Fig. 2.1
Two-layers of anti-inflammatory mucosal immune defense preserving the integrity of the intestinal epithelial barrier. Depiction of the homeostatic mechanisms: (1) Immune exclusion to control epithelial colonization of microorganisms and penetration of exogenous antigens. This first line of defense is principally mediated by secretory antibodies of the IgA (and IgM) class in cooperation with innate protective factors (not shown). The secretory antibodies are actively exported by the epithelial polymeric Ig receptor (pIgR), also called membrane secretory component (mSC). Secretory immunity is preferentially stimulated by particulate antigens such as pathogens taken up through M cells (M) located in the dome epithelium covering gut-associated lymphoid tissue (see Figs. 2.2 and 2.3). (2) Innocuous soluble antigens (e.g., food proteins; magnitude of normal uptake indicated) and the commensal microbiota are also stimulatory for secretory immunity (graded arrows), but induce additionally suppression of pro-inflammatory Th2-dependent responses (IgE antibodies), Th1-dependent delayed-type hypersensitivity (DTH), IgG antibodies, and Th17-dependent neutrophilic reactions. This homeostatic Th-cell balance is regulated by a complex mucosally induced phenomenon called “oral tolerance.” The suppressive effects can be observed both locally and in the periphery, and are mediated by regulatory T (Treg) cells
Oral tolerance is clearly a robust adaptive immune function because even healthy adult subjects absorb small amounts of intact food antigens, particularly after meals—corresponding to 10-5 of the intake and reaching a circulating level of 3–10 ng/ml [23]—as part of the total daily protein uptake of 130–190 g [24]. The epithelial tightness and the immunoregulatory network remain fragile for a variable period after birth [25, 26]. Importantly, animal experiments show that the postnatal development of mucosal homeostasis depends on the establishment of a balanced commensal microbiota as well as on adequate timing and dosing of foreign dietary antigens when first introduced [7, 10, 26, 27].
Inductive Gut-Associated Lymphoid Tissue Structures
It follows from the preceding sections that immune cells are located in three intestinal compartments: GALT, the mucosal lamina propria, and the surface epithelium (Figs. 2.2 and 2.3). GALT structures represent inductive sites for immune responses, while the lamina propria and epithelial compartment principally constitute effector sites but may nevertheless contribute to retention, proliferation, and differentiation of immune cells [2, 28].



Fig. 2.2
Development of the intestinal immune system. Inherent and environmental signals drive the mucosal changes observed both in mice and humans; the postnatal establishment of an increasingly complex and dense gut microbiota is a decisive variable. The lymphoid structures of Peyer’s patches and mesenteric lymph nodes are generated before birth but mature during the postnatal period. By contrast, cryptopatches (seen clearly only in mice) and isolated lymphoid follicles (ILFs) are formed after birth. Specialized antigen-sampling epithelial cells, known as M cells, reside above Peyer’s patches and ILFs and facilitate antigen transport from the gut lumen to the underlying lymphoid cells. Simultaneously, innate lymphocytes, such as lymphoid tissue inducer (LTi) cells, as well as adaptive T cells leave the liver and thymus, respectively, and colonize the mucosa, including the epithelium. Intraepithelial lymphocytes (IELs) reside in close proximity to epithelial cells. Also, increasing numbers of CD103+ dendritic cells and CX3CR1+ macrophage-like cells home to the gut mucosa. In contrast to innate lymphocytes, regulatory T (Treg) cells populate the intestinal mucosa in response to bacterial colonization. Although B cells are present in gut tissue during early development, plasma cells producing dimeric IgA are only generated after birth to provide secretory IgA (SIgA) which is transported to the lumen by the polymeric Ig receptor (pIgR). Maternal SIgA is provided by breast milk during the early postnatal period. Modified from Renz et al. [29]

Fig. 2.3
Depiction of the intestinal mucosal immune system. Inductive sites for T and B cells are constituted by gut-associated lymphoid tissue (GALT ) such as Peyer’s patches with B-cell follicles and M cell (M)-containing follicle-associated epithelium through which exogenous antigens (Ag) are transported to reach antigen-presenting cells (APC), including dendritic cells, macrophages (Mφ), and follicular dendritic cells (FDC). After being primed, naïve T and B cells become memory/effector cells and migrate from GALT to mesenteric lymph nodes via lymph and then via the thoracic duct to peripheral blood for subsequent extravasation at mucosal effector sites. This process is directed by the profile of adhesion molecules and chemokines expressed on the local microvasculature—the endothelial cells thus exerting a “gatekeeper function” for mucosal immunity (see Figs. 2.3 and 2.5). The lamina propria (effector site) is illustrated with its various immune cells, including B cells (B), the approximate proportions of various Ig-producing plasma cells, and CD4+ T cells. The distribution of intraepithelial T lymphocytes (mainly CD8+ with α/β T-cell receptor; some γ/δ) is also depicted. Additional features are the generation of secretory IgA (SIgA) and secretory IgM (SIgM) via pIgR/membrane secretory component (mSC)-mediated epithelial export. The combined effect of oral tolerance mechanisms, mainly the action of regulatory T (Treg) cells, provides a suppressive tone in the gut, normally keeping inflammation driven by IgG and IgE antibodies as well as cell-mediated (CD4+ T cell and Mφ) delayed type hypersensitivity (DTH) under control
The lymphoid structures of Peyer’s patches and the draining mesenteric lymph nodes (MLNs) are formed before birth with discrete T- and B-cell areas being apparent after 19 weeks’ gestation in humans [25]; but the size of these structures and germinal centers (GCs) of B-cell follicles depend on the postnatal microbial colonization. Also, ILFs cannot be observed until after birth. Cryptopatches are seen only in mice, perhaps depending on the age at tissue sampling, and these structures are believed to develop into ILFs [29] (Fig. 2.2). B cells of GCs in GALT express mainly surface IgA as a result of Ig heavy-chain gene switching in the course of B-cell differentiation to IgA-producing plasmablasts. Notably, IgA induction is much more prominent in GALT than in other structures of mucosa-associated lymphoid tissue (MALT) [2, 28].
It takes some time after birth before the Peyer’s patches become activated as signified by GCs, and the induction of ILF organogenesis from cryptopatches as seen in mice depends on postnatal exogenous stimuli [2, 30]. The B-cell follicles of GALT are covered by a specialized follicle-associated epithelium (FAE) containing very thin “microfold” or “membrane” (M) cells which, together with intraepithelial dendritic cells (DCs), transport antigens from the gut lumen into the lymphoid tissue [31].
Peyer’s patches are defined as at least five aggregated B-cell follicles. These structures resemble lymph nodes with inter-follicular T-cell zones and a variety of antigen-presenting cells (APCs) such as DCs and macrophages, whereas ILFs have a sparser T-cell zone. All GALT structures are devoid of encapsulation and contain no afferent lymphatics [2, 5]; their supply of antigens depends exclusively on sampling directly from the mucosal surface through the FAE. Induction and regulation of mucosal immunity hence takes place primarily in GALT and mucosa-draining lymph nodes, while terminal differentiation of B cells to PCs occurs in the lamina propria (Figs. 2.2 and 2.3) where secondary T-cell signals are generated when antigens are presented by local DCs and macrophages [2, 28]. However, animal experiments have shown that oral tolerance can be induced in the absence of GALT, thus being dependent on antigen transport to mesenteric lymph nodes (MLNs) from gut mucosa through lymph by specialized DCs, as discussed later [32, 33].
Activation and Homing of Intestinal B Cells
Antigens are presented to T cells in GALT and draining lymph nodes by APCs after intracellular processing. The activated helper T (Th) cells release mediators (cytokines), and especially transforming growth factor (TGF)-β induces the switch of B cells from surface membrane expression of IgM to IgA in GCs of GALT [2, 28]. Memory/effector cells migrate rapidly via lymphatics to MLNs where B cells may be further differentiated to plasmablasts, which then reach peripheral blood via the thoracic duct and finally become seeded into secretory effector sites (Fig. 2.3). This homing targets particularly the intestinal lamina propria but to some extent also distant secretory mucosae and glandular sites—notably the lactating mammary glands [2, 34].
The extravasation of plasmablasts at effector sites is facilitated by compartmentalized homing receptors interacting with ligands (addressins) on the microvascular endothelium, while additional fine-tuned navigation is conducted by chemokines [1, 2]. The extent of B-cell retention and terminal differentiation to PCs in the gut lamina propria (Fig. 2.3) depends on the local intensity of second signals, provided by chemokines and APC-processed antigens via activated CD4+ Th cells and their cytokines [2, 28].
Retinoic acid (RA) derived from vitamin A exerts a positive impact both on intestinal differentiation of naïve B cells arriving in GALT and their subsequent migration as precursors for the mucosal IgA-producing PCs [2, 35]. Thus, the heavy chain switching to IgA both in humans and mice is enhanced by RA, and so is the expression of the gut-homing molecules integrin α4β7 and the CC chemokine receptor CCR9 (Figs. 2.4 and 2.5). The phenotype of APCs in GALT, which seems to be imprinted by the action of gut bacteria on cellular PRRs, promotes RA generation by the expression of retinaldehyde dehydrogenase (RALDH); and the expression of inducible nitric oxide synthase (iNOS) enhances, via nitric oxide (NO), the release of innate switch factors which are cytokine members of the tumor necrosis factor (TNF) family, namely APRIL (A Proliferation Inducing Ligand) and BAFF/BlyS (B cell-activating factor of the TNF family/B lymphocyte stimulator), as well as the release of the activated IgA switch factor TGF-β from Th cells [2]. Furthermore, it has been reported that in mice a fraction for these follicular T helper (TFH) cells may be derived from regulatory T (Treg) cells [36]. There may thus be a cellular link between intestinal IgA and mucosal tolerance induction, for which TGF-β is likewise important. Also interleukin (IL)-10 that contributes to terminal differentiation of IgA+ PCs together with IL-6 (Fig. 2.4) is an important cytokine in oral tolerance, as discussed later.



Fig. 2.4
Induction of IgA switch in mucosal B cells (B) and imprinting of their gut-homing molecules α4β7 and CCR9 occurs in gut-associated lymphoid tissue (GALT) and mesenteric lymph nodes (not shown). As described in the text, antigen-presenting cells (APC/DC) in GALT are, through their pattern recognition receptors, activated by commensal bacteria and express iNOS and RALDH. The latter enzyme converts vitamin A from the diet to retinoic acid (RA) which stimulates expression of the heterodimeric integrin α4β7 and the chemokine receptor CCR9—attracting the B cells to their ligands in the small intestinal lamina propria (see Fig. 2.5). The level of α4β7 is particularly high on lymphoblasts, and the B-cell adherence to microvascular endothelium is strengthened by interactions between generalized adhesion molecules such as LFA-1 and ICAM-1/ICAM-2, as indicated. Also the switching from IgM to IgA expression is enhanced by RA in B cells expressing activation-induced cytidine deaminase (AID), and this process is stimulated by follicular helper T (TFH) cells which may be derived from RORγt+ Th17 cells or from Foxp3+ regulatory T (Treg) cells. Cytokines promoting the IgA development are framed: TGF-β is a switch factor and nitric oxide (NO) may contribute to its activation; IgA-inducing protein (IGIP) is another switch factor whose expression in DCs may be stimulated by vasoactive intestinal polypeptide (VIP); and IL-6 and IL-10 stimulate terminal differentiation to IgA-producing plasma cells. The T cell-independent switch factors APRIL and BAFF are also expressed in GALT, as indicated

Fig. 2.5
Depiction of homing mechanisms that attract gut-associated lymphoid tissue (GALT)-derived B and T memory/effector cells to the small intestinal lamina propria (to the right; see Figs. 2.2 and 2.3). Interactions between the multidomain unmodified (containing no l-selectin-binding O-linked carbohydrates) mucosal addressin cell adhesion molecule (MAdCAM)-1 expressed on ordinary flat lamina propria venules is important as part of the endothelial “gatekeeper function” to direct mucosal α4β7-bearing memory/effector B and T cells to the normal gut mucosa (solid arrows). Selectively produced by the epithelium of the small intestine, the chemokine TECK (CCL25) attracts GALT-derived B and T cells expressing CCR9 to this segment of the gut, whereas MEC (CCL28) is a more generalized chemokine interacting with CCR10 on mucosal B cells. A GALT structure with its M cells (M), antigen-presenting cells (APC) such as macrophages (Mφ) and follicular dendritic cells (FDC), is depicted on the left. The bottom left panel shows immunohistology of a Peyer’s patch with lymphoid follicles containing germinal centers (GC); the insert shows that MAdCAM-1 (with l-selectin-binding capacity) is expressed (brown color) on high endothelial venules (HEV) to attract naïve lymphocytes for priming. The access to the mucosal effector site is normally limited (broken arrows) for circulating proinflammatory cells such as monocyte (Mo)-derived Mφ, polymorphonuclear neutrophils (PMN), eosinophils (Eos) and mast-cell (MC) precursors, whereas the favored GALT-derived B and T cells promote mucosal immunity including polymeric Ig receptor (pIgR)-dependent secretory IgA (SIgA) generation. Mo-derived mucosal dendritic cell (DC) may develop through a transitional Mφ phenotype, as indicated. Right bottom panel shows paired immunofluorescence staining for IgA- and IgG-producing plasma cells (see color key) in normal colonic human mucosa and crypts with selective transport of IgA to the lumen. Note the negatively stained goblet cells
However, a more recent study was unable to reproduce these findings and showed, instead, that the TFH cells were derived from Th17 cells [37]. It has been speculated that this disparity might be explained by differences in gut microbiota of the experimental mice [38]. In addition, a proper function of the GCs depends on the presence of follicular Treg (TFR) cells, controlling the proliferative activity of the B cells and the quality of the IgA produced [38].
The long-lasting debate about the role of vasoactive intestinal polypeptide (VIP) in IgA induction seems to be clarified (Fig. 2.4). An IgA-inducing protein (IGIP), first identified in the bovine species, has also been characterized in humans—with somewhat different properties [39]; its CD40L-stimulated expression in DCs was found to be 35-fold enhanced by VIP, and IGIP was directly shown to induce CSR to IgA in naïve (IgM+IgD+) B cells.
However, a problem with all the experimental studies on IgA-promoting factors operating in GALT, such as TGF-β, RA and IGIP, is that they have not been tested for J chain-inducing properties. This small polypeptide is a prerequisite for the production of dimeric IgA and its binding to the epithelial polymeric Ig receptor (pIgR), as discussed later. Although there is considerable information about the regulation of J-chain expression in mice, such knowledge is lacking in humans; and the factors responsible for the high level of J chain in GALT-derived B cells are not known in any species [2, 40].
Regional Intestinal Immune Differences
The effect of the indigenous bacteria on the postnatal development of GALT and intestinal PCs is strikingly revealed in experimental animals colonized with a conventional microbiota after being reared in a germ-free state [41, 42]. Also food proteins contribute [2], as for instance observed in mice reared on a diet containing casein compared with a balanced amino acid-based diet [43]. Whereas MAMPs and small chain fatty acids (SCFAs) such as butyrate from the gut microbiota are abundant as stimulatory factors in the distal gut, this is not the case in the upper small intestine (Fig. 2.6). It may therefore seem surprising that the number of PCs per intestinal length unit is virtually the same in the duodenum as in the colon, and there is a higher proportion of the IgM phenotype in the former (Fig. 2.6). The reason may be the impact of high levels of food antigens and retinoids from bile and vitamin A in the diet [18], and also an involvement of the celiac lymph node complex in the immune induction in that region.


Fig. 2.6
Histomorphometric estimation of the distribution of plasmablast/plasma cell (PC) phenotypes in healthy human intestinal mucosa. A “tissue unit” is defined in a 6-μm thick section prepared for immunohistochemical analysis. The included lamina propria area varies among different specimens depending on the height of the tissue unit; and the total number of PCs per unit is determined by this variable as well as by the actual tissue density of such cells. The pie charts depict the average percentage and numerical distribution of PCs in various segments of the gut, with median numbers (and ranges) per mucosal tissue unit indicated. All units are 500 μm wide (vertical axis), and the median height (horizontal axis) for each specimen category is shown (n = number of subjects). Based on published data from the author’s laboratory; see Fig. 20 in Brandtzaeg [2]. The right part of the figure is modified from Fig. 2 in Mowat and Agace [18]
The propensity of the mucosal immune system to generate low-affinity cross-reactive antibodies, at least in the distal gut, is probably explained by the extensive innate drive exerted by MAMPs derived from the abundant indigenous microbiota interacting with PRRs, as alluded to above. Thus, experiments have revealed a role of the important PRRs designated Toll-like receptors (TLRs) for B-cell differentiation in GALT [2, 5]. Interestingly, human GALT follicles contain the apparatus to support both T cell-dependent and T cell-independent (not involving CD40–CD40L interactions) class switch recombination (CSR) pathways to IgA (Fig. 2.4). This has been documented by showing GALT-restricted human expression of activation-induced cytidine deaminase (AID)—an essential enzyme for CSR to take place [44, 45]. Also notable, the T cell-independent switch factor APRIL, and its receptors TACI (Transmembrane Activator and CAMEL Interaction) and BCMA (B Cell Maturation Antigen) are expressed both in human GALT and human intestinal mucosa but, importantly, there is no co-expression of these receptors and AID beyond the GALT structures [44].
Thus, previous claims about B-cell switch to IgA in human colonic lamina propria appear questionable, and the same is true for the proposed extrafollicular switch from IgA1 to IgA2 [46]. A recent study showed that if these events occur outside of GALT structures, their biological importance must be negligible [47]. The possibility remains, however, that the unique T cell-independent B1 cell population generated in the mouse omentum [48] may provide a substantial fraction of lamina propria PCs and be subjected to IgA switch either in the peritoneal cavity or in the lamina propria of this species [49, 50]. However, other studies suggest that T cell-independent CSR in the mouse gut does depend on GALT structures although not on GCs [51]. Thus, a likely possibility is that T cell-independent switch to IgA in the mouse mainly takes place in the numerous ILFs present in the distal gut [2, 5, 52], and that APRIL outside of GALT structures both in mice and humans mainly promotes the survival of PCs in the mucosa, similarly to the role of BAFF in the bone marrow [53].
Intestinal Immunity in Infancy
In parallel with the bacterial colonization, the homing of lymphocytes (including IgA+ plasmablasts) to the gut lamina propria seems to follow a defined kinetics—apparently reflecting a series of endogenous and exogenous signals regulating intestinal postnatal immune maturation (Fig. 2.2). Innate cells—such as lymphoid tissue inducer (LTi) cells, natural killer (NK) cells, NK-like NKp46+ cells, and T helper 2 (Th2)-like cells—migrate during the first 4 weeks after birth from the murine fetal liver to the gut mucosa driven by endogenous signals [29]. By contrast, enhanced recruitment of CD8+ intraepithelial lymphocytes (IELs) and forkhead box protein 3 (Foxp3)+ Treg cells to the rodent gut, and also the production of anti-inflammatory IL-10, have been associated with the establishment of the intestinal microbiota [29, 54]. The Treg cells help to keep proinflammatory CD4+ Th cells and cytotoxic CD8+ T cells under control to preserve the epithelial barrier [55].
The delay of the postnatal mucosal immune activation parallels a temporary immaturity of systemic immunity [25]. Very few plasmablasts occur in peripheral blood of newborns [56], but after 1 month those with IgA-producing capacity (presumably GALT-derived) are remarkably increased [57], signifying progressive microbial stimulation. Thus, the number of IgA+ plasmablasts in the blood of newborns is <8 per million mononuclear cells, but is increased to ~600 per million mononuclear cells already after 1 month, apparently reflecting the progressive microbial stimulation of GALT [57]. An initial early elevation of circulating plasmablasts (mainly IgM+) occurs in preterm infants, especially those with intrauterine infections [56]. Thus, mucosal immune cells are competent at least during the final trimester, but APCs need to be activated by microbial factors that enable them to provide appropriate co-stimulatory signals to naïve T cells [58]. The commensals are important to this end as shown by the fact that the number of intestinal IgA+ PCs is normalized 4 weeks after exposure of germ-free mice to a conventional complex gut microbiota [59, 60]. Bacteroides and Escherichia coli strains seem to be particularly immunostimulatory, but also lactic acid-producing bacteria contribute [61, 62]. A study showed that an optimal stimulatory effect requires a host-specific microbiota, and the same holds true for small-intestinal T-cell activity [63].
In agreement with these observations, only scattered IgM+ (and IgG+) intestinal PCs could be seen in newborns, and IgA+ cells were either absent or extremely rare even at 10 days of age [25]. The numbers of IgM- and IgA-producing cells increased rapidly after 2–4 weeks—the latter becoming predominant at 1–2 months, usually peaking around 12 months. However, in affluent societies it may take several years for the size of the IgA+ PC population to reach that of healthy adults, whereas a fast postnatal increase of SIgA was observed in children living in developing countries with a heavy microbial load [55].
The retarded postnatal activation of GALT parallels the functionally decreased systemic immunocompetence in the newborn period [25, 26, 58, 64]. Thus, peripheral CD4+ Th cells of infants show reduced capacity for cytokine production and B-cell help. One reason may be that there are relatively few circulating memory (CD45R0+) T cells in infancy, although the responsiveness of neonatal naïve (CD45RA+) T cells does not differ significantly from that of virgin counterparts in adults. Indeed, the chief explanation for the immunological immaturity in infancy appears to be a deficient APC function [65]. Interestingly, pioneering studies in mice showed that the microbiota stimulates a self-limiting intestinal SIgA response [66]. Such transient SIgA production is probably necessary to allow access of microbial constituents to GALT. In this manner, it seems that the intestinal IgA response is continuously adapting to the changing microbiota [67], which would be especially relevant in the early postnatal period.
Secretory Immunity and the Epithelial Barrier Function
Formation and Properties of Secretory Antibodies
Most mucosal PCs produce dimers and larger polymers of IgA (collectively called pIgA), which contain a disulfide-linked 15-kDa polypeptide called the “joining” or J chain (Fig. 2.7). The J chain is a prerequisite for active dimerization and the export of pIgA through secretory epithelia such as the intestinal crypts [68, 69]. This transport is mediated by a ~100-kDa glycoprotein called pIgR, which is also known as membrane secretory component (SC) [70]. J chain-containing pentameric IgM is externally transported by the same mechanism (Figs. 2.3 and 2.7) [68, 71], and this peptide is part of the binding site for pIgR/SC independent of its involvement in the polymerization process [72].


Fig. 2.7
Receptor-mediated export of dimeric IgA and pentameric IgM to provide secretory antibodies (SIgA and SIgM) functioning in immune exclusion of antigen (Ag) at the mucosal surface. Polymeric Ig receptor (pIgR) is expressed basolaterally as membrane secretory component (mSC) on mucosal epithelial cells and mediates transcytosis of dimeric IgA and pentameric IgM, which are produced locally with incorporated J chain (IgA + J and IgM + J) by local plasma cells. Although J chain is often expressed by mucosal IgG plasma cells (70–90 %), it does not combine with this isotype and is therefore degraded intracellularly as denoted (±J). Locally produced (and serum-derived) IgG is therefore not subject to pIgR-mediated transport, but can be transmitted paracellularly to the lumen together with monomeric IgA, as indicated. Free SC (depicted in mucus) is generated when pIgR in its unoccupied state (top basolateral symbol) is cleaved at the apical face of the epithelium like bound SC in SIgA and SIgM. Commensal bacteria in the right-hand panel are coated in vivo with SIgA, which aids their containment and thereby promotes microbial–host mutualism. Immunofluorescence illustration from Brandtzaeg et al. [161]
Apical proteolytic cleavage of the extracellular portion of pIgR enables release of SIgA and secretory IgM (SIgM) to the lumen. In this manner the ectodomain of pIgR (~80 kDa) is “sacrificed” to become bound SC which stabilizes the quaternary structure of the secretory antibodies, particularly SIgA where a disulfide bridge is formed between the two partners [28]. Unoccupied pIgR (some 50 %) is constitutively exported in the same manner; the cleaved ectodomain is then called free SC [70] and exhibits certain innate immune properties such as affinity for E. coli and Clostridium difficile toxin [3]. Bound SC confers such properties to SIgA and contributes to its mucus-binding capacity and its property of trapping bacteria in biofilms [73].
Immune exclusion performed by SIgA and SIgM thus depends on an intimate cooperation between the mucosal B-cell system and the pIgR-expressing epithelium (Fig. 2.7). Serum-derived and locally produced IgG antibodies may also to some extent contribute to immune exclusion when reaching the lumen by noninjurious paracellular diffusion [74] or after being transported by the neonatal Fc receptor, FcRn [75]. However, IgG is rapidly degraded in the gut lumen, although the hepatic super antigen (protein Fv) may form large complexes with degraded antibodies of different specificities, thereby reinforcing their immune exclusion function [76].
As alluded to above, only smaller amounts of pentameric IgM are normally exported by the pIgR, and SIgM is not as stable as SIgA because bound SC is covalently stabilized only in the latter [74]. Immune exclusion is therefore normally performed mainly by SIgA (Fig. 2.3) in cooperation with innate defenses such as mucus, defensins, and peristalsis [55]. In newborns and subjects with selective IgA deficiency, however, SIgM antibodies are of greater importance than in healthy adults [25, 28]. Thus, while IgA is generally undetectable in the mucosa before 10 days of age, IgM+ PCs may remain predominant up to 1 month. Thereafter a rapid expansion of IgA+ PCs takes place, and some increase may be seen up to 1 year of age (Fig. 2.8). The epithelial production of pIgR/SC, however, begins in fetal human life around 3–5 months and is constitutively regulated [25]; its expression increases steadily until birth and may be followed by a postnatal peak (Fig. 2.8), which could reflect the microbial encounter. Accordingly, traces of SIgA and SIgM occur in intestinal fluid during the first postnatal period, and some IgG is often present—mainly reflecting passive transmission from the lamina propria which after 34 weeks of gestation contains readily detectable maternal IgG [25].


Fig. 2.8
Maturation of the intestinal immune system and the epithelial barrier. The production of the human polymeric Ig receptor (pIgR) begins in fetal life at 3–5 weeks gestation, but the plasma cells producing its ligand dimeric IgA are hardly presence before birth. The murine neonatal mucosa is characterized by little epithelial cell proliferation, absence of developed crypts (intestinal glands) and crypt-based Paneth cells, but marked expression of cathelicidin-related antimicrobial peptide (CRAMP); by contrast, the formation of intestinal crypts late during the second week after birth in mice initiates increased proliferation and rapid epithelial cell renewal, generation of α-defensin-producing Paneth cells, and upregulation of the antibacterial C-type lectin regenerating islet-derived protein 3γ (REG3γ). A decrease in the epithelial expression of Toll-like receptor 4 (TLR4) before birth, and a steady increase in the level of the nuclear factor-kB inhibitor IkBα during the postnatal period reduce the responsiveness to bacterial lipopolysaccharide and other pro-inflammatory stimuli. Such acquisition of epithelial TLR tolerance creates a neonatal period of decreased innate immune responsiveness. Note that the small intestinal epithelium at birth has a more mature phenotype in humans than in mice. Modified from Renz et al. [29]
A much faster establishment of SIgA immunity can be seen in developing countries with a heavy microbial load [77]. Some reports suggest that also probiotic treatment enhances the production of IgA, but this was not confirmed by measuring IgA in saliva [78]. Nevertheless, after a combination of prebiotic and probiotic treatment given perinatally and for the first 6 months of life, infants that showed an early elevation of fecal IgA had reduced risk of allergies before 2 years of age [79]. Also, in another study it was shown that early intestinal colonization with Bifidobacterium species was associated with significantly elevated levels of SIgA in saliva at 6 months of age [80].
The Epithelial Barrier Function
The neonatal gut varies among species in terms of maturity, depending in part on the length of the gestation period. The small intestinal mucosa of newborn humans has a mature crypt–villous architecture, with continuous stem cell proliferation, and epithelial cell migration and differentiation. In mice, small intestinal crypts only develop 10–12 days after birth, accompanied by increased epithelial cell renewal and transcriptional reprogramming of enterocytes, which includes changed expression of genes involved in nutrient transport, metabolism, and cell differentiation [29]. Also, the enteric spectrum of antimicrobial peptides changes significantly during neonatal development in mice (Fig. 2.8). During the first 2 weeks of postnatal life, when mature crypt-based Paneth cells are absent, the mouse intestinal epithelium expresses cathelicidin-related antimicrobial peptide, or CRAMP. The Paneth cells start to produce defensins at weaning, and then the CRAMP expression decreases.
While defensin production by Paneth cells is independent on bacterial colonization, expression of other antimicrobial peptides by epithelial cells—such as the C-type lectin regenerating islet-derived protein 3γ (REG3γ)—requires a microbiota (Fig. 2.8) and is supported by IL-22-producing RORγt+NKp46+ lymphocytes [29]. Thus, administration to mice of broad-spectrum antibiotics by gavage has been shown to reduce significantly the colonic epithelial expression of 70 genes; and five of the seven genes that were more than fourfold less active than normal, encoded antimicrobial peptides including Ang4, Pla2g2a, Retnlb, REG3γ, and REG3β [55]. REG3γ has furthermore been detected in murine γδ IELs in response to microbial stimulation of epithelial cell-intrinsic adapter protein MyD88 signaling, probably mediated through TLRs [81, 82]. Also human γδ IELs seem to belong to the first-line innate mucosal defense as deemed from their behavior in AIDS patients and lack of response to antiretroviral treatmen t [83].
Although the so-called “gut closure ” normally occurs in humans mainly before birth, the mucosal barrier may be inadequate up to 2 years of age; the mechanisms involved remain poorly defined [84], but the development of secretory immunity is probably one decisive variable. Importantly in this context, pIgR-deficient knockout mice that lack SIgA and SIgM exhibit aberrant mucosal leakiness [85] and have increased uptake of food proteins and commensal bacteria [86]. A thick virtually microbe-devoid inner mucus layer has been revealed at the apical epithelial surface of the normal colon [87]; this protected zone apparently limits direct bacterial contact with host cells, but it may nevertheless be permeable for MAMPs and antigens derived from the abundant microbiota present in the outer mucus layer—particularly when SIgA is absent (see later).
Mice lacking pIgR (knockout mice) show significantly elevated production of IgG antibodies to commensal bacterial antigens but, interestingly, not to food proteins [85, 86]. The undue influx of microbial products causes a generalized hyperreactive state with overactivation of the innate cellular NF-kB transcription pathway, resulting in 50 % liability of these mice to anaphylactic death after systemic antigen sensitization (ovalbumin, OVA) and low-dose intradermal challenge [88]. However, the pIgR-deficient mice exhibit enhanced capacity for induction of oral tolerance, which after OVA feeding was fully able to control IgG1- and T cell-dependent hypersensitivity against the same antigen (Fig. 2.9). This observation might imply that at the same time as an inadequate intestinal barrier in the infant represents a risk for hypersensitivity reactions, it will promote tolerance against cognate antigens when they are continuously present in the gut [12].


Fig. 2.9
Depiction of experimental model for hypersensitivity and oral tolerance. Lack of secretory antibodies (SIgA and SIgM) in pIgR knockout mice leads to inadequate immune exclusion of bacterial components from the gut microbiota. Such conserved microbe-associated molecular patterns (MAMPs), e.g., lipopolysaccharide (LPS), will interact with pattern recognition receptors (PRRs) on innate immune cells, including macrophages (Mφs), which become hyperreactive. These cells are therefore sensitive to IgG-containing immune complexes interacting with Fc receptors (FcγRIII), which renders the mice predisposed to hypersensitivity (anaphylaxis). The deficient epithelial barrier also allows increased uptake of food antigens from the gut lumen (e.g., fed ovalbumin) which enhances induction of oral tolerance, providing a net anti-inflammatory effect against undue penetration of the same antigen into the body by any route (e.g., dermal). Production of IgG antibodies against this sensitizing antigen will thus be downregulated, and the animal is protected against anaphylaxis and delayed hypersensitivity (not shown) after antigen challenge. Adapted from Karlsson et al. [88]
The postnatal balance between the epithelial barrier function and oral tolerance thus appears to be critical for the induction of immunological homeostasis . Notably, although the incidence of food allergy (apparently non-IgE-mediated) is increased in children with IgA deficiency, it is not strikingly elevated [89]—perhaps because the induction of Treg cells is enhanced in addition to compensatory SIgM, which in such individuals partially replaces the lacking SIgA in the gut [31, 90]. Also, bacterial overgrowth occurs in the jejunum of vagotomized patients only when IgA deficiency is combined with suboptimal function of innate defenses such as gastric acid and peristalsis [91]. In this context, it is notable that the frequency of selective IgA deficiency among IBD patients in Sweden is significantly increased, especially for those with Crohn’s disease (prevalence ratio, 5.7, compared with 3.9 in ulcerative colitis) [92].
AID knockout mice that have SIgA deficiency due to lack of Ig class switching, and also exhibit a defect IgM antibody response, show massive intestinal overgrowth of commensal anaerobic bacteria with a resulting striking hypertrophy of ILFs over time [93]. This development has some resemblance to the irregular lymphoid aggregates seen in long-standing IBD [94]. In contrast to AID and pIgR knockout mice, however, IgA-deficient mice (like IgA-deficient humans) have compensatory SIgM antibodies in their gut lumen [95]. Interestingly, these mice show no increased susceptibility to various gut infections or to dextran sulfate sodium (DSS)-induced colitis, whereas pIgR deficient mice do—probably because they lack both SIgA and SIgM [95, 96].
It has been suggested that postnatal hyperreactivity of the immune system may also result from intrauterine events, probably causing genetically or epigenetically determined poor Treg-cell function and immunological immaturity [97, 98], as also suggested from cord blood studies [99]. In normal mice it has been shown that neonatal (but not adult) CD4+ T cells are strikingly prone to differentiate into Treg cells upon stimulation [100]. Moreover, the intestinal epithelium itself is equipped with a vast array of features to control immune barrier homeostasis (Fig. 2.8) [101]. These key intrinsic mechanisms have been reviewed and include variables such as secretion of mucins and defensins, inflammasome function, intercellular junctional complex regulation, and PRR signaling [102]. As discussed in a subsequent section, the latter is downregulated in the neonatal gut epithelium to preserve its integrity upon the encounter with the commensal microbiota [103, 104].
Additional IgA Antibody Function s
Antibody production by the numerous intestinal pIgA+ PCs may also be important for homeostasis within the lamina propria as a result of several anti-inflammatory mechanisms. IgA lacks ordinary complement-activating properties [105] and can therefore block nonspecific biological amplification triggered by locally produced or serum-derived IgG antibodies (Fig. 2.10), which may actually increase the penetration of exogenous bystander antigens through the surface epithelium [106]. This is important in view of the fact that immune complexes are probably formed even within the normal lamina propria due to some influx of soluble antigens, particularly following food intake [23]. Also, in vitro and in vivo experiments have suggested that soluble antigens—after pIgA-mediated noninflammatory trapping in immune complexes—may be cleared by the secretory epithelium via pIgR-mediated translocation to the lumen (Fig. 2.10) [3, 4, 107]. Similar experiments have suggested that pIgA antibodies can neutralize lipopolysaccharide (LPS) and viruses within secretory epithelial cells during pIgR-mediated export [3, 4], and thus return harmful microbial components to the gut lumen (Fig. 2.11). Mouse models have confirmed that the latter mechanism contributes to the intestinal defense against rotavirus infection [108], and IgA+ PCs with specificity for rotavirus occur in normal jejunal mucosa of adults with no signs of current infection [109].



Fig. 2.10
Mucosal homeostasis in the normal gut . Contributing variables are presented as a balance between Ig classes (for simplicity, only IgA and IgG are indicated) and regulatory T (Treg) cells. Secretory IgA (SIgA) is generated from dimeric IgA (with associated J chain) produced by local plasma cells and transported to the lumen by the polymeric Ig receptor (pIgR), also called membrane secretory component (mSC). After apical cleavage, unoccupied receptor is translocated in the same manner and called free SC (f-SC), in contrast to the bound SC in SIgA. The secretory antibodies act in first-line defense by performing antigen exclusion at the mucosal surface (to the right). Antigens penetrating the epithelial barrier may meet serum-derived IgG antibodies in the lamina propria. The formed immune complexes can activate complement, and the resulting inflammatory mediators may cause temporarily increased paracellular leakage of IgG antibodies (broken arrow). Sustained inflammation is normally inhibited by blocking antibody activities in the lamina propria exerted by serum-derived or locally produced IgA (competition for antigen depicted) and anti-inflammatory Treg cells. Independent of antibody specificity, IgA-containing immune complexes may also inhibit pro-inflammatory mediator release (TNF-α depicted) from activated phagocytic cells such as macrophages (Mφ). Moreover, antigens bound to dimeric IgA may be returned in a non-inflammatory manner to the gut lumen by the pIgR-mediated transport mechanism, as indicated

Fig. 2.11
Different principles for how secretory antibodies (SIgA and SIgM) may contribute to mucosal homeostasis . In addition to immune exclusion, the pIgR-mediated external transport of dimeric IgA and pentameric IgM (pIgA/IgM) may be exploited for intraepithelial virus and toxin neutralization, as well as non-inflammatory antigen (Ag) excretion from the lamina propria (see Fig. 2.11). However, when infection with invasion occurs, systemic immunity takes over; this involves proinflammatory mechanisms such as activation of complement (Ċ) by IgG antibodies, cell-mediated immunity (CMI), and cytotoxicity—all of which may cause tissue damage. Research forming the basis for the depicted mechanisms is reviewed in more detail elsewhere [4, 5, 40]
The mucosal clearance function exhibited by pIgA (and probably pentameric IgM) antibodies reinforces the immune exclusion mediated by SIgA (and SIgM) with innate-like low-affinity antibody activity generated against commensal bacteria [20, 101], and which to some extent may be cross-reactive (Fig. 2.11). The high-affinity antibodies induced by pathogens or properly adjuvanted oral vaccines may even more efficiently contribute to both immune exclusion and mucosal clearance, as reviewed elsewhere [4].
Locally produced pIgA may further influence homeostasis by interacting with the Fcα receptor (FcαRI, CD89) on leukocytes in the lamina propria. First, on the one hand it has been shown that pIgA-containing immune complexes are able to suppress attraction of neutrophils, eosinophils, and monocytes, thereby reducing their proinflammatory activities. On the other hand, when such complexes interact with CD89 on neutrophils, leukotriene B4 is released as a chemotactic factor attracting more of these cells [111]. Second, IgA can apparently downregulate the secretion of proinflammatory cytokines such as TNF-α from activated monocytes [112]. However, it is uncertain whether this mechanism operates in the normal gut (Fig. 2.10) because mucosal macrophages do not express detectable surface CD89—at least not in the small intestine [113, 114]. Third, neutrophil and monocyte activation that results in generation of reactive oxygen metabolites (“respiratory burst”) is reportedly inhibited by IgA [115]. Conversely, pIgA may temporarily trigger monocytes to enhanced activity—including TNF-α secretion [116]—and IgA (particularly SIgA) appears to be a potent activator of eosinophils [117, 118]. Indeed, complexed SIgA induces respiratory burst and degranulation of these cells, while soluble SIgA enhances their survival in vitro [119].
Thus, cross-linking of CD89 during infection with IgA-opsonized pathogens may cause proinflammatory responses, whereas naturally occurring IgA (not complexed) may induce inhibitory signals through CD89, thereby damaging excessive reactions [120]. Together, these results suggest that the participation of pIgA in mucosal homeostasis is quite fine-tuned [121]—perhaps being skewed towards a proinflammatory potential in IBD where there are numerous granulocytes [111] along with recently recruited monocyte-like macrophages which express of the LPS co-receptor CD14 and PRRs such as TLR2 and TLR4 [122, 123].
Initially, the shift from the normal predominance of mucosal pIgA production to IgG and monomeric IgA in IBD lesions [124] may represent a powerful second line of defense because these antibodies may efficiently mediate immune elimination of penetrating bacteria via phagocytosis and antibody-dependent cell-mediated cytotoxicity (Fig. 2.11). Notably, by means of CD89 both neutrophils [111] and liver Kupffer cells [125] may phagocytose translocated gut bacteria opsonized even with serum-type monomeric IgA as alluded to above. Rodent studies suggest that the liver is designed to handle this antigen elimination in a silent manner because of its unique tolerogenic capacity, including induction of Treg cells by plasmacytoid DCs [126]. Antigen elimination within the intestinal lamina propria, however, may more readily cause inflammation and tissue damage when systemic type of immunity is involved; this is a risk the immune system sometimes must take to hinder sepsis and save life (Fig. 2.11). The role of mucosal IgA and oral tolerance is to counteract overactivation of potentially harmful immune reactions.
Effect of Microbial–Host Interactions on Innate and Adaptive Immunity
The Epithelial Barrier and Secretory Immunit y
In fetal life, murine gut epithelial cells are sensitive to microbial factors such as LPS (endotoxin) because they highly express intracellularly a PRR for this MAMP, namely TLR4 (Fig. 2.8) [103]. Exposure to LPS in the vaginal tract during birth activates the neonatal gut epithelium via TLR4 and temporarily upregulates microRNA-146a (miR-146a), which leads to degradation of the TLR signaling molecule IL-1R-associated kinase 1 (IRAK1). Such translational repression of IRAK1 protects the epithelium from microbiota-induced damage during transition from a relatively sterile environment [104, 127]. In remarkable contrast, epithelial tolerance to the commensal bacteria does not occur in mice delivered by cesarean section [103]. These experimental observations may be related to the fact that children delivered by cesarean section appear particularly prone to develop food allergy if they have a genetic predisposition for atopy [128, 129].
The epithelial expression of the NF-kB inhibitor IkBα steadily increases during the postnatal period [130]. The combination of decreasing levels of TLR4 and increasing levels of IkBα in the epithelial cells effectively increases the threshold of immune activation in the gut epithelium (Fig. 2.8). Also interestingly, decreased IRAK1 protein expression in mouse neonatal epithelium requires continuous TLR signaling. This facilitates prolonged upregulation of miR-146a expression and simultaneously induces sustained expression of genes supporting cell maturation, survival and nutrient absorption [131]. Innate immune signaling by epithelial cells seems to be essential for immune tolerance, as lack of the proinflammatory signaling molecule TGF-β-activated kinase 1 (TAK1) specifically in the murine intestinal epithelium leads to early inflammation, tissue damage and postnatal mortality [132]. Thus, although inappropriate stimulation of the neonatal innate immune system by the microbiota must be prevented, controlled innate immune activation significantly contributes to nutrient absorption, angiogenesis, epithelial cell differentiation, and barrier reinforcement [133].
Despite the decreased sensitivity of epithelial cells to TLR stimulation in murine neonates, other innate immune signaling pathways remain fully functional. For example, rotavirus infection of the intestinal epithelium in neonatal mice is efficiently sensed by the helicases retinoic acid-inducible gene I (RIG-I) and melanoma differentiation-associated gene 5, or MDA5 [134]. Confronted with the colonization of the gut microbiota, therefore, the neonatal intestinal epithelium seems to calibrate its bacterial sensitivity and modify its signaling pathways after initial stimulation while maintaining antiviral host defenses.
Mouse experiments have thus demonstrated a crucial postnatal role of commensal gut colonization, both in establishing and regulating the epithelial barrier, which also includes upregulation of pIgR expression [133, 135]. Such beneficial effects of microbiota-derived MAMPs appear again to be mediated largely via PRRs, particularly TLRs and similar innate sensors on the epithelial plasma membrane (apically or basolaterally) or on endosomal membranes [101, 136]. Cell culture experiments with the polarized human colon carcinoma cell line HT-29 have shown that ligation of TLR3 and TLR4 with the respective MAMPs (double-stranded RNA and LPS) from the apical side upregulates pIgR expression (Fig. 2.12) [137], which may then increase SIgA (and SIgM) export and thus enhance secretory immunity [138]. Gut bacteria of the family Enterobacteriaceae can induce this effect [139].


Fig. 2.12
Schematic illustrations of three possible manners in which pIgR expression can be upregulated by activation of its gene locus PIGR. The effect of the cytokines interferon (IFN)-γ, interleukin (IL)-4, IL-17, tumor necrosis factor (TNF)-α, and IL-1 can be enhanced by the vitamin A derivative retinoic acid (not shown). The microbial fermentation product butyrate in combination with cytokines also has an enhancing effect on PIGR, and butyrate may directly induce regulatory T (Treg) cells that counteract colitis. Activation of epithelial Toll-like receptors (TLR) may not only active PIGR but also innate defense genes
Vitamin A supports secretory immunity, not only by enhancing IgA switch and homing of mucosal B cells (Fig. 2.4) but also by a positive effect of RA on cytokine-induced pIgR expression in intestinal epithelial cell at the transcriptional level [140, 141]. Several cytokines derived from activated Th cells or APCs may upregulate pIgR expression and thereby increase the export of secretory antibodies in response to microbial stimulation (Fig. 2.12) [40, 138]. A similar pIgR-enhancing effect has been shown for the SCFA butyrate in combination with various cytokines (Fig. 2.12) [142]. Butyrate is an anaerobic microbial fermentation product of oligosaccharides and an important energy source of colonic epithelial cells. Notably, it can increase gene transcription levels through specific DNA sequences [143]. It has, moreover, been shown in different studies that butyrate can directly induce Treg cells in the gut [144] and thereby inhibit experimental colitis in a mouse model [145].
Altogether, there are many mechanisms by which exogenous variables may maintain the “tone” of intestinal pIgR expression above the constitutive level and thus reinforce immune exclusion. In addition, polarized gut epithelial cells seem to retain their ability to dampen the proinflammatory effect of PRR-mediated signals coming from the luminal side [135, 136, 146]; but after bacterial invasion, PRR signaling from the basolateral side results in NF-kB activation with release of epithelial defensins to join the combat against infection [101, 147]. Accumulating evidence suggests that barrier-related homeostasis depends on “cross talk” between the epithelium (via cytokines and other factors) and lamina propria cells including macrophages, DCs, and T cells [148–151].
Thus, when immune regulation is operating in a healthy manner, the small amounts of MAMPs and exogenous antigen penetrating into the lamina propria seem to be handled in a homeostatic manner by DCs and macrophages, with a balanced cytokine secretion and induction of Treg cells (Fig. 2.13). However, if the influx is excessive or regulatory mechanisms are defect, immune reactions may be driven into hypersensitivity and enter a vicious circle with proinflammatory cytokines and epithelial apoptosis [12, 150–152]. In this manner a “point of no return” may be reached, as seen in IBD with apoptosis-resistant pathogenic T cells and tissue damage (Fig. 2.13). The take-home lesson from most disease models in gene-manipulated animals is that a predilection exists for immunopathology to occur in the distal gut—where most commensals reside—when adaptive immunity is dysregulated and innate immunity or the intestinal barrier function compromised [153, 154].


Fig. 2.13
Model for maintenance of mucosal homeostasis in the gut and abrogation of oral tolerance. Epithelial barrier variables, including secretory IgA (SIgA), mucus, defensins, genes (e.g., regulating tight junctions), and cytokines are indicated in the light green panel to the left. (1) Normal permeability of the gut epithelium allows some MAMP (microbe-associated molecular pattern) and exogenous antigen (Ag) uptake (amounts of dietary proteins detected in the circulation after a meal are indicated). (2) A minor barrier defect results in increased uptake, but mucosal homeostasis is maintained (green panel) when oral tolerance is adequately induced by quiescent dendritic cells (DCs) and macrophages (Mφs), which both can convert dietary vitamin A to retinoic acid (RA) as an aid in their induction of regulatory T (Treg) cells (providing the suppressive cytokines IL-10 and TGF-β). If there is an innate or immunoregulatory defect, a vicious circle will develop which reciprocally acts also on the regulatory network. (3) With more skewing towards regulatory dysfunction, the vicious circle will activate the epithelium, and MAMP and Ag uptake is enhanced (graded vertical arrows), both by increased permeability and aberrant receptor (R) expression apically on epithelial cells. Food allergy may occur in the dysfunctional, to some extent reversible, zone between 2 and 3, as indicated. (4) The adverse development may finally result in epithelial activation and apoptosis, increased secretion of proinflammatory cytokines, chronic inflammation, apoptosis-resistant effector T cells, chronic inflammatory bowel disease (IBD), and tissue damage (red panel to the right)
The Role of Mucus in Intestinal Barrier Function
Goblet cells secrete mucin glycoproteins and MUC2 is the major mucin making up the mucus coat of the intestinal epithelium [87]. This coating is of great importance for the interactions between the microbiota and the immune system [155]. The epithelium of the small intestine is covered by a single loose mucus layer which allows microbial penetration (Fig. 2.14a). Nevertheless, bacteria are normally kept at a certain distance from the epithelial cells because of antimicrobial peptides such as REG3γ (Fig. 2.8) [156]. In the colon, however, the mucus coat is stratified, with a relatively compact inner layer ranging in thickness from 50 μm in the mouse to several hundred microns in humans (Fig. 2.14b) [157]. This layer normally resists microbial penetration although allowing diffusion of structural components of bacteria (e.g., MAMPs) and metabolic products such as SCFAs (Fig. 2.12). The outer mucus layer is much looser and contains a large number of bacteria which are retained there partly because of SIgA antibodies (Fig. 2.14c).


Fig. 2.14
Schematic depiction of the small intestine and colon with the varying distribution of mucus. (a, b) Intestinal epithelial cells are produced from stem cells near the bottom of the glands (named crypts) and move upwards to replace the loss of apoptotic cells. Stem cells also give rise to mucus-producing goblet cells, and to Paneth cells which move downwards to the bottom of the crypts and produce a variety of antimicrobial peptides (AMP). Intraepithelial lymphocytes (IEL) are more numerous in the small intestine than in the colon. The small intestine is covered by a loose layer of mucus whereas the colon has a compact inner layer and an outer loose layer where bacteria are retained. (c) Three-color immunofluorescence of a tissue section from the human colon, showing that IgA is concentrated in the outer mucus layer (OM) but hardly detectable in the inner mucus layer (IM). The cartoon on the left is adapted from Fig. 1 in Mowat and Agace [18] while the immunofluorescence illustration on the right is from Fig. 6 in Rogier et al. [159]
Rogier et al. [158] examined the role of mucus and SIgA in inhibiting translocation of bacteria from the gut lumen by exploiting knockout mice deficient in pIgR. Pigr +/− females were crossed with Pigr −/− males, and vice versa. Colonic lumens of newborn offspring of Pigr −/− dams were devoid of IgA, whereas offspring of Pigr +/− dams had abundant IgA in colonic lumens and feces. At weaning these levels dropped, reflecting the loss of maternal SIgA, but then at about 4 weeks began to increase in Pigr +/− offspring because of endogenous pIgR-mediated external transport of SIgA. Failure to receive maternal or endogenous SIgA resulted in translocation of aerobic bacteria into MLNs. Early exposure to SIgA in breast milk produced a gene expression pattern of epithelial cells different from that of mice not receiving SIgA, which mimicked that associated with IBD in humans. Also, maternal SIgA reduced colonic damage in a DSS-model of IBD. It was concluded that breastfeeding may promote life-long intestinal homeostasis.
In a different publication [159], the same authors found that SIgA co-localized with gut bacteria in the outer colonic mucus layer. By using mice deficient for pIgR and/or Muc2, they found that Muc2 plays a dominating role in excluding bacteria. Accordingly, mice deficient in Muc2 develop a spontaneous colonic inflammation similar to IBD in humans [160].
Interactions of SIgA with Commensal Bacteria
A great proportion of the commensal bacteria in the healthy human gut or at other mucosal surfaces are coated by IgA [161, 162], probably representing mainly low-affinity SIgA antibodies (Fig. 2.7). Such microbial–host interactions contain commensal bacteria in the mucus without eliminating them (Fig. 2.14). Thus, it has been shown in a murine infection model with Salmonella typhimurium that bacteria coated with innate-like, cross-reactive SIgA antibodies showed reduced shedding and less liability to horizontal spreading by the fecal–oral route [95, 110]. Mouse experiments have further documented that a gut IgA response to a single commensal microbial epitope (capsular polysaccharide A, PSA) can be immunomodulatory and protect against pathogen-induced colitis [48]. Germfree mice monocontaminated with the gut commensal Bacteriodes thetaiotaomicron demonstrated that specific SIgA antibodies directed against PSA in the gut inhibit activation of innate response markers such as oxidative burst and NFkB, thereby inducing crucial modulation of immune homeostasis as well as antigenic drift [163]. SIgA antibodies can hence control the intestinal microbiota in a non-inflammatory manner, promoting mutualism with the host [164].
Although mucosal IgA responses to commensal bacteria may be multicentered, of low affinity and diverse, oral immunization of mice with a cholera toxin-adjuvanted novel antigen was shown to result in a strong oligoclonal response of affinity-matured IgA+ B cells [165]. Interestingly, it was found that the response was highly synchronized throughout the entire intestine by involving multiple Peyer’s patches. Thus, by reutilizing already existing CGs, antigen-specific B cells would be subjected to clonal expansion and somatic hypermutation. This process was shown to require antigen recall by multiple immunizations, and the study helps to clarify mechanisms underlying the functional flexibility of mucosal antimicrobial IgA responses: from “natural” polyreactive (or cross-reactive) low-affinity to a specific high-affinity “classical” response (PB)—a distinction that is a major challenge to mucosal vaccine design [166].
The difficult issue of affinity maturation of mucosal B cells, and how the mucosal immune system can distinguish between the indigenous microbiota and overt exogenous pathogens, has been discussed in several articles [167–171]. The mucosal barrier and its reinforcement by SIgA, as well as the mucosal immunoregulatory network, require both adaptive and innate induction by antigens and conserved MAMPs—the latter activating germ line-encoded cellular PRRs such as TLRs [10, 11]. It is elusive how such receptors would be able to discriminate between signals provided by MAMPs from commensals and MAMPs from pathogens (previously called pathogen-associated molecular patterns, PAMPs); but this distinction is clearly required to elicit tolerogenic versus proinflammatory immune responses needed for protection against invasive infections (Fig. 2.15). Various scenarios may be visualized—the most likely being that overt pathogens, in addition to signaling through PRRs and B-cell receptors, exhibit special danger signals or immune evasion mechanisms related to the pathogenicity—that is, factors determining virulence and invasiveness [172–174], or the so-called effector-triggered immunity [175, 176], while competition for metabolically shared nutrients may also be involved [177, 178].


Fig. 2.15
Hypothetical depiction of how the intestinal immune system handles symbionts and potentially pathogenic residents (pathobionts) of the commensal microbiota versus overt exogenous pathogens. Secretory IgA (SIgA) antibody levels against commensal bacteria may go in waves because of epitope drift and shielding of gut-associated lymphoid tissue from antigen uptake. The overall affinity of SIgA antibodies probably increases with age and may be enhanced (or reduced?) against pathobionts during dysbiosis, and particularly raised by persistent stimulation with overt pathogens. One goal of mutualism with commensals is mucosal barrier reinforcement by mechanisms listed such as SIgA export and induction of regulatory T cells and antimicrobial peptides, whereas pathogens as exemplified in the right panel exhibit various virulence mechanisms to break the barrier. Adapted from Brandtzaeg [55]
In human feces, some 40 % of the anaerobic bacteria are normally coated with IgA162 and this phenomenon can be observed in early childhood [179]. Such IgA containment of commensals, without eliminating them, is probably important for the mutual host–microbe interaction, contributing to sustainable homeostasis by dampening proinflammatory signaling in the host and providing an immune pressure on commensal bacteria [178, 180]. This results in antigenic drift without altered composition of the microbiota, or the so-called dysbiosi s [163, 181]. Most likely this IgA coat largely represents “natural” cross-reactive antibodies [20, 60] but may also depend on innate properties of SIgA, as discussed previously [3]. In mouse experiments with Salmonella typhimurium infection it was found that bacteria coated with such “natural” IgA showed reduced shedding and less liability to horizontal spreading by the fecal–oral route [110].
The IgA coating of gut bacteria in mice has been shown to be unrelated to the total amount of SIgA exported to the intestinal lumen, supporting that a specific reaction is involved [182]. Also, other mouse experiments demonstrated that the commensal coating with IgA in feces depended on appropriate clonal B-cell selection and affinity maturation in GCs of GALT, and perhaps to some extent also in the lamina propria [181]. This finding showed that the coating to a substantial degree reflects a specific IgA response. It has therefore been speculated that potentially pathogenic commensals (pathobionts) might show increased IgA coating in the gut lumen. This idea is supported by findings in patients with IBD, where there is dysbiosis [178, 183] and the fraction of anaerobic bacteria with IgA is raised to 65 %, with 45 % also carrying IgG [184]. Thus, increased antibody coating could reflect dysbiosis (Fig. 2.15). However, in celiac disease, where there is also dysbiosis [185], the IgA coating of fecal bacteria is significantly reduced [179]. So the biological significance of this phenomenon remains uncertain, and the degree of IgA coating might reflect a combination of innate and specific bacterial binding properties of SIgA. In a recent study, however, it was suggested that high SIgA coating of certain commensals trapped in the mucus identified colitogenic bacteria able to drive colitis in a mouse model [186].
Altogether, SIgA does not seem to cause clearance of commensal bacteria, but controls in various ways their colonization and inhibits the penetration of agents that could potentially cause hypersensitivity reactions or infection [55]. In the absence of B cells, or when IgA is lacking, the intestinal epithelium of mice will, in response to commensal bacteria, upregulate its innate defense in an NFkB- and interferon-dependent manner [55, 180]; this could be at the expense of expression of genes that regulate fat and carbohydrate metabolism [187]. As a consequence, the epithelial gene signature might correlate with the development of lipid malabsorption [188]. The intestinal epithelial barrier is a cross-road between surface defense and nutrition, and SIgA is apparently essential to keep the balance between these two functions and thus maintain mucosal homeostasis.
Experimental studies have also revealed how intestinal homeostasis is mediated by host–microbe interactions in mice mono-colonized with the Clostridia-related segmented filamentous bacterium (SFB) which particularly grows in the distal ileum [189, 190]. SFB adheres to murine GALT structures and stimulate T-cell as well as IgA responses. Here a distinction is needed between murine B1 (T cell-independent) and B2 (T cell-dependent) responses when comparing these structures with the lamina propria; this distinction is as yet not clear [5, 36]. It is also possible that GALT may take up immune complexes containing SIgA bound to commensals via an as yet uncharacterized receptor for IgA on M cells and then induce a homeostatic response (Fig. 2.16). This mechanism was suggested to direct the opportunistic bacterium Alcaligenes into murine GALT and thereby make these tissue structures more resistant to pathogen invasion [191]. More recently it has been shown that goblet cells may also be involved in uptake of antigens from the gut lumen [192].


Fig. 2.16
Putative integration of the immune systems of mother and her breast-fed baby via M cell-mediated antigen uptake. Secretory IgA (SIgA) antibodies in breast milk may guide induction of the infant’s intestinal immune system because M cells of Peyer’s patches express receptor(s) for IgA. This as yet uncharacterized receptor may facilitate uptake of antigens that have formed immune complexes with cognate maternal SIgA antibodies in the gut lumen of the infant. The complexes may be further target to dendritic cells (DC) which carry them to mesenteric lymph nodes where a homeostatic immune response dominated by secretion of TGF-β and IL-10 is induced. Based on experimental data reviewed by Corthésy [3]. Details of M-cell pocket with its cellular content is schematically shown in the panel on the left. FAE follicle-associated epithelium, Mφ macrophage, APC antigen-presenting cell, FDC follicular DC, TGF transforming growth factor, IL interleukin
Role of IgA and Breastfeeding in Immune Contro l
The level of SIgA appears to contribute to an individual’s threshold for hypersensitivity reactions to exogenous antigens [12]. Thus, the risk of contracting allergy seems increased when the development of the IgA system is retarded and the SIgA-dependent barrier function insufficient [193]. Minor dysregulations of both innate and adaptive immunity (especially IgA) have been observed in children with multiple food allergies [194]. These clinical observations are in accordance with the hyperreactivity (Fig. 2.9) and sensitivity to DSS-induced colitis seen in pIgR knockout mice [96].
It is therefore not surprising that exclusive breastfeeding up to the age of at least 4 months has an allergy-preventive effect, also in families without atopic heredity [195–197]. Moreover, having been breast-fed for at least 3 months protects against both Crohn’s disease and ulcerative colitis in adulthood [198], and probably also against pediatric IBD [199]. Importantly, mothers with IBD receiving anti-inflammatory drugs can in most cases safely breastfeed their babies, and it may even provide a protective effect against disease flare [200–202].
In addition to the remarkable reinforcement of mucosal defense provided by maternal SIgA (and SIgM) antibodies as a natural immunological “substitution therapy,” it is important to emphasize the positive nutritional effect of breastfeeding on immune development [193]. Mother’s milk also contains a number of immune cells, cytokines, and growth factors that may exert a significant biological effect in the breast-fed infant’s gut, apparently enhancing in an indirect way even the subsequent health of the individual [34, 193, 203]. Moreover, its high content of human-specific oligosaccharides serves as a prebiotic promoting the growth of lactic acid-producing bacteria and reducing anaerobic bacteria in the gut of breast-fed infants [193]. Probiotic bacteria can reportedly also occur in breast milk [204], perhaps after being transported to the mammary glands from the gut by DCs [205].
Numerous studies of the effect of breastfeeding on the development of secretory immunity have been performed with salivary IgA measurements as a readout system. Discrepant observations have been made and the influence of contaminating the sample with milk SIgA, shielding of the suckling’s mucosal immune system by maternal SIgA antibodies, and altered growth and composition of the infant’s gut microbiota have been discussed as possible uncontrollable variables. Moreover, several prospective studies have reported that the postnatal increase of salivary IgA (and IgM) initially is more prominent in formula-fed than in solely breast-fed infants [25].
Nevertheless, evidence suggests that breastfeeding promotes the development of secretory immunity over time [26, 193], apparently even at extraintestinal sites such as the urinary tract [203]. Thus, although breastfeeding initially may reduce the induction of SIgA, it appears later on in infancy (up to 8 months) to boost secretory immunity. As mentioned above, one possibility is that SIgA antibodies in mother’s milk guide the uptake of cognate luminal antigens via receptor(s) for IgA on M cells (Fig. 2.16); as suggested by mouse experiments, the antigens may further be targeted to DCs which migrate to MLNs where they induce a homeostatic immune response [3].
Traces of exogenous antigen transferred into the milk of mothers may thus contribute to the induction of oral tolerance in the breast-fed infant; therefore, allergen avoidance during lactation is no longer recommended [12]. Various mouse models have indeed documented that antigen appears in the milk of exposed mothers and that it can induce Treg cells in the suckling neonate, either together with TGF-β or after complexing with IgG antibody and transfer by FcRn into gut mucosa [206, 207]. In human milk, maternal SIgA-containing immune complexes may perform a similar function (Fig. 2.16). Interestingly in this context, a review of TGF-β levels in human milk suggested that this cytokine protects against allergy in the breast-fed infants and young children [208]. It has also been reported that genes modulated during epithelial gut differentiation in the neonate, are differentially expressed in breast-fed and formula-fed infants [209]. It is unknown whether this was a direct effect of milk components or caused by differences in the microbiota of the two feeding groups.
Mucosal Homeostasis Versus Hypersensitivity
Oral Tolerance in Human s
It is believed that oral tolerance is largely explained by different T-cell events such as anergy, clonal deletion, and induction of Treg cells by conditioned APCs, although other regulatory principles may be involved [12, 26, 193, 210–212]. For ethical reasons, the existence of mucosally induced tolerance in human beings is supported mainly by circumstantial evidence. Thus, the gut mucosa of healthy individuals contains virtually no hyperactivated T cells and hardly any proinflammatory IgG production, and their serum levels of IgG antibodies to food antigens are low [193]. Moreover, the systemic IgG response to dietary antigens tends to decrease with increasing age [213, 214], and a hyporesponsive state to bovine serum albumin has been demonstrated by intradermal testing in adults [215]. Interestingly, nasal application or feeding of a novel antigen (keyhole limpet hemocyanin) in healthy people induced peripheral downregulation of T-cell immunity and, less consistently, also suppressed systemic antibody responses to subsequent parenteral immunization [216, 217]. By contrast, oral tolerance could not be induced in patients with IBD where the epithelial barrier is severely deteriorated and the immunogenetics favors mucosal inflammation [218, 219].
Mucosal Tolerance Induction
In healthy human gut mucosa, resident APCs are quite inert in terms of immune-productive stimulatory properties [220], and they hardly express detectable surface levels of TLR2 or TLR4 [221]. Also, only negligible expression of the LPS co-receptor CD14 is normally observed on these cells, and their proinflammatory cytokine response is usually low after LPS stimulation [113, 222]. Nevertheless, the phagocytic and bacteriocidal activity of mucosal macrophages is maintained [223], which would be important for silent clearance of commensal bacteria normally penetrating into the mucosa in small numbers [11, 20, 224].
These observations support the notion that both macrophages and DCs play a central role in oral tolerance [13], and most human intestinal APCs come from a common myeloid progenitor and often show an intermediate phenotype [225, 226]. Heterogeneity of murine lamina propria APCs has also been highlighted [227, 228]. In vivo observations in mice suggest that DC development from monocytes to the phenotype DC-SIGN/CD209+ is a predominant pathway when abundant LPS is available [229], such as in the gut. The subepithelial band of monocyte-derived CD103–CX3CR1+ cells in the murine gut mucosa is indeed macrophage-like [226]. These cells express tight-junction proteins and can extend their dendrites into the lumen to sample antigens.
In a quiescent steady state, however, mucosal CD103+CCR7+ DCs (and possibly macrophages) are migratory and carry penetrating dietary and innocuous microbial antigens away from the gut mucosa after being transferred to them from CX3CR1+ cells through gap junctions [33, 230–232]. This may be particularly relevant for the upper small intestine where the CD103+ DCs end up in lymph nodes whose environment is different from that of MLNs in the more distal gut [18]. In the mucosa-draining lymph nodes a portion of the migrating CD103+ DCs becomes conditioned for tolerance induction and drives the expansion of Treg cells [26]. It has been shown in humans that the tolerogenic properties of these DCs partly depends on expression of indoleamine 2,3-dioxygenase (IDO) [233]—an enzyme known to be involved in DC induction and function [234]. Hyperactivation of effector T cells in the intestinal mucosa with accompanying inflammation can thus be inhibited by this DC-dependent regulatory mechanism, both initially and subsequently. This is so because homeostatic control is also exerted when the generated Treg cells home from the lymph node to the lamina propria [12]—a homing that seems to be particularly active in infancy [235]. In the lamina propria the Treg cells are then expanded by IL-10 derived from macrophages [236].
A dietary effect on the induction of Treg cells is exerted through the conversion of vitamin A to RA by the enzyme RALDH, which is expressed both by intestinal DCs, macrophages, and epithelial cells [37, 227], as well as by MLN stromal cells [237]. In the upper part of the small bowel, retinoids from bile may exert an important enhancing effect [18, 238]. In a recent study RA signaling in B cells was, moreover, shown to be essential for their IgA response and for interacting with the microbiota [239]. The impact of bile retinoids on the mucosa of the upper small intestine may therefore be crucial for immune homeostasis in relation to both dietary and microbial antigens in that region.
Together with IL-2, TGF-β and IL-10, RA can drive induction of Treg cells [240–243]—which in the human periphery are heterogeneous and apparently may differentiate either by conversion from a naive (CD45RA+) phenotype or from rapidly proliferating T cells with a memory/effector (CD45R0+) phenotype with different migratory properties [244, 245]. It is the latter that expresses high levels of the transcription factor Foxp3 and seems to be actively suppressive [246].
Most information about Treg cells is necessarily derived from mouse experiments. Human Treg cells seem to be functionally and phenotypically more diverse than the murine counterparts, including the expression of the activation marker CD25 and the Foxp3 transcription factor [247]. There is also a need for better understanding for the functional stability and plasticity (e.g., acquisition of a Th17 phenotype) of Treg cells [248].
Recognition of Microbial Components
Final cellular conditioning for oral tolerance in MLNs appears to depend on appropriate stimulation of the migrating mucosal APCs by certain MAMPs derived from commensal bacteria (Fig. 2.17), which induce the signaling molecules and transcription factors dictating the differentiation pathways and cytokine profiles of the activated T cells [11, 12, 249]. Components of intestinal parasites such as helminths can exert similar immune modulation [10, 14, 250–252]—apparently to some extent through induction of Treg cells by mimicking the effect of TGF-β by ligation of its receptor [253]. Indeed, helminth-derived immunomodulators may be important in future medication for IBD [254]. Also of note, several studies suggest that LPS plays a central role in the early programing of the immune system [255, 256]; and there is an ongoing search to find out if hypersensitivity to exogenous factors is associated with hereditary single nucleotide mutations (polymorphism) in PRRs recognizing this and other MAMPs, such as CD14, TLR2, TLR4, and NOD [257, 258]. For instance, a proof of principle in Crohn’s disease is the dose effect of mutations affecting the intraepithelial PRR function of the NOD2 (CARD15) gene encoded by the IBD susceptibility locus (IBD1) on chromosome 16 [259]. One of several putative pathogenic mechanisms is illustrated (Fig. 2.18) [260].



Fig. 2.17
Decision-making in the mucosal immune system is modulated by co-stimulatory signals (cytokines and ligands) operating in the synapse between antigen-presenting cell (APC) and T cell. Activation of CD4+ T cells occurs when APC takes up antigen and processes (degrades) it to immunogenic peptides for display to the T-cell receptor (TCR) in the polymorphic grove of HLA class II molecules (HLA-II). The level of co-stimulatory signals determines T-cell modulation (activation and conditioning). When CD4+ helper T (Th) cells are primed for productive immunity, they differentiate into Th1, Th17, or Th2 effector cells with polarized cytokine secretion (blue panels on the right). Such skewing of the adaptive immune response depends on the presence of microenvironmental factors, including cytokines, as well as signals from microbial components. Bacterial endotoxin (lipopolysaccharide), lipoproteins, unmethylated CpG DNA, and other conserved structural motifs are called microbe-associated molecular patterns (MAMPs); they are sensed by cellular pattern-recognition receptors (PRRs) such as CD14, toll-like receptors (TLRs), C-lectin receptors (CLRs), and NOD-like receptors (NLRs), including NOD2. Signaling from PRRs to the nucleus of APCs and T cells stimulates various degrees of activation and functional maturation of APCs and will thereby dictate differential expression of various co-stimulatory signals directing activation of either Th1 or Th17 or Th2 cells. Their cytokines induce various adaptive defense mechanisms or immunopathology (red panels on the right). The Th cytokine profiles are further promoted by the depicted positive and inhibitory feedback loops. Under certain conditions, rather immature but yet conditioned APCs may induce various subsets of regulatory T (Treg) cells as indicated in the light green area; by their cytokines IL-10 and TGF-β, or by interactions depending on CTLA-4 and the transcription factor Foxp3, the Treg cells can suppress Th1, Th17, and Th2 responses, including pathogenicity with detrimental innate immunity and inflammation (red panels on the right). CTLA-4, cytotoxic T-lymphocyte antigen 4; IFN, interferon; IL, interleukin; TGF, transforming growth factor; TNF, tumor necrosis factor. CMI cell-mediated immunity, DTH delayed-type hypersensitivity

Fig. 2.18
Hypothetical model for the role of NOD2 (CARD15) in maintenance of intestinal mucosal homoeostasis (left), and how a defect in this susceptibility gene for Crohn’s disease may impair the epithelial barrier function and lead to innate inflammation (right). Secretion of antimicrobial peptides, such as defensins from epithelial cells (particularly Paneth cells), depends on intracellular sensing of CARD15/NOD2 ligands, particularly the peptidoglycan muramyl dipeptide (MDP). Certain mutations in CARD15/NOD2 impair this defense function, leading to penetration of luminal antigens and bacteria which will result in acute inflammation with accumulation of polymorphonuclear granulocytes (PMN). However, when this innate response is defect, as seen in Crohn’s disease, antigen-presenting cells (APC), such as dendritic cells (DC), will be hyperactivated, leading to a strong chronic effector response with stimulation of pathogenic Th1 and Th17 cells. Model based on an idea proposed by Marks et al. [265]
Altogether, the extended hygiene hypothesis implies that suboptimal PRR stimulation, with delayed maturation of the mucosal immune system with insufficient induction of Treg cells or other components of the mucosal barrier defense, contributes significantly to the increasing incidence of not only allergy—commonly reflecting overactivation of Th2 cells—but also other immune-mediated inflammatory disorders such as IBD—reflecting overactivation of Th1 or Th17 cells (Fig. 2.18). In the perspective of evolution, Th2 cells have had a crucial role in host defense against parasites [261], whereas Th1 and Th17 cells are normally important for proper defense against infections [10, 14].
This basis for the hygiene hypothesis has been tested in several clinical studies evaluating the beneficial effect on immune homeostasis exerted by probiotic bacterial preparations derived from the commensal intestinal microbiota and eggs of the porcine helminth (whipworm) Trichuris suis [14, 252]. In this context, viable strains of lactobacilli and bifidobacteria have been reported to enhance IgA, both in humans and experimental animals, but these responses have not translated convincingly into clinical effects [262, 263]. To select the right probiotic strains, or symbiotic combinations with prebiotics or breast milk, remains a difficult task—and there are safety issues [264, 265]. Experimental studies have indicated that certain probiotic bacterial strains in fact may be proinflammatory while others may induce DCs, directly or via epithelial mediators, to exert anti-inflammatory effects [266].
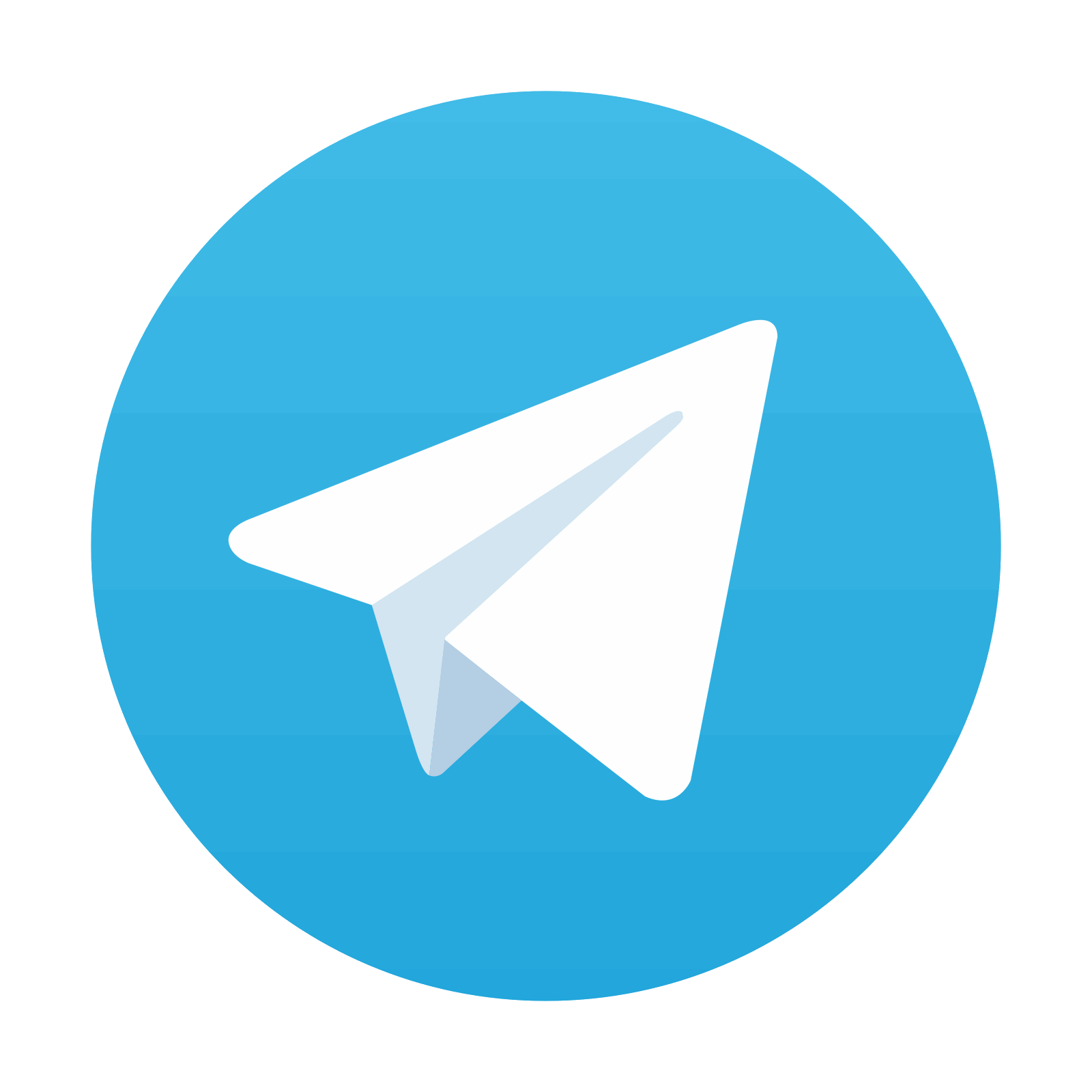
Stay updated, free articles. Join our Telegram channel

Full access? Get Clinical Tree
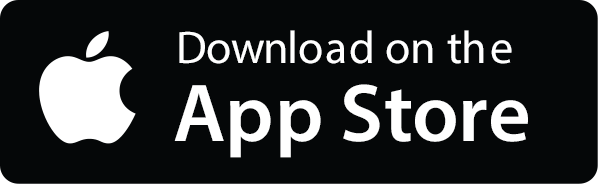
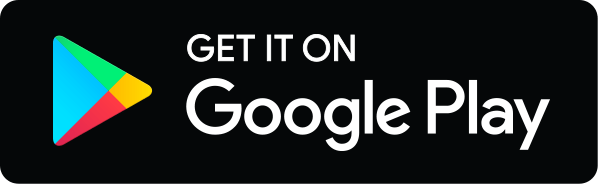