The treatment of kidney stone disease has changed dramatically over the past 30 years. This change is due in large part to the arrival of extracorporeal shock wave lithotripsy (ESWL). ESWL along with the advances in ureteroscopic and percutaneous techniques has led to the virtual extinction of open surgical treatments for kidney stone disease. Much research has gone into understanding how ESWL can be made more efficient and safe. This article discusses the parameters that can be used to optimize ESWL outcomes as well as the new concepts that are affecting the efficacy and efficiency of ESWL.
Key points
- •
Stone radiodensity and skin to stone distance can both be used to optimize extracorporeal shock wave lithotripsy (ESWL) outcomes.
- •
The importance of acoustic coupling cannot be overemphasized. Air pockets within coupling gel can significantly reduce ESWL efficiency.
- •
ESWL outcomes are maximized using a frequency of 60 shock waves per minute compared with 120 shock waves per minute.
- •
Power ramping with a brief pause can not only improve ESWL stone fragmentation outcomes but also decrease renal tissue injury.
- •
Lithotripters with larger focal zones have superior stone fragmentation rates along with better renal safety profiles.
- •
Improved acoustic feedback and stone monitoring systems, as well as residual stone fragment clearance techniques, are being developed that will further improve the stone fragmentation outcomes of ESWL.
Introduction
The treatment of kidney stone disease has changed dramatically over the past 30 years. This change is due in large part to the arrival of ESWL. Before the advent of ESWL in the early 1980’s, most kidney stones were treated with open surgery. ESWL along with the advances in ureteroscopic and percutaneous techniques has led to the virtual extinction of open surgical treatments for kidney stone disease.
The first successful ESWL treatment was accomplished in 1980 in Germany by Dr Christian Chaussy using a Dornier HM1 lithotripter. Owing to its effectiveness and its rare side effects, ESWL was quickly approved by the US Food and Drug Administration (FDA) for clinical use. Dr James Lingeman using the unmodified Dornier HM3 lithotripter performed the first ESWL procedure in North America. Since then, ESWL has been used with increasing frequency to treat more and more complex stones. Multiple sources have confirmed that kidney stone disease is on the rise, and as a result, surgical treatments for these kidney stones are also on the rise. Turney and colleagues have recently shown that over the past decade, ESWL for upper tract stones has increased by 55%. Most of this increase was caused by ESWL performed on kidney stones (69% increase). ESWL performed on the ureter remained stable. With the increase in stone disease, surgical treatment must not only be effective but also be efficient.
Initial work with ESWL was very optimistic because it was reported to be extremely effective even when applied to complex stone cases. Furthermore, this noninvasive technique was found to have very few side effects. With the increasingly widespread acceptance and use of ESWL, it was discovered that this procedure was associated with a few important limitations. Some urinary stones were found to be resistant, and their fragmentation could not be accomplished consistently using ESWL. Also, some renal stones that could be fragmented would not fragment completely and secondary treatments were necessary. In addition, renal anatomy (calyceal diverticulum or acute infundibulopelvic angles) and location (lower pole calyx) of the stone were crucial to ESWL stone-free outcomes. Finally, many reports have demonstrated the frequent minor complications and the rare major complications.
For the reasons stated above, much research has gone into understanding how ESWL can be made more efficient and safe. This article discusses the parameters that can be used to optimize ESWL outcomes as well as the new concepts that are affecting the efficacy and efficiency of ESWL.
Introduction
The treatment of kidney stone disease has changed dramatically over the past 30 years. This change is due in large part to the arrival of ESWL. Before the advent of ESWL in the early 1980’s, most kidney stones were treated with open surgery. ESWL along with the advances in ureteroscopic and percutaneous techniques has led to the virtual extinction of open surgical treatments for kidney stone disease.
The first successful ESWL treatment was accomplished in 1980 in Germany by Dr Christian Chaussy using a Dornier HM1 lithotripter. Owing to its effectiveness and its rare side effects, ESWL was quickly approved by the US Food and Drug Administration (FDA) for clinical use. Dr James Lingeman using the unmodified Dornier HM3 lithotripter performed the first ESWL procedure in North America. Since then, ESWL has been used with increasing frequency to treat more and more complex stones. Multiple sources have confirmed that kidney stone disease is on the rise, and as a result, surgical treatments for these kidney stones are also on the rise. Turney and colleagues have recently shown that over the past decade, ESWL for upper tract stones has increased by 55%. Most of this increase was caused by ESWL performed on kidney stones (69% increase). ESWL performed on the ureter remained stable. With the increase in stone disease, surgical treatment must not only be effective but also be efficient.
Initial work with ESWL was very optimistic because it was reported to be extremely effective even when applied to complex stone cases. Furthermore, this noninvasive technique was found to have very few side effects. With the increasingly widespread acceptance and use of ESWL, it was discovered that this procedure was associated with a few important limitations. Some urinary stones were found to be resistant, and their fragmentation could not be accomplished consistently using ESWL. Also, some renal stones that could be fragmented would not fragment completely and secondary treatments were necessary. In addition, renal anatomy (calyceal diverticulum or acute infundibulopelvic angles) and location (lower pole calyx) of the stone were crucial to ESWL stone-free outcomes. Finally, many reports have demonstrated the frequent minor complications and the rare major complications.
For the reasons stated above, much research has gone into understanding how ESWL can be made more efficient and safe. This article discusses the parameters that can be used to optimize ESWL outcomes as well as the new concepts that are affecting the efficacy and efficiency of ESWL.
Optimizing parameters
Numerous parameters can be used to optimize ESWL outcomes. These parameters include stone characterization, acoustic coupling, and shock wave rate and sequence.
Stone Characterization
One of the main drawbacks of ESWL is its inability to fragment certain types of stones. This drawback is extremely important because patients who harbor these types of stones will be subjected to ESWL and will thus be exposed to its complications (minor and major) without the achievable benefit of fragmentation. In addition, failure of stone fragmentation will lead to secondary treatments, which will increase medical costs. The stones most resistant to ESWL include brushite, calcium oxalate monohydrate, and cystine. Therefore, if one could predict the type of stone, then the likelihood of that stone being fragmented by ESWL could be predicted. Numerous studies have demonstrated that noncontrast computed tomography (NCCT) can be used to measure differences in radiodensity, which can be used to distinguish between different types of stones. More recently, studies have shown that preoperative NCCT can be used to predict the likelihood of stone fragmentation. El-Assmy and colleagues demonstrated that stones with Hounsfield units (HU) greater than 1000 required a statistically significant higher number of shock waves to be fragmented. Also, Wang and colleagues concluded that stone densities greater than 900 HU were a significant predictor of ESWL failure.
Along with the type of stone, the distance of the stone from the patient’s skin can have a significant impact on the likelihood of stone fragmentation. Numerous studies have shown that the distance of the stone from the skin, also known as the skin to stone distance (SSD) can be used as an indicator of the likelihood of stone fragmentation. SSD is measured by averaging 3 fixed distances from the stone to the skin. These 3 measurements include 1 horizontal, 1 vertical, and 1 diagonal measurement from the stone to the skin. Pareek and colleagues found SSD to be a statistically significant predictor of ESWL failure when it was greater than 10 cm. In 2009, Patel and colleagues found that patients with an average SSD of 10.7 cm had a statistically significant higher rate of residual stone after ESWL compared with those who had an average SSD of 8.3 cm.
As both SSD and stone radiodensity have been demonstrated to be independent predictors of stone fragmentation by ESWL, Perks and colleagues used both these factors to evaluate their combined ability to predict stone fragmentation by ESWL. In this retrospective study on 111 patients, Perks and colleagues found that stones with a radiodensity of less than 900 HU combined with an SSD of less than 9 cm predicted ESWL success, an observation that was independent of stone size, location, or body mass index.
As mentioned previously, cystine calculi are extremely hard to fragment with ESWL. Kim and colleagues evaluated the hypothesis that cystine stones with specific morphologies might be easier to fragment with ESWL. This study demonstrated that cystine stones with a “rough” morphology contained void regions that are visible on NCCT. The investigators concluded that cystine calculi that appeared homogenous on NCCT required 61% more shock waves for fragmentation than did stones that appeared heterogeneous.
In summary, careful attention to stone characterization, including SSD and stone radiodensity, can be used to accurately select patients who have a greater possibility of having their stones fragmented using ESWL. This ability will translate into fewer secondary treatments, which will cut down on medical costs.
Acoustic Coupling
Over the past 30 years, ESWL has gone through numerous changes with the ultimate goal of complete and universal stone fragmentation with minimal side effects. Much research has gone into the achievement of this goal. One parameter that has recently undergone reevaluation is acoustic coupling. The HM3 uses water as a medium for coupling, which has been found to be ideal. This is because body tissue has an acoustic impedance very close to that of water, and therefore, a shock wave generated in water will pass into the body with minimal reflection or absorption of energy at the water–skin interface. Unlike the Dornier HM3, modern lithotripters have incorporated dry head energy sources that have treatment heads that are brought into contact with the patient. At present, the only machine that does not have a “dry head” is the Storz SLX (Storz Medical AG, Tägerwilen, Switzerland), which uses a partial water bath for coupling. Typically, gel is used to couple the dry head lithotripters to the skin of the patients. Once the gel is applied, the head of the lithotripter is brought into contact with the patient’s skin. An important potential problem of gel coupling is the introduction of air pockets into the gel. These air pockets, which are often abundant, have a deleterious effect on shock wave propagation. Shock waves travel well through water and coupling gels, but not through air.
In 2006, Pishchalnikov and colleagues demonstrated a negative relationship between increasing air pockets and ESWL efficiency. More specifically, using a Dornier DoLi-50 electromagnetic lithotripter, this in vitro study established that with as little as 2% of the coupling area covered with air pockets, there was a reduction in stone breakage by 20% to 40%. Jain and colleagues found that optimal fragmentation was obtained using bubble-free ultrasound gel. In addition, Bergsdorf and colleagues demonstrated in a clinical study that gel with lower viscosity and better quality provided significantly better stone fragmentation. Finally, in a study by Neucks and colleagues it was discovered that the quality of coupling can be improved by the way the gel is handled and applied. The most effective way to apply coupling gel is to place it directly from the container as a mound to the center of the treatment head. Then, without spreading it around, the treatment head should be pressed against the patient.
The importance of acoustic coupling through gel application cannot be overemphasized. It is paramount that air pockets be limited so that shock waves can be delivered with the greatest efficiency. If coupling is overlooked, ESWL becomes less effective, leading to inferior outcomes and the need for increased secondary treatments.
Shock Wave Rate
Another important parameter that has a significant impact on ESWL outcomes is the rate at which shock waves are delivered. In vitro work first reported that slowing the rate at which shock waves are delivered can have a major impact on both stone fragmentation and acute renal tissue damage. Numerous prospective clinical trials have confirmed that ESWL stone fragmentation outcomes are improved at 60 shock waves per minute compared with the standard 120 shock waves per minute. Porcine models have also demonstrated that acute renal injury can be reduced at 60 shock waves per minute. A meta-analysis by Semins and colleagues showed that a treatment performed at a rate of 60 shocks per minute is associated with a higher rate of treatment success than treatment performed at a rate of 120 shocks per minute. One drawback of slowing the rate of shock wave delivery is the increased time required to break urinary calculi, which may extend the time that the patient is subjected to sedation.
One hypothesis proposed for the decreased stone fragmentation with an increased shock wave rate was an increase in the formation of cavitation bubbles, which would interfere with the transmission of the positive pressure phase of shock waves. However, Pishchalnikov and colleagues proposed an alternative explanation suggesting that the main reason was actually the loss of the negative pressure portion of the shock wave and not the interference with the positive pressure portion.
In summary, many studies have confirmed that ESWL outcomes are maximized using a frequency of 60 shock waves per minute compared with 120 shock waves per minute. These outcomes includes a higher rate of stone fragmentation as well as a decreased rate of renal tissue damage.
Shock Wave Sequence
The last ESWL parameter to consider, which can have a dramatic impact on ESWL outcomes, including stone fragmentation and effect on acute renal tissue injury is the sequence that is used to deliver the shock waves. Sequence refers to the timing of shock waves and the number of shock waves given at a specific power level. Power ramping refers to the delivery of several shock waves at lower power levels before getting to the desired treating power level. Most centers treating under sedation perform some form of power ramping to help the patient get accustomed to the shock waves.
In in vitro studies on pigs, power ramping has been shown to enhance stone breakage. In vitro studies using the Dornier HM3 lithotripter demonstrated that progressively increasing the output voltage can produce superior stone comminution. It has also been confirmed that stepwise power ramping can significantly decrease renal injury. In a porcine model, treatment with 100 shocks at 18 kV followed by 2000 shocks at 24 kV significantly decreased acute renal lesion sizes compared with 2000 shocks at 24 kV. It was thought that the key to the protective effect in power ramping was a brief pause (roughly 3 minutes) between the lower and higher power settings. However, more recently Handa and colleagues confirmed that renal protection can be achieved without instituting a pause in ESWL treatment but that a ramping protocol is, however, necessary.
Therefore, power ramping with a brief pause can not only improve ESWL stone fragmentation outcomes but also decrease renal tissue injury and should be incorporated into all ESWL protocols.
In conclusion, optimizing parameters including SSD, stone radiodensity, acoustic coupling, reduced shock wave rate, and power ramping can and should always be applied when performing ESWL. Application of these will not only improve stone fragmentation but also decrease renal tissue injury. These simple yet effective techniques will reduce, if not eliminate, the need for secondary procedures, which will in turn reduce medical costs.
New concepts
With the tremendous amount of research being done in ESWL, more and more new concepts are being investigated. This section concentrates on these newer concepts including small versus large focal zone lithotripters, tandem and dual head lithotripters, acoustic feedback systems, stone monitoring systems, and residual fragment clearance techniques.
Small versus Large Focal Zone Lithotripters
ESWL acoustic energy is focused on to a relatively small zone surrounding the focal point of the lithotripter. The focal point is a geometric point and is usually the location of the kidney stone of interest. The focal zone can be either small or large, and the amount of energy or peak pressure that is applied to it can be manipulated. This relationship is also known as the acoustic output, which is the amplitude and spatial distribution of the acoustic energy delivered to a specific focal volume. The original Dornier HM3 produces peak positive pressures of about 40 MPa on a focal width of about 10 to 12 mm. Most modern lithotripters generate higher pressures between 60 and 160 MPa delivered to a more narrow focal width of 3 to 6 mm. The XX-ES CS-2012A (Xi Xin Medical Instruments Co. Ltd, Suhou, China) and the LithoGold LG-380 (Tissue Regeneration Technologies, Woodstock, GA, USA) produce low pressures (roughly 20 MPa) with broad focal zones (roughly 18–20 mm).
In vitro studies have demonstrated that a larger focal zone can improve stone breakage. Owing to respiratory movements, urinary stones are in continual motion. It is hypothesized that the stone will have a greater chance of staying within the target focal zone if the zone is larger. Pishchalnikov and colleagues demonstrated this hypothesis in an in vitro study. Furthermore, lithotripters with tighter focal zones tend to have fewer shock waves that actually hit the stone and more shock wave energy that is deposited directly into renal tissue. This mechanism is not the only one by which large focal zone lithotripters improve stone breakage. Focal width also seems to have a crucial role in the mechanism of stone comminution. Numerical modeling studies have shown that shear waves necessary to cause large internal stresses are enhanced when the focal width is larger than the diameter of the stone.
An important aspect of ESWL is safety, and numerous studies have shown that the use of a tighter focal zone with higher peak pressures is associated with not only a higher retreatment rate but also a higher rate of side effects when compared with a wider focal width. Porcine models using treatment protocols recommended by both the XX-ES CS-2012A and the LG-380 broad focal zone lithotripter machines produce minimal renal lesions.
Tandem and Dual Head Lithotripters
Tandem head lithotripters
As ESWL progresses, more and more research is being focused on ways of improving the fragmentation of stones. In light of this, efforts have been made to enhance one of the key mechanisms of stone breakage. Cavitation bubbles are an essential part of stone comminution, and a novel idea is to use 2 shock waves in rapid succession to drive the forceful collapse of bubbles against the stone. This method of stone breakage has been achieved by 2 methods. One is to add an auxiliary piezoelectric array to generate a second shock wave along the same axis as the first shock wave. This method has led to significant improvements in stone comminution in vitro. The other method is to fit a piezoelectric lithotripter with an additional charging and discharge circuit to produce a second pulse. Fernandez and colleagues found clinically that this technique did not increase the efficiency of fragmentation but that it did significantly decrease the time required to fragment the stone.
Dual head lithotripters
Dual head lithotripters deliver closely timed shock waves from different treatment heads that are aligned to the exact same focal point. Initial reports suggest that these machines can be safe and effective.
Acoustic Feedback Systems
The main disadvantages of ESWL are the inefficiency side effects associated with it. These disadvantages are interrelated in that excess shock waves are commonly applied in an effort to assure stone breakage and ESWL effects on tissue are related to the shock wave dose. Recent research has demonstrated the possible role for an acoustic feedback system to monitor stone fragmentation. This acoustic feedback system uses a broadband receiver to monitor shock waves and reverberations from the acoustic wave transmitted into the stone. As the stone fragments, reverberations from smaller fragments generate higher frequency signals. In vitro studies have demonstrated the feasibility of this system to discriminate between fragments that differ in size by only 1 to 2 mm. This system allows the urologist to know when the stone is fragmented and therefore reduces excess or unnecessary shock waves to the renal tissue.
Stone Monitoring Systems
Once a stone has been localized, its movement in and out of the focal zone affects fragmentation. The longer the stone remains within the focal zone, the more likely it is to fragment. Maintaining the stone in the focal zone is challenged by respiratory motion. Depending on the patient’s respiratory rate, the stone can be outside the focal zone during 50% or more of the administered shock waves. This movement translates to more shock wave energy missing the stone and hitting renal tissue instead.
Several targeting systems have been developed to track stones during ESWL. These systems use ultrasound imagers, tracking algorithms, and piezoelectric lithotripters with built-in systems to continually locate the stone before shock wave firing. Although these systems show promise, none of them are currently being used in the clinical setting.
Residual Fragment Clearance Techniques
ESWL has been proved to be an excellent treatment of renal stone disease. However, one of its main limitations is the clearance of residual fragments, which will often lead to secondary treatments. This drawback is especially important for lower pole calculi that are subjected to ESWL. Numerous methods of residual fragment clearance have been proposed including percussion, diuresis, and inversion therapy. The success of these methods of residual stone fragment clearance have been limited.
There has been recent research in the domain of focused ultrasound technology directed at residual stone fragment clearance, and it shows great promise. Shah and colleagues recently demonstrated the use of focused ultrasound technology in a study involving live porcine models implanted with human stones. With this technology they were able to expel calculi effectively and safely from the kidney in their animal model. This technology could hold promise in the clearance of residual fragments after ESWL.
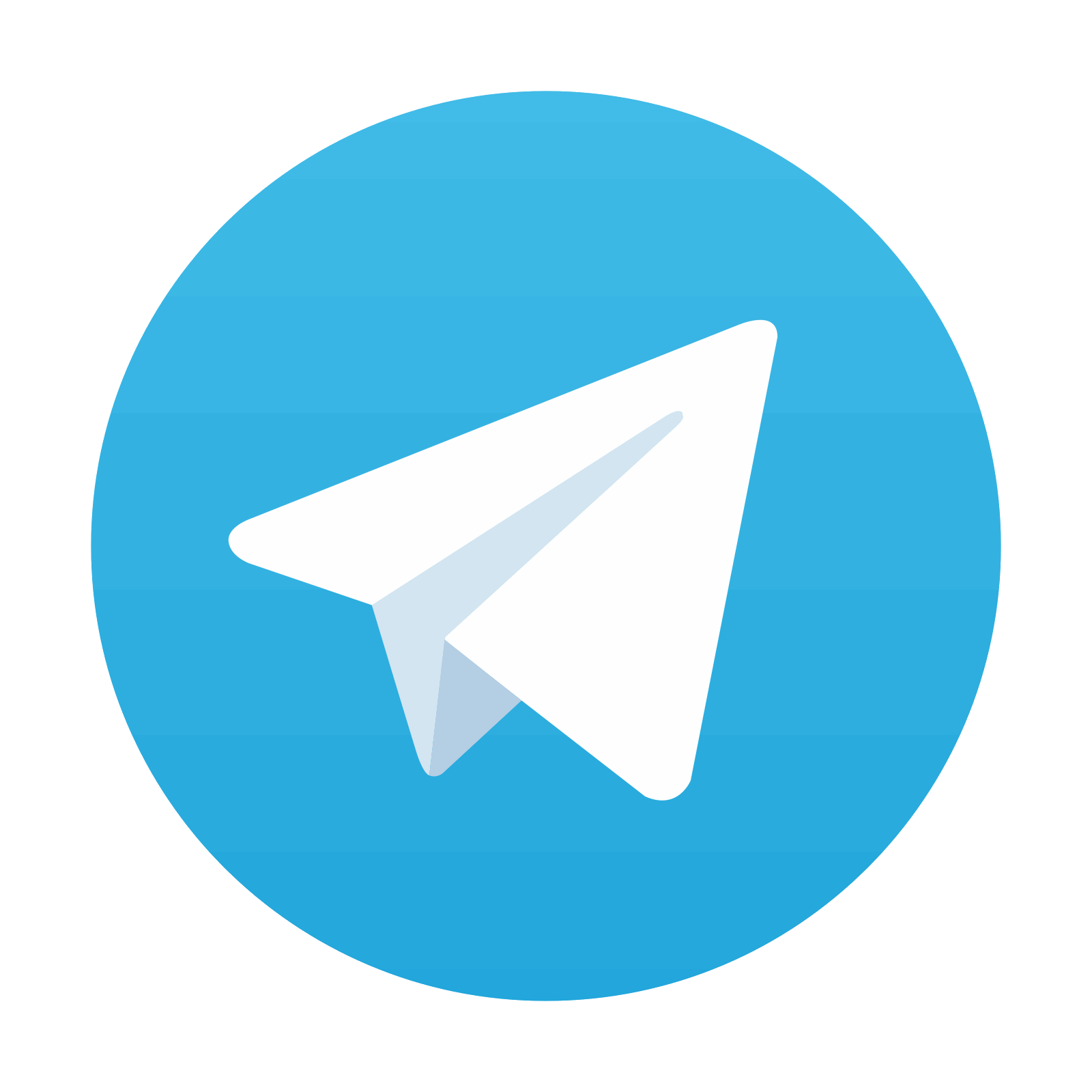
Stay updated, free articles. Join our Telegram channel

Full access? Get Clinical Tree
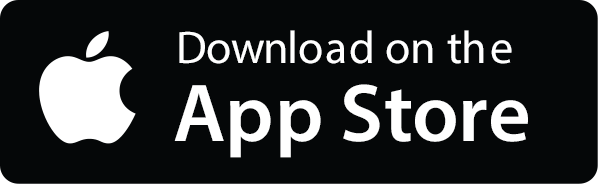
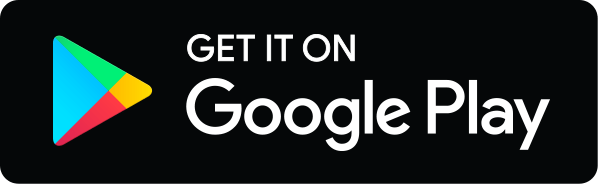