Chapter 42 Surgical Robotics
General surgery
CoBRASurge
The University of Nebraska Medical Center for Advanced Surgical Technology (our laboratory), in association with the University of Nebraska—Lincoln Department of Mechanical Engineering, has created a robot for surgical assistance. The CoBRASurge (Complex Bevel-geared Robot for Advanced Surgery) is a small device for laparoscope tool movement and placement that consists of a compact table-mounted robot with multiple gears and a joystick for movement and control (Fig. 42-1). It uses a bevel-geared mechanism and a spherical mechanism that allow the tools and laparoscope to center on a specific point in space, namely, the trocar. The device mounts to the operating room table, so it does not require floor space. The robot can control a laparoscopic camera and articulated robotic graspers. In its current application, it is controlled from a joystick across the room. Our plans are to engender surgeon control of the robot with a foot joystick. The compact design of this robot allows increased room around the operating table for personnel and instruments.
VECTOR Capsule Endoscope
The aim of the VECTOR project (Versatile Endoscopic Capsule for gastrointestinal TumOr Recognition and therapy) is to develop a mobile endoscopic robot with future surgical applications. The project currently includes 19 university laboratory participants in Europe, including the Center for Research in Microengineering (CRIM) in Italy, with input from Vanderbilt University’s MED lab. The original VECTOR prototype consisted of a cylindrical body with a camera and two motorized sets of six legs that unfolded from the body of the robot to interact with the intestinal wall (Fig. 42-2). These legs allowed the robot to “walk” insect-like along a small distance in the intestine. The legs are made of heat-treated nitinol, a flexible nickel-titanium alloy with elastic properties designed to reduce the risk for perforation. The robot was engineered to permit passage through a deflated colon in order to reduce one of the main sources of pain from gastrointestinal imaging procedures (i.e., an insufflated colon). This ability is partially facilitated by the robot’s unique leg design. The 12 legs are capable of independent motion, which can aid in tight turns, such as the splenic flexure. The robot has been successfully tested in a porcine model. The VECTOR robot may represent an improvement on current swallowable endoscopes because of its movement control and possible future augmentation with accessories, such as for biopsy.
Therapeutic Capsule Endoscope
Carnegie Mellon’s NanRobotics Laboratory is currently developing a capsule endoscope that can stop and reorient itself during passage through the gastrointestinal system. The robot consists of a cylindrical body with three legs extending from the sides; the legs are covered with a micropatterned elastomer adhesive designed to mimic the feet of a gecko so that the robot can “stick” to the walls of the gut (Fig. 42-3). The legs, when activated, open outward and come in contact with the intestinal lining, causing increased friction and decreasing capsule velocity. Once the area of interest has been examined and biopsied, the legs then peel off, permitting anterograde movement. Further plans for this robot include tools for biopsy and cauterization.
SILS and NOTES Applications
ARES (Assembling Reconfigurable Endoluminal Surgical System)
The ARES robot (Fig. 42-4), developed by a consortium of European universities and laboratories, was designed for transgastric surgical applications and represents an attempt to address some of the limitations of endoscopic or natural orifice procedures. The robot consists of multiple modular units molded from an acrylic photopolymer. The current module size is 36.5 mm in length and 15.4 mm in diameter, or about the size of a current “pill cam.” These dimensions are designed to allow the patient to swallow the modules. Once in the stomach, the robot undergoes magnetic self-assembly. The researchers have demonstrated a 74% success rate for self-assembly in a model stomach. The robot’s placement potentially could be tracked by ultrasonic emissions from one of the modules. Power would be supplied by an onboard battery or with electromagnetic induction. Currently, the design requires a number of modifications to be usable in human surgery.
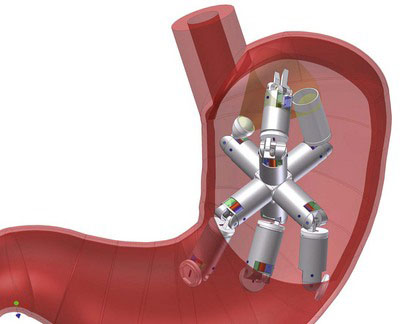
FIGURE 42-4 The ARES device (Assembling Reconfigurable Endoluminal Surgical System) shown assembled within the stomach.
(Courtesy of Dr. Kanako Harada.)
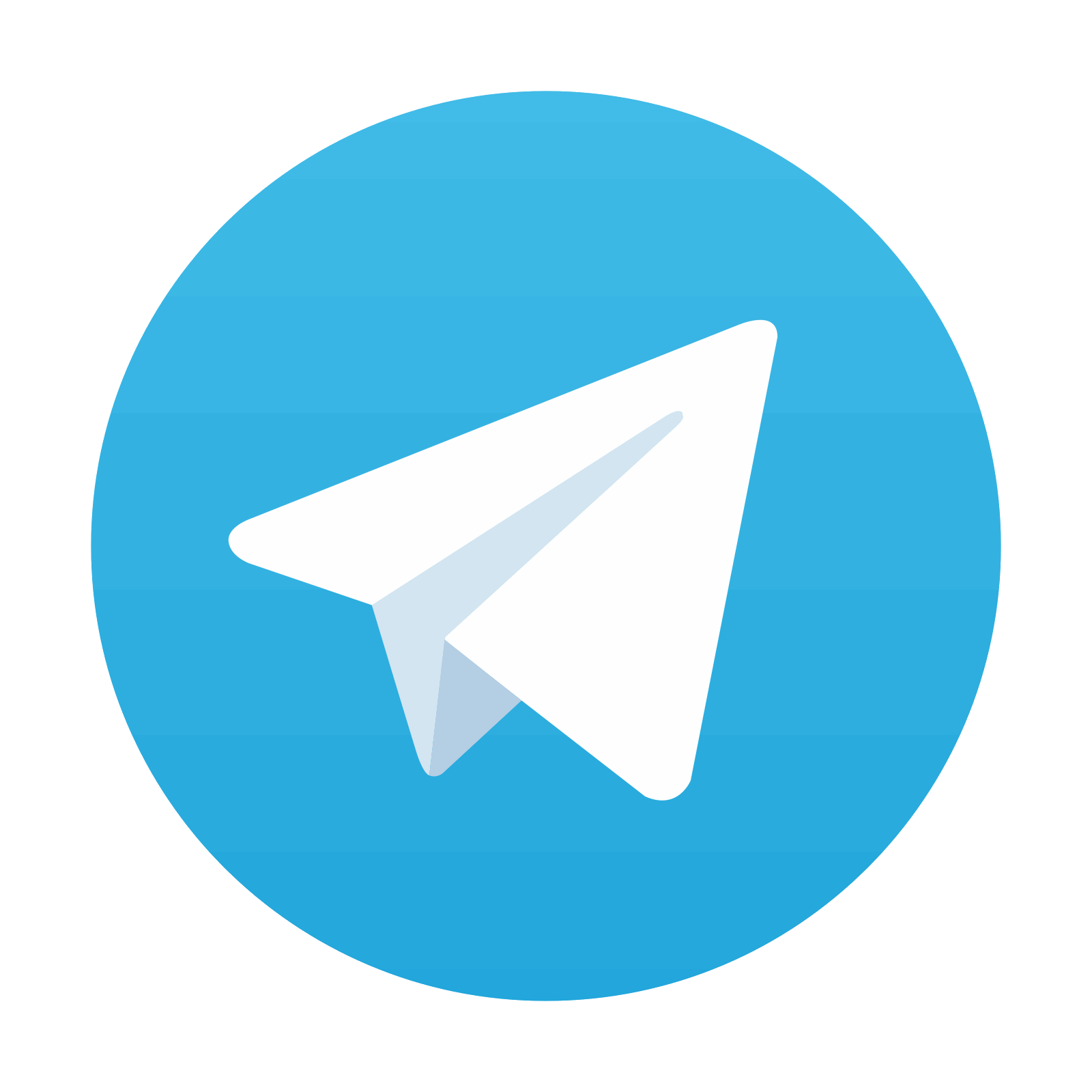
Stay updated, free articles. Join our Telegram channel

Full access? Get Clinical Tree
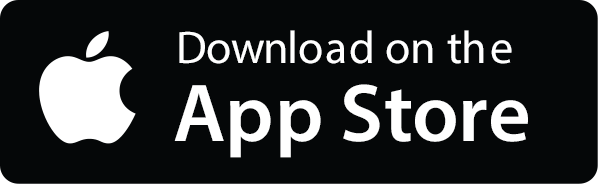
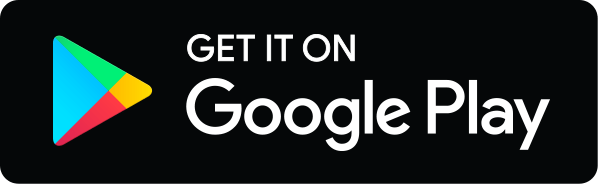