Fig. 8.1
(a) First generation ablation system. Generator and 8 F ablation catheter. (b) Second generation generator with a touch screen
The second generation generator is slimmer and has updated futures (Fig. 8.1b). It can be operated via a touch screen and features allow simultaneous activation of all electrodes. The new algorithm aims at a target temperature of 70 °C and burning time of 60 s, thus substantially shortening procedure time. A new ablation catheter that is 6F is in preparation.
The first generation ablation system was used in the early animal studies and EnligHTN I study. The second generation system is being used in EnligHTN III and EnligHTN IV studies.
Procedure
The first generation ablation system is utilizing an 8F guide catheter which is advanced and engages each renal artery sequentially. Renal angiography is performed to confirm renal artery anatomy. An appropriate basket size is chosen based on the renal artery diameter (small basket 4.0–5.5 mm diameter/large basket 5.5–8.0 mm diameter). The ablation procedure is being standardized as follows: The ablation catheter is introduced to the renal artery and advanced so the tip is situated before the first major renal artery branch. The basket is then expanded, and after assuring good contact with the arterial wall, each electrode is activated sequentially for 90 s (the second generation system will be using simultaneous activation of all four electrodes, aiming at 70 °C and burning time of 60 s). The basket is then collapsed, pulled back, turned and re-expanded to repeat the ablation procedure. A total of 4–8 discrete ablation lesions are delivered. The ablation catheter is then withdrawn and a renal angiogram is repeated to assess safety. The same procedure is repeated in the contra-lateral renal artery. Heparin is given during the procedure (3,000–7,000 U) as per protocol, to avoid clot formation. Visceral pain occurring during the ablation procedure is managed with intravenous analgesics, sedatives and/or narcotics.
Animal Data
The early animal experiments were carried out in miniature swine (Fig. 8.2) and the objective was primarily the feasibility and safety of the procedure. The early results showed that it was feasible to place radiofrequency ablation lesions transvascularly through the arterial wall and ablate the fibers coursing through the adventitia of the renal artery. The lesions were transmural and reached up to 3 mm from the endothelium of the artery. It was also shown that the procedure was safe, there was no aneurysm formation or perforation. One month after the procedure it was shown that the lesions would heal with no residual findings. Acutely however, it was found that a clot could be formed at the ablation site, but it could be prevented using heparin and aspirin. The ablation catheter allowed placement of the electrodes in a pre-determined manner and geometric distribution that would ensure circumferential interruption of the sympathetic fibers (Fig. 8.3).



Fig. 8.2
Swine animal model for renal denervation

Fig. 8.3
The ablation procedure, acute and chronic ablation lesions of the dissected renal artery
The first animal study was designed to further assess feasibility and safety, but also to evaluate the effects of renal denervation on renal hemodynamics [36]. In this study we investigated the acute and chronic effects of catheter-based renal nerve ablation on renal hemodynamics assessed by average peak velocity (APV), renal blood flow (RBF), renal flow reserve (RFR) and resistive index (RI). The aims of this study were based on the premise that sympathetic overdrive is accompanied by impaired RBF and renal denervation would result in improvement in renal blood flow. Experiments were performed in nine farm swine. APV was measured by a 0.014-in. Doppler flow wire placed in the stem of the renal artery under baseline and hyperemic conditions, induced by intrarenal dopamine (50 μg/kg). RFR was calculated as the ratio of hyperemic to basal peak velocity, and RI was estimated as peak systolic velocity – end-diastolic velocity)/peak systolic velocity. RSD was achieved via the lumen of the main renal artery with a specifically designed catheter connected to a radiofrequency generator according to prespecified algorithm. APV and RBF increased acutely post ablation in all animals, compared to APV and RBF before ablation (61.44 ± 32.6 versus 20.44 ± 6.38 cm/s, p < 0.001; and 407.4 ± 335.1 versus 161.1 ± 76.6 ml/min, p = 0.003; respectively), whereas RFR and RI were reduced (1.51 ± 0.59 versus 2.85 ± 1.33, p < 0.001; and 0.67 ± 0.07 versus 0.74 ± 0.07, p = 0.005; respectively). One month post ablation APV and RBF compared to APV and RBF before ablation remained significantly higher whereas RFR and RI remained lower as compared to baseline. From these experiments we concluded that catheter-based renal nerve ablation exerts acute and chronic effects on renal hemodynamics in a large animal model.
Human Data
EnligHTN I Study
EnligHTN I was the first in human study, using the multi-electrode SD Jude EnligHTN ablation system [37]. The study was designed to assess the safety and efficacy of the procedure. The primary efficacy objective was reduction of office blood pressure from baseline to 6 months. Blood pressure was measured at baseline, pre-discharge, 1-, 3-, and 6-month post-procedure. Additional efficacy data collection included changes in anti-hypertensive medication, home BP monitoring, and 24-h ambulatory BP. The primary safety objective was the rate of AEs. Other data collection included blood analysis: complete blood count, basic metabolic profile, serum creatinine concentration, eGFR using the modified diet in renal disease (MDRD) formula and serum cystatin C, urine analysis (albumin-to-creatinine ratio), 12-lead ECG, and renal artery evaluation (using duplex ultrasonography and/or CT scan).
The study enrolment was conducted from October 2011 to March 2012. A total of 62 patients were consented for enrolment. Sixteen patients were excluded during the screening process. One of the 16 patients was excluded from the study due to multiple renal arteries found on the screening renal angiogram. In total, 46 patients completed baseline evaluation and underwent the renal denervation procedure. Forty four of the 46 patients (96 %) met all the inclusion and no exclusion criteria. The baseline characteristics of study participants are depicted in Table 8.1.
Table 8.1
Baseline characteristics
n = 46* | |
---|---|
Gender (female) | 15 (33 %) |
Ethnic origin (white) | 45 (98 %) |
Body mass index (kg/m2) | 32 (5) |
Coronary artery disease | 9 (20 %) |
Hyperlipidemia | 27 (59 %) |
Type II diabetes mellitus | 15 (33 %) |
Sleep apnea | 14 (30 %) |
eGFR (mL/min/1.73 m2) | 87 (19) |
Serum creatinine (μmol/L) | 78 (17) |
Cystatin C (mg/L) | 1.14 (0.29) |
Number of anti-hypertensive medications | 4 (0.6) |
Office systolic blood pressure (mmHg) | 176 (16) |
Office diastolic blood pressure (mmHg) | 96 (14) |
Heart rate (bpm) | 71 (12) |
The procedure was generally performed with conscious sedation at the operators’ discretion. This was not mandated in the protocol. It included, but was not limited to the combination of intravenous midazolam and fentanyl, titrated as appropriate. Most patients experienced back pain during the denervation procedure, which was generally well controlled with sedation and analgesia. The median procedure time (from initiation to completion of RF energy delivery) was 34.0 min and the mean (+SD) number of ablations delivered was 7.7 (+0.8) for the right and 7.4 (+1.4) for the left renal arteries. One patient had the renal denervation procedure performed on a single side only due to difficulty engaging the other renal artery. The mean fluoroscopic time was 11.0 ± 7.1 min and the mean contrast volume used was 139.5 ± 93.2 ml. All the AEs were collected in the study.
A CEC adjudicated the events for seriousness and relatedness to the procedure and device. No serious vascular AEs occurred during the procedure, including no renal artery damage (i.e. no renal artery dissections, aneurysms, or flow limiting renal artery vasospasms) or serious vascular access site complications. Minor peri-procedural events which were attributed to either the device or procedure were reported and include: non-flow limiting vasospasms, vascular access site haematomas, hypotension, vasovagal episodes, bradycardia, transient haematuria, pain, and nausea. Vasospasm was reported as an AE although no specific threshold was established for reporting the event. An independent CEC reviewed all vasospasms and determined none were flow limiting (>50 % reduction in vessel lumen diameter). All minor peri-procedural events were reported to have resolved without further clinical sequelae. Serious AEs that were deemed possibly related to the procedure and/or device were reported in three (6.5 %) patients over 6 months of follow-up. Events include hypotension, progression of pre-existing renal artery stenosis, and a progression of hypertensive renal disease with an increase in serum creatinine.
Renal artery evaluation was conducted on all patients by CT imaging at 6 months. No patients developed a new haemodynamically significant renal artery stenosis. Two patients with pre-existing renal artery stenosis at baseline experienced asymptomatic progression of their renal artery stenosis at 6 months. One was adjudicated as serious (>50 % occlusion in artery diameter) and one was non serious.
No patient required clinical intervention at 6 months and their renal function parameters (eGFR, serum creatinine, and cystatin C values) remained stable. Renal function was evaluated by repeated measurements of eGFR, serum creatinine and cystatin C from baseline through 6 months of follow-up (Table 8.2). No patient experienced a reduction in eGFR >50 %, a twofold increase in serum creatinine, or progressed to end-stage renal disease. While the eGFR decreased (baseline mean of 87–82 mL/min/1.73 m2 at 6 months, p = 0.004) and serum creatinine increased (baseline mean of 78–83 mmol/L at 6 months, p = 0.004), the mean cystatin C decreased (baseline mean of 1.14–1.00 mg/L at 6 months, p = 0.00013). To further evaluate changes in renal function, a more meaningful assessment of eGFR with a clinically relevant cut-off of <60 mL/min/1.73 m2 was also undertaken. At baseline, three patients had an eGFR level of <60 mL/min/1.73 m2. One of these patient’s eGFR remained <60, while the other two improved to 60 or greater at 6 months. In contrast, two other patients with baseline eGFR values >60 (63 and 61) experienced an eGFR reduction <60. The urine albumin-to-creatinine ratio decreased significantly throughout the course of the study, with absolute results at baseline 169.4 mg/g, 1 month 142.9 mg/g, 3 months 141.2 mg/g, and 6 months 139.3 mg/g (p = 0.007).
Table 8.2
Renal function
Baseline n = 46 | Month 1 n = 46 | p value | Month 3 n = 46 | p value | Month 6 n = 45 | |
---|---|---|---|---|---|---|
eGFR, mL/min/1.73 m2 | 87 (19) | 85 (20) | 0.32 | 84 (22) | 0.21 | 82 (20) |
Serum creatinine, μmol/L | 78 (17) | 79 (19) | 0.26 | 81 (20) | 0.19 | 83 (20) |
Cystatin C, mg/l | 1.14(0.29) | 1.00 (0.25) | <0.0001 | 0.97 (0.20) | <0.0001 | 1.00 (0.23) |
Compared with baseline, office and ambulatory systolic blood pressure of the entire cohort significantly decreased at all-time points (p < 0.0001). The average office blood pressure (mmHg) at baseline was 176/96 mmHg. The resulting average office blood pressure reductions from baseline at 1 month, 3 months, and 6 months were 22.8/21.0, 22.7/21.0, and 22.6/21.0 mmHg. Over the follow-up period, as many as 80 % of patients had a reduction in office systolic blood pressure of at least 10 mmHg or greater and up to 41 % had an office blood pressure of <140 mmHg. Baseline systolic blood pressure was a strong predictor of response (p < 0.0001). In addition, the in-office resting heart rate was collected at baseline (71 beats/min), 1 month (69 beats/min), 3 months (67 beats/min), and 6 months (66 beats/min) and demonstrated a decrease over time (P = 0.007 at 6 months). The baseline HR was a predictor of change in office systolic blood pressure (r = 0.31, p = 0.039). In addition, the reduction in HR was correlated with reduction in office systolic blood pressure (r = 0.33, P = 0.025). The average 24-h ambulatory BP at baseline was 150/83 mmHg. The average 24-h ambulatory blood pressure reduction from baseline to 1 month, 3 months, and 6 months was 10/5, 10/5, and 10/6 mmHg, respectively. The change in average 24-h ambulatory blood pressure correlated with both the change in office systolic blood pressure (r = 0.56, p < 0.0001) and office diastolic blood pressure (r = 0.55, p < 0.0001). These reductions in office and ambulatory blood pressures were achieved with minimal modifications to the cohort’s antihypertensive medical regimen during the follow-up period. Over the 6-month follow-up period, six (13.0 %) patients had a decrease in their antihypertensive medications and four (8.7 %) had an increase in antihypertensive medications. For patients that did not have an increase or decrease in their antihypertensive medications the 6-month office BP reduction was similar to the entire cohort at 22.5/21.0 mmHg, as well as mean ambulatory blood pressure (ABPM) reductions of 10/5 mmHg.
Effect of Renal Nerve Ablation on LVH and Heart Failure Variables
The beneficial effects of renal nerve ablation in resistant hypertension may extend beyond blood pressure. In a sub-study we assessed whether multi-electrode catheter-based renal nerve ablation has favorable effects on left ventricular structural and functional indices, as well as on neurohormonal activation reflected by N-terminal pro B-type natriuretic peptide (NT pro-BNP). This hypothesis was tested in twenty patients with resistant hypertension (age: 57 ± 10 years, 13 males, office blood pressure: 180/96 ± 19/16 mmHg receiving 4.4 ± 0.61 drugs) that underwent renal denervation and were followed for 6 months. A full transthoracic echocardiographic study was performed in all patients at both baseline and follow-up. Left ventricular mass was calculated using the Devereux formula and was indexed for body surface area and height, while tissue Doppler imaging indices of diastolic function were measured. Moreover, blood sampling was performed in order to estimate metabolic profile and NT pro-BNP levels.
At 6 months following renal nerve ablation, office systolic and diastolic blood pressure was significantly reduced and the mean interventricular septal thickness and left ventricular mass index substantially decreased. Left atrial diameter and volume were reduced from and LV function improved. Renal nerve ablation resulted in an increase of mitral valve E′/A′ ratio and a decrease in the E/E′, whereas isovolumic relaxation time was shortened. Furthermore, renal denervation resulted in a statistically significant reduction in NT pro-BNP levels. From these data we concluded that in patients with resistant hypertension and left ventricular hypertrophy, renal nerve ablation besides blood pressure reduction leads to favorable cardiac remodeling and attenuation of neurohormonal overdrive.
Effect of Renal Nerve Ablation on Arrhythmias
We assessed the effects of renal denervation on heart rate variability and cardiac arrhythmia. Fourteen patients with resistant hypertension underwent ambulatory blood pressure measurements and Holter monitoring at baseline and at 1 and 6 months after renal denervation using the EnligHTN ablation catheter. Supraventricular and ventricular ectopic activity was recorded in all patients. SyneScope analysis system calculated the recommended time- and frequency-domain parameters of heart rate variability.
Office and 24-h systolic and diastolic blood pressure were significantly reduced both at 1 and 6 months after renal denervation. The total number of premature supraventricular and ventricular contractions was significantly decreased both at 1 and at 6 months after renal denervation. A significant increase was observed in time and frequency domain indexes during the 24-h Holter monitoring both 1 and 6 months after the procedure. We concluded that renal nerve ablation in addition to blood pressure lowering, significantly reduces mean heart rate and arrhythmia burden while it restores autonomic balance in patients with resistant hypertension, providing evidence for the beneficial impact of denervation-induced neuro-modulation on heart rhythm.
The Future of RNA in Resistant Hypertension: Issues to Be Addressed
Resistant hypertension is likely to remain the main target of renal nerve ablation in the near future. Several issues however need to be addressed before getting wide application in this patient population. Unsolved issues can be categorized to procedure-related and device-related, safety-related, and efficacy-related.
Procedure-Related and Device-Related Unsolved Issues
As of today, renal nerve ablation is a ‘blind’ procedure. All renal ablation systems aim to achieve near-complete disruption of renal sympathetic fibers through the circumferential placement of ablating electrodes and the performance of several (4–8) discrete ablation lesions. However, there is currently no accurate method to confirm intra-procedurally the success of renal nerve ablation and guide the interventionalist to stop or continue the procedure. Recently, two tests have been proposed as markers of procedural success: electrical stimulation of renal arterial nerves [38], and renal blood flow during dopamine infusion [36]. Whether the proposed tests or other methods will actually prove to be accurate markers of procedural success remains to be validated in future studies.
The relative contribution of efferent and afferent renal sympathetic fibers in the pathogenesis of resistant hypertension remains poorly clarified. Subsequently, the effects of renal nerve ablation on efferent and afferent sympathetic nerve disruption and the contribution of each component on blood pressure reduction is not known. Furthermore, the possibility of sympathetic nerve re-generation following renal nerve ablation raises further questions. In addition, whether nerve re-generation takes place in the anatomical level alone or it is functional as well remains to be verified by future basic research in this field.
Current availability of six devices for renal nerve ablation and the active research for the development of many more devices (approximately 60 devices are under development) raises two important questions: (a) whether the source of energy plays an important role for the success of renal nerve ablation and the efficacy and safety of the procedure, and (b) whether clinically significant differences between the devices using the same energy source exist. Head-to-head trials are needed to answer these clinically meaningful questions. Until then, indirect comparisons may be useful, but special attention is needed in order to avoid inappropriate comparisons and premature conclusions.
Safety-Related Unresolved Issues
Safety issues represent another considerable concern. Up to now, no serious or life threatening adverse events have been reported. Vascular complications seem rare and are not usually irreversible. So far, minor vasospasm of the renal artery post-denervation, small hematomas, pseudo-aneurysms, minor bleeds, and one dissection requiring stenting have been reported. Endothelial injury at the site of ablation lesions seems to be transient and re-endothelization occurs soon enough. Clot formation at ablation sites can be prevented by appropriate antithrombotic and antiplatelet therapy.
Renal artery stenosis is another significant concern, since it was observed with radiofrequency ablation for the management of atrial fibrillation. Fortunately enough the probability of renal artery stenosis seems much lower than in the pulmonary and coronary arteries, possibly due to the fact that the energy delivered for renal ablation is much lower than the energy delivered for atrial ablation and the diameter of renal artery is much greater than the coronary and pulmonary vessels. Indeed, only two cases of renal artery stenosis due to renal nerve ablation have been reported so far [39, 40], while the procedure has been already performed in several thousand patients.
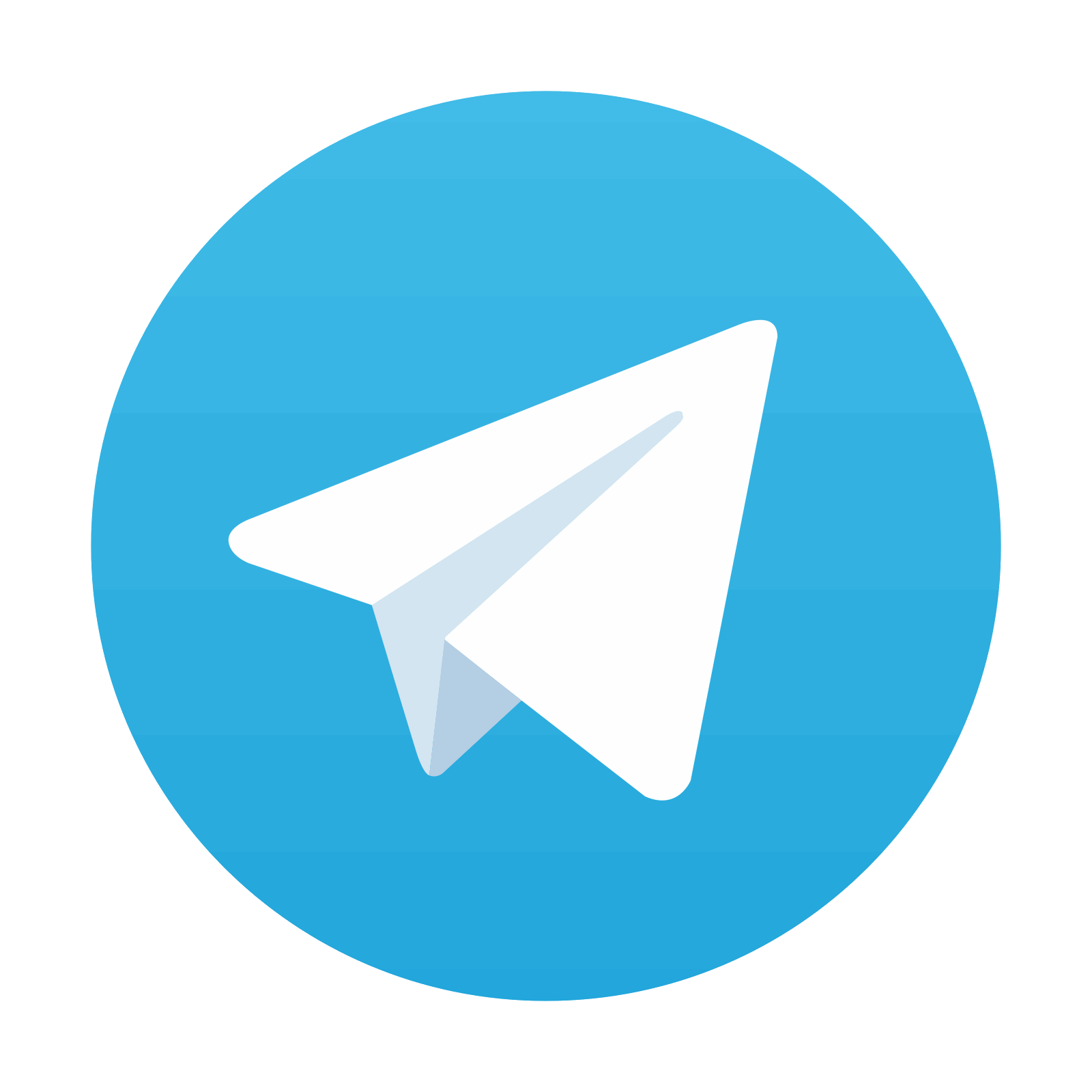
Stay updated, free articles. Join our Telegram channel

Full access? Get Clinical Tree
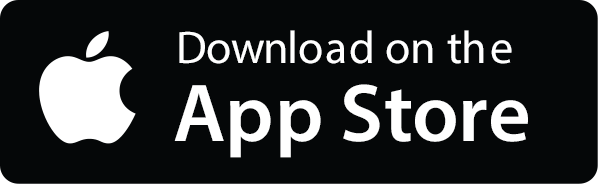
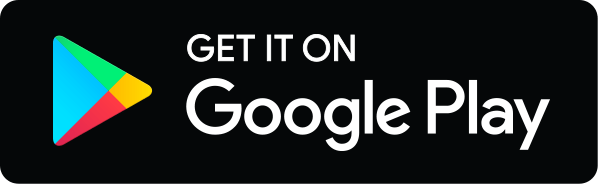