013). These two natural autoantibodies were more definitely detected in sera of TGC-induced EAO. Furthermore, the immune serum samples also reacted with three additional autoantigens of approximately 25, 40, and 250 kDa.
Later, Terayama et al. (2016) tried to identify TGC-specific autoantigens relevant to EAO. In that study, 11 TGC-specific autoantigens including (1) tubulin, beta 2cl; (2) ATPase, H+ transporting, lysosomal V1 subunit A; (3) pyruvate dehydrogenase lipoamide beta; (4) heat shock protein 1-like; (5) fructose bisphosphatase 1; (6) leucine-rich repeat containing 34; (7) aspartyl aminopeptidase isoform b; (8) glyceraldehyde-3-phosphate dehydrogenase, spermatogenic; (9) pyruvate dehydrogenase E1 alpha 2; (10) DAZ-associated protein 1; and (11) unnamed protein product (fumarate hydratase-related protein) were specifically reacted with serum IgG from TGC-immunized mice by TGC liquid chromatography-tandem mass spectrometry analysis, followed by two-dimensional gel electrophoresis. Real-time RT-PCR analysis by the use of various organs such as the testis, epididymis, submandibular gland, spleen, heart, kidney, skeletal muscle, small intestine, liver, brain, lung, and pancreas showed that the mRNA expressions of 9 of identified 11 TGC-specific autoantigens except for (2) ATPase, H+ transporting, lysosomal V1 subunit A, and (7) aspartyl aminopeptidase isoform b were extremely higher in the testis compared to in other organs. Moreover, mRNA expressions of (1) tubulin, beta 2 cl, (2) ATPase, H+ transporting, lysosomal V1 subunit A, (4) heat shock protein 1-like, (5) fructose bisphosphatase 1, (6) leucine-rich repeat containing 34, (8) glyceraldehyde-3-phosphate dehydrogenase, spermatogenic, and (10) DAZ-associated protein 1 in normal testes of 8-week-old mice were significantly higher than those of 2-week-old mice. Exceptionally, only mRNA expression of (7) aspartyl aminopeptidase isoform b in normal testes of 8-week-old mice appeared lower than those of 2-week-old mice. It is also noted that mRNA expressions of 10 of identified 11 autoantigens except for (7) aspartyl aminopeptidase isoform b were more in TGC than in epididymal spermatozoa. Moreover, the identified each protein was produced by HEK293T cells that were transfected plasma vector with each candidate gene and then reacted with serum antibodies from EAO-induced mice by the Western blot analysis. The results showed that (2) ATPase, H+ transporting, lysosomal V1 subunit A, (4) heat shock protein 1-like, (5) fructose bisphosphatase 1, (9) pyruvate dehydrogenase E1 alpha 2, and (10) DAZ-associated protein 1 specifically reacted with the immune sera, but not control ones. Therefore, three proteins composed of (4) heat shock protein 1-like, (5) fructose bisphosphatase 1, and (10) DAZ-associated protein 1 are important target autoantigens for EAO. In particular, in adult human testes, (4) Heat shock protein 1-like and (10) DAZ-associated protein 1 but not (5) fructose bisphosphatase 1 are specifically detected in round and elongated spermatids, indicating that these two proteins are critical for EAO induction (Uhlen et al. 2010, 2015).
By subtracted cDNA library screening, Nagahori et al. (2017) also tried identification of TGC-specific autoantigens related to EAO. In that study, 24 TGC-specific autoantigens including (1) 1700034O15, (2) diazepam binding inhibitor, (3) 1700093K21, (4) ADP-ribosylation factor-like 4A, (5) 2210012G02, (6) N-acetyltransferase 9, (7) 1700054F22, (8) interferon-stimulated protein, (9) zinc and ring finger 4, (10) Y box protein 2, (11) Y box protein 1, (12) abhydrolase domain containing 6, (13) syntaxin 18, (14) germ cell-specific gene 1, (15) peroxisomal biogenesis factor 3, (16) ubiquinol cytochrome c reductase core protein 2, (17) eukaryotic translation initiation factor 3, subunit E, (18) atlastin GTPase 3, (19) Zona pellucida 3 receptor, (20) LIM domain containing preferred translocation partner in lipoma, (21) G-protein-coupled receptor kinase-interactor 1, (22) nicotinamide nucleotide transhydrogenase, (23) heat shock protein 4-like, and (24) ankyrin repeat domain 36 were specifically reacted with serum IgM from TGC-immunized mice by screening cDNA library from mouse TGC. Real-time RT-PCR analysis by the use of various organs such as the testis, epididymis, submandibular gland, spleen, heart, kidney, skeletal muscle, small intestine, liver, brain, lung, and pancreas showed that the mRNA expressions of 19 of identified 24 TGC-specific autoantigens except for (2), (8), (16), (20), and (22) were extremely higher in the testis compared to other organs. Moreover, mRNA expressions of (1), (3), (6), (9), (10), (13), (14), (17), (18), (20), (21), and (23) in normal testes of 8-week-old mice were significantly higher than those of 2-week-old mice. It is also noted that mRNA expressions of 23 of identified 24 autoantigens except for (2) were more in TGC than in epididymal spermatozoa. Therefore, it is indicated that TGC-specific autoantigens such as (1), (3), (6), (9), (10), (13), (14), (17), (18), (21), and (23) are relevant target autoantigens for EAO. In adult human testis, (3), (14), and (21) are detected on various-staged TGC, and (6), (13), and (17) are detected on not only various-staged TGC abut also Leydig cells (Uhlen et al. 2010, 2015). In contrast, both (10) Y box protein 2 and (23) heat shock protein 4-like are detected definitely on round spermatids and faintly on other immature TGC, implying that these two antigens are critical for EAO induction.
4.2.1.7 Cytokines and Hormones in EAO
Both IFN-gamma and TNF-alpha were detected from cultured spleen cells and lymph node cells in EAO-induced mice in vitro (Tokunaga et al. 2007). A significant stimulation of IL-5 and IL-6 (Th2 cytokines) production by sensitized spleen cells was detectable when the TGC from 3-week or older mice were used as stimulants (Soramoto et al. 1993). IL-2 and IFN-gamma (Th1 cytokines) production was detected by immunization with TGC from 4-week or older mice. From this evidence, it is suggested that both Th1 and Th2 cells are involved in the immunization process of this EAO model. No IL-4 production by TGC-immunized spleen cells was detectable in C3H/He mice. The one reason for this result is that higher levels of IFN-gamma production may inhibit IL-4 production. For another reason, it is thought that levels of lL-4 production may be innately low in C3H/He compared with BALB/c mice. Actually, it has been shown that soon after Leishmania major infection, lymphocytes from a resistant strain C3H/He mice secrete higher levels of IFN-gamma and no IL-4, whereas lymphocytes from a susceptible strain BALB/c mice secrete IL-4 but little IFN-gamma (Scott 1991; Coffman et al. 1991). Splenic production of both Th1- and Th2-related cytokines increased after the second but not the first immunization with TGC, indicating that secondary immune responses are critical for the EAO induction (Tokunaga et al. 2007). Furthermore, the production of Th1-related cytokines became predominant at the clinical stage of EAO. In particular, IFN-gamma, a Th1-producing cytokine, significantly increased after the second immunization with TGC, decreased temporally at the preclinical phase of the EAO, but increased again at the clinical phase. Similar kinetics of cytokine secretion were observed for both IL-3 and granulocyte-macrophage colony-stimulating factor, which are produced by both Th1 and Th2 cells. The secretion of IL-2, another Th1-producing cytokines, gradually increased up to the clinical phase. IL-1, a DTH-related cytokine derived from macrophages, was also continuously produced after the second immunization. In contrast, IL-4, a Th2-producing cytokine, was not produced by the cultured spleen cells. Furthermore, the levels of other Th2-producing cytokines such as IL-5 and IL-10 were temporally increased after the second immunization with TGC but decreased later. However, IL-6, another Th2-producing cytokine, was continuously produced after the second immunization (Tokunaga et al. 2007).
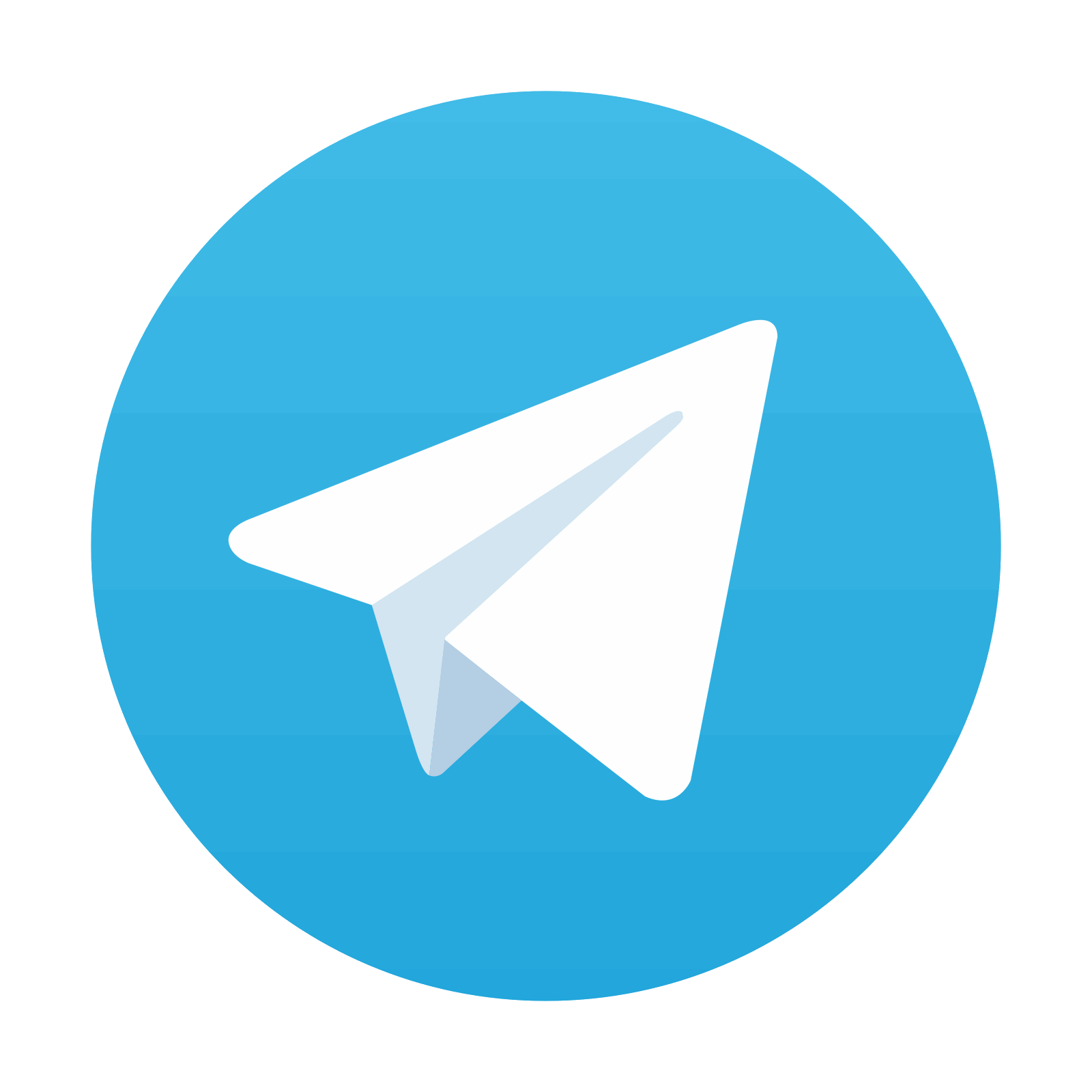
Stay updated, free articles. Join our Telegram channel

Full access? Get Clinical Tree
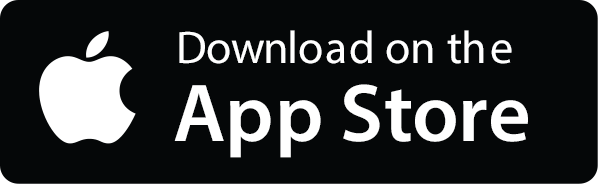
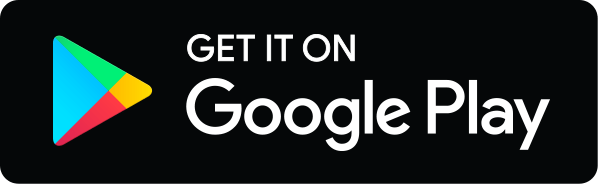
