Target of investigation
Available tests
Antisperm antibodies
Mixed agglutination reaction (MAR) test
Direct immunobeads binding test (direct IBT)
Fertilization defects
Hemizona assay
Sperm–zona binding ratio test
Hyperactivation (HA) motility
Acrosome reaction assays
Sperm penetration assay (SPA)
Oxidative stress
Thiobarbituric acid reactive substances (TBARS) assay
Chemiluminescence assays
Superoxide anion by nitroblue tetrazolium (NBT) reduction test
Sperm DNA integrity
Terminal deoxy nucleotidyl transferase mediated dUTP nick end labeling (TUNEL) assay
Comet assay
Sperm chromatin structure assay (SCSA)
Sperm chromatin dispersion (SCD) test
For many years, tests that assessed ASA, AR, capacitation, sperm–zona, and ovum–membrane binding, and penetration were used not only to investigate males with UI but also to predict the fertilizing potential of sperm. Unfortunately, clinicians do not have effective clinical treatment to offer their patients when defects in the aforementioned steps are found. Still, with the advent of intracytoplasmic sperm injection (ICSI) , these issues are no longer a concern as these critical steps are bypassed by ICSI. As such, many of the tests have been abandoned or are rarely used. In contrast, modern Andrology testing involving genetics , proteomics, and metabolomics holds much more promise since there is an opportunity for treatment in the face of abnormal results. Examples of tests in these categories, which have already been made available in the clinical setting, include sperm DNA chromatin tests (genetics) and quantification of oxidative stress markers (metabolomics). Sperm DNA integrity tests are of particular interest provided they are low cost and easy-to-implement. Moreover, results correlate with spontaneous, conventional in vitro fertilization (IVF), and ICSI outcomes, thus hindering sperm chromatin testing a valid sperm biomarker.
In this chapter, we describe the tests clinically available to investigate males with UI , including the traditional and novel ones, and discuss their utility in practical terms. We also present our experience in using some of these tests in our laboratories.
Antisperm Antibodies
ASA, the hallmark of autoimmune male infertility, are implicated in sperm dysfunction by their direct effect on various sperm antigens. ASA may induce sperm apoptosis and untimely AR [3]. ASA may also hinder fertilization by interfering with sperm penetration into the cervical mucus, zona pellucida (ZP) binding, and sperm–oocyte fusion. Furthermore, ASA may alter chaperone function, protein folding, and disulphide bonds [4]. These alterations ultimately decrease the chances of achieving a natural conception [5]. As a matter of fact, Ayvaliotis et al. reported a significantly lower spontaneous conception rates (15.3 %) in couples where autoimmunity to sperm was detected compared with those without clinically relevant levels of ASA (66.7 %, p < 0.05) [5].
Sperm agglutination and/or immobilization is usually observed in association with ASA, and may limit sperm progression through the female genital tract [6]. The findings of sperm agglutination, “shaking motility,” or both, during conventional semen analysis are highly suggestive of elevated ASA titers [7]. The term “agglutination” describes spermatozoa that are stuck to each other by antibodies. Sperm agglutination usually occurs in specific manners such as head-to-head, midpiece-to-midpiece, tail-to-tail, or tail-tip-to-tail-tip [8]. On the other hand, “shaking motility” is a pattern of sperm movement characterized by a very strong tail movement associated with a nonprogressive motility that results in immobilization [8].
Although immunoglobulin subclasses IgA, IgG, and IgM can be found in human ejaculates, IgA seems to be the most important one from a biological standpoint [9, 10]. IgA bound to the sperm surface significantly impair sperm progression through the cervical mucus [10]. Nevertheless, immunoglobulins can adhere to various sperm sites regardless of their subclasses [5].
It is out of our scope to provide a comprehensive review on immunologic male infertility since it is discussed in Chap. 9. Our focus is otherwise to present the tests to determine ASA, as well as their advantages and limitations.
Laboratory Tests to Detect Antisperm Antibodies
Concerning the testing for ASA in UMI, the specimen of interest is the semen . ASA can also be assessed in the seminal plasma when low sperm counts and/or motility limits the applicability of the assay. However, such conditions are associated with abnormal semen analysis results, and therefore do not meet the criteria of UMI. The immunobeads binding test (IBT) and the mixed agglutination reaction (MAR) test are the most commonly used among andrology laboratories performing ASA determination (Table 11.2) [11].
Table 11.2
Methods for assessing antisperm in men with unexplained male infertility
Assay | Principle | Advantage | Disadvantage | Specimen | How results are expressed | Normal limits | Clinical significance in unexplained male infertility |
---|---|---|---|---|---|---|---|
Direct immunobeads test (IBT) | Polyacrylamide beads coated with antihuman immunoglobulin antibodies against α-, γ-, and μ-chains that bound to antibodies present in spermatozoa | Identify the proportion of antibody-bound sperm, the antibody class, and the location of the antibodies on the sperm surface | More time consuming than the MAR test; ASA-positive spermatozoa and ASA-negative spermatozoa should be included as controls in each test | Ejaculated sperm | Percentage of motile spermatozoa with beads bonded to their membrane | ≤ 50 % of motile sperm bounded to the beads | Significantly lower (15.3 %) conception rates in couples with sperm autoimmunity compared to couples without clinically relevant levels of ASA (66.7 %, p < 0.05) [5] |
Mixed agglutination reaction (MAR) test | A “bridging” antibody (anti-IgG or anti-IgA) is used to bring the antibody-coated beads into contact with unwashed spermatozoa in semen bearing surface IgG or IgA | Inexpensive, quick and sensitive screening test; agglutination between beads serves as a positive control for antibody–antigen recognition | Provides less information than the direct immunobead test |
Mixed Agglutination Reaction Test
The MAR test is an inexpensive, quick, and sensitive screening test in which sheep erythrocytes or latex particles coated with human IgG or IgA are used to detect and localize antibody-bound sperm [12, 13]. The formation of mixed agglutinates between the particles/red blood cells and motile spermatozoa indicates the presence of IgG or IgA antibodies on the spermatozoa.
Immunobeads Binding Test
IBT is more time consuming than the MAR test, but it identifies the proportion of antibody-bound sperm in a given sample, the antibody class, and the location of antibodies on the sperm surface. IBT is our preferred approach to determine the levels of ASA in the semen, seminal plasma, and cervical mucus. IBT involves the use of poliacrilamide microspheres coated with human anti-immunoglobulin combined IgA, IgG, and IgM classes. These treated beads adhere to light or heavy antibody chains [14]. The test is termed “direct” when it investigates the presence of ASA on the surface of motile spermatozoa in ejaculates (direct IBT), and is termed “indirect” when used to test for ASA in the seminal plasma or cervical mucus (indirect IBT). First, a screening test is performed to determine the percentage of spermatozoa with surface antisperm-bound, regardless of immunoglobulin subclasses. In case of positive results, ASA subclasses can be assessed and quantified. The method involves the incubation of an aliquot of liquefied semen containing 8–10 million motile sperm with a suspension of immunobeads diluted with protein-supplemented phosphate buffer saline (PBS). The mixture is then washed by centrifugation and resuspended in PBS supplemented with 5 % bovine serum albumin (BSA). An aliquot of 8 µL of sperm suspension and immunobeads is placed on a glass microscope slide, and checked for the presence of ASA. Slides are analyzed under phase-contrast microscope at 400× magnification to determine the presence of beads bounded to the sperm surface (Fig. 11.1). Only motile spermatozoa are evaluated and at least 200 cells are analyzed. Results are based on the percentage of spermatozoa with beads bounded to their membrane [6, 15]. The cutoff value for normality is ≤ 50 % of motile sperm bounded to the beads according to the World Health Organization (WHO) guidelines for assessing the human sperm [16].
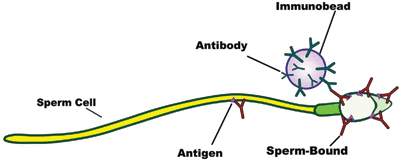
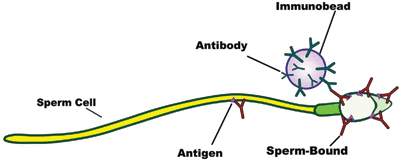
Fig. 11.1
Illustration depicting the immunobeads binding test (IBT). Human anti-immunoglobulin-coated beads are mixed with motile spermatozoa. Anti-immunoglobulins (antibodies) combine with the immunoglobulins (sperm-bound) present on the sperm surface. The presence of beads bounding to the sperm surface is examined under phase-contrast microscopy
Fertilization Defects
The sperm fertilizing potential is related to its ability of undergoing capacitation, ZP binding, and AR, which ultimately leads to sperm penetration into the oocyte and fusion with the oolema .
In vivo, ejaculated spermatozoa from all Eutherian mammals are unable to fertilize until they have undergone capacitation, which allows the AR to take place, when they approach or contact the oocyte [17]. Capacitation has been recognized as a time-dependent phenomenon, with the absolute time course being species-specific [18]. Capacitation prepares the sperm to undergo AR with the accompanying release of lytic enzymes and exposure of membrane receptors, which are required for sperm penetration through the ZP and for fusion with the oolema [18]. Sperm transport through the female genital tract can occur quite rapidly (times as short as 15 to 30 min have been reported in humans), whereas the capacitation process can take from 3 to 24 h [18]. It is speculated, therefore, that capacitation is not completed until after spermatozoa have entered the cumulus oophorus. This delay is physiologically beneficial because spermatozoa do not respond to AR-inducing signals until they have approached the ZP, thus preventing premature AR that ultimately leads to sperm inability to timely penetrate the egg vestments [18, 19]. Sperm capacitation is a postejaculatory modification of the sperm surface, which involves the mobilization and/or removal of certain surface components of the sperm plasma membrane, such as glycoproteins, decapacitation factor, acrosome-stabilizing factor, and acrosin inhibitor. Sperm capacitation involves major biochemical and biophysical changes in the membrane complex and energy metabolism . The presence of high concentrations of cholesterol in the seminal plasma, which maintains high cholesterol concentration in the sperm membranes, seems to be the most important factor for inhibition of capacitation [20]. Capacitation is associated with increased membrane fluidity caused by removal of cholesterol from sperm plasma membrane via sterol acceptors from the female tract secretions [21, 22]. A marked change in sperm motility, named hyperactivation (HA), is the first step in this complex process. Hyperactivated spermatozoa exhibit an extremely vigorous but nonprogressive motility pattern, as a result of a Ca2+ influx, which causes increased flagellar curvature [23] and extreme lateral movement of the sperm head [8]. Proteasome participates in activating calcium channels that also leads to increased membrane fluidity and permeability [24–27]. These events are followed by or occur simultaneously with (i) a decrease in net surface charge, (ii) the absence of intramembrane protein and sterols area, and (iii) increased concentrations of anionic phospholipids [27, 28]. Hyperactivated motility is essential for sperm penetration into the intact oocyte-cumulus complexes in vitro and in vivo [29, 30]. Various components of the female reproductive tract act as physiological stimuli for HA, such as progesterone, ions, and secretions in the oviduct luminal fluid [31]. The extent of which sperm is hyperactivated is positively correlated with the sperm’s ability of zona binding, AR, zona-free oocyte penetration, and thus fertilizing capacity in vitro [31] .
The spermatozoon binds to the ZP with its intact plasma membrane after capacitation and penetration into the cumulus oophorous. Sperm binding occurs via specific receptors to ZP glycoproteins located over the anterior sperm head [32]. Glycosylation of ZP glycoproteins is important in sperm-ZP interaction. It is believed that human ZP glycoprotein-3 (ZP3) has a central role in initiating the AR [33]. However, human ZP1 and ZP4 are also implicated in the process [21, 22, 34–37]. ZP3-induced AR involves the activation of T-type voltage operated calcium channels (VOCCs), whereas ZP1- and ZP4-induced ARs involve both T- and L-type VOCCs. Chiu et al. [38] reported that glycodelin-A, a glycoprotein present in the female reproductive tract , sensitizes spermatozoa to undergo ZP-induced AR. The process involves the activation of the adenylyl cyclase/PKA pathway along with up-regulation of ZP-induced calcium influx and suppression of extracellular signal-regulated kinase activation. It is, therefore, suggested that glycodelin-A may be important in vivo to ensure full responsiveness of human spermatozoa to the ZP. Defective ZP-bound sperms are present in approximately 15 % of subfertile men with normal semen analysis, and it may explain the low success of IVF and intrauterine insemination (IUI) when these methods are applied in certain cases of UI [39, 40]. The presence of defective sperm–ZP binding in infertile men with normal semen parameters may be due to either a defective signal transduction pathways upstream of protein kinases A and C, or downstream disorders, structural defects, or absence of sperm receptors for ZP binding [12]. Such defects are not identified unless specialized sperm function tests are used [41].
The AR is a stimulus-induced process that involves fusion of the sperm plasma membrane with the outer acrosomal membrane. This process leads to the release of exocytotic proteolytic enzymes in response to sperm–ZP binding [42, 43]. The mammalian sperm head is composed of two main structures, the acrosome and the nucleus. The acrosome is a membrane-bound organelle derived from the Golgi complex located in the anterior portion of the sperm head. In humans, the acrosome occupies 40–70 % of the sperm head and its contents include proteases such as proacrosin, hyaluronidase, and phospholipase A2. Spermatozoa bind to the ZP with intact acrosomes. Then, multiple fusions between the outer acrosomal membrane and the plasma membrane result in the release of hydrolytic enzymes (mostly acrosin) and exposure of new membrane domains, both of which essential for fertilization . The released hydrolytic enzymes digest the ZP, allowing the spermatozoa to penetrate the oocyte [32].
The AR seems to be physiologically induced by natural stimulants such as follicular fluid (FF), progestin, progesterone, and hydroxy progesterone, all of which bind to sperm receptors [28]. FF and cumulus cells have protein-bound progesterone, and this hormone is considered among the most important AR-inducing agents [43, 44]. However, other sperm-deriving factors may also play a role in this process. For instance, a link between estradiol locally produced by ejaculated spermatozoa, sperm capacitation, and AR have been demonstrated [45]. These stimulatory signals are intracellularly transduced via second messengers ultimately leading to exocytosis [46]. A number of second-messenger pathways have been identified in human spermatozoa, including those that result in the activation of cyclic adenosine monophosphate (cAMP), cyclic guanosine monophosphate (cGMP), and phospholipid dependent protein kinases [27, 47–49]. These kinases are called, respectively, protein kinases A, G, and C. It is possible that these pathways interact to assure an optimal response at the correct place and time during the fertilization process.
The AR is a time-dependent phenomenon that cannot take place prematurely or too late [47]. As such, acrosome integrity is crucial for normal fertilization both in vivo and in vitro. In fact, a high proportion of sperm with intact acrosomes is seen in ejaculates of normal men. In such individuals, ~ 5–20 % sperm cells exhibit spontaneous AR devoid of clinical significance [48]. In contrast, abnormal conditions affecting the sperm may lead to decreased fertilization ability. One example is acrosomeless round-headed spermatozoa (globozoospermic spermatozoa) that are unable to fertilize. Increased percentages of morphologically abnormal acrosomes have also been related to fertilization failure in assisted conception using conventional IVF [49]. In addition, premature AR and sperm inability to release the acrosomal contents in response to proper stimuli (AR insufficiency) have been associated with unexplained male infertility [50]. Although the cause of premature AR is unknown, the premature (stimulus independent) initiation of acrosomal exocytosis seems to be related to a perturbation of the plasma membrane stability. In this context, the AR may not involve a premature activation of the receptor-mediated process, but rather reflect an inherent fragility of the sperm membrane, leading to a receptor-independent acrosomal loss [51].
ASA may also adversely affect the ability of sperm to undergo capacitation and AR [15]. Chang et al. [52] reported a reduction in fertilization either by the action of IgG directly bound to sperm or IgM present in the female serum. Moreover, the combination of IgG and IgA has synergistic negative effects on fertilization [53–56]. Gonadotoxic substances can also influence AR. High concentrations of dietary phytochemicals, such as genistein isoflavone and β-lapachone, were shown to suppress the AR in a dose- and time-dependent manner in the rat model [57]. Inhibition of AR by genistein seems to involve the protein kinase C pathway while β-lapachone has a direct cytotoxic effect on the sperm cell membrane. It is suggested that genistein and β-lapachone may impact male fertility via AR suppression in high doses and AR induction in low doses [57]. Calcium channel blockers may also interfere with the AR exocytic event. Sperm incubation with different blockers, such as trifluoperazine (calmodulininhibitor), verapamil (Ca2+ channel inhibitor), and nifedipine (voltage-dependent Ca2+ channel inhibitor) significantly reduces the ability of hamster sperm to undergo AR [58]. The frequency of acrosome-reacted spermatozoa is also increased by the recreational use of marijuana [59]. Falzone et al. [60], studying the effect of pulsed 900-MHz GSM mobile phone radiation on the AR of human sperm , observed that despite not adversely affecting the AR rate, radiofrequency electromagnetic fields (RF-EMF) exposure significantly impaired sperm morphometry and the ability of sperm binding to the hemizona. Finally, Mukhopadhyay et al. evaluated the in vitro effect of benzo[a]pyrene, a substance present in cigarettes, on sperm HA and acrosome status of normozoospermic semen specimens. They observed a significant increase in sperm HA as well as in the premature AR rates in the presence of benzo[a]pyrene [61]. In conclusion, exogenous factors may affect the sperm’s ability to undergo timely AR, thus hindering such gametes infertile.
Laboratory Tests to Measure Fertilization Defects
Several tests can be used to measure sperm fertilization defects at different levels, as summarized in Table 11.3.
Table 11.3
Functional in vitro tests for measuring sperm fertilization defects at different levels
Level of assessment | Assay | Principle | Specimen | How results are expressed | Normal limits | Clinical relevance |
---|---|---|---|---|---|---|
Sperm binding to the human zona | Hemizona assay (HZA) | In HZA, a single ZP is split in half; one half is incubated with donor sperm (positive control) and the other half is incubated with patient sperm | Ejaculated sperm | Ratio of patient and control spermatozoa bound to the zona | ≥ 30 % | Men with defective ZP-binding showed a reduced chance of achieving successful fertilization when undergoing IVF [62] |
Sperm–zona binding ratio test (SZBT) | In SZBT, a complete zona is incubated with equal numbers of motile spermatozoa from control and test populations, each labeled with a different fluorescent dye | Ejaculated sperm | Identical to HZA assay | ≥ 30 % | Two of eighteen men with unexplained infertility showed lack of sperm binding to the zona despite having sperm morphology and hyperactivation status similar to fertile individuals [41] | |
Capacitation | Hyperactivated motility | Assessment of sperm motility using computerized motion analysis in conjunction with kinematics module | Washed sperm incubated under capacitating conditions | Percentage of motile sperm exhibiting hyperactivation motility | ≥ 20 % | Significant decrease in the percentage of hyperactivated sperm in infertile men compared to fertile controls after overnight incubation with capacitating conditions [63] |
Follicular fluid (FF)-induced hyperactivation significantly lower in patients with unexplained infertility in comparison with normal fertile men [41] | ||||||
Acrosome | ZP-induced AR (ZPIAR) | Assessment of the human sperm acrosome using lectins, monoclonal antibodies, or staining techniques to distinguish the proportions of cells with intact and reacted acrosomes after exposure to zona pellucida | Washed sperm incubated under capacitating conditions | A score is calculated by subtracting the baseline frequency of AR from the values obtained following incubation with zona pellucida | ≥ 15 % | Patients with unexplained infertility in whom sperm-ZP binding is normal may have a defective ZP-induced AR (ZPIAR), which will result in reduced sperm–ZP penetration and fertilization failure [64] |
Acrosome reaction to ionophore challenge (ARIC) | Baseline frequency of AR above 15 % indicates spontaneous and premature AR | Defective ZPIAR was found in 25 % of normozoospermic subfertile men [65] | ||||
Fusogenic ability of the acrosome-reacted sperm with the oolema | Sperm penetration assay (SPA) | Sperm incubation with capacitation media and acrosome reaction stimulants, and assessment of the number of sperm that penetrate zona-free hamster oocytes | Washed sperm incubated under conditions that stimulates capacitation and acrosome reaction | Percentage of eggs penetrated by at least one spermatozoon and the number of spermatozoa per penetrated egg | > 10 % of eggs penetrated | 34.1 % of patients with unexplained infertility showed less than 10 % oocyte penetration compared to none in a control group of fertile men [66] |
Assessment of Sperm Binding Using Human ZP Assays
The hemizona assay and the sperm–zona binding ratio test are used to evaluate sperm binding to the human ZP [12]. The hemizona assay uses human oocytes from which a single ZP is isolated and split in half. One half is incubated with fertile donor sperm (positive control) and the other half is incubated with patient sperm (Fig. 11.2). In the sperm–zona binding ratio test, a complete zona is incubated with equal numbers of motile spermatozoa from control and test populations, each labeled with a different fluorescent dye [67]. Irrespective of the method, the number of spermatozoa bounded to the zona is counted and results are expressed as a ratio of the number of test sperm to that of control, with less than 30 % considered abnormal [18]. These tests are clinically useful in cases of UI and low or failed fertilization after conventional IVF [12, 62, 68].
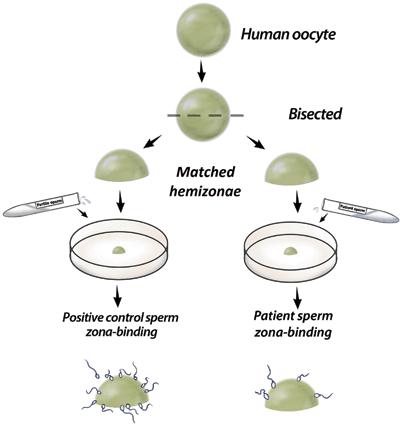
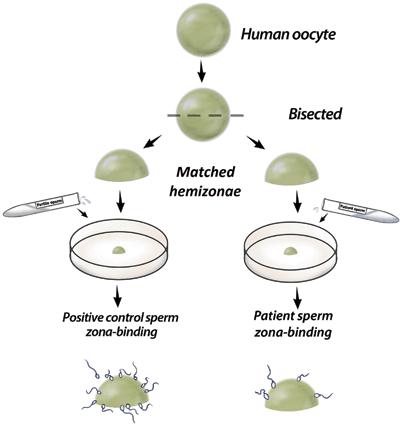
Fig. 11.2
Illustration depicting the hemizona assay. A single zona is bisected and each half is incubated with control and patient sperm suspensions. The number of spermatozoa from each population is counted and the number of test sperm is expressed as a ratio of that of the control
Assessment of Sperm Capacitation by Hyperaticvation Motility
Assessment of HA motility in vitro involves the use of computerized motion analysis in conjunction with a kinematics module to distinguish different subpopulations of motile spermatozoa. Populations of motile sperm are first incubated under capacitating conditions. This step can be carried out by the swim-up method. Briefly, liquefied semen is diluted with an equal volume of culture media (e.g., modified Biggers–Whitten Whittingham [BWW]. After centrifugation at 300g for 10 min, the supernatant is removed and the pellet resuspended in 2–3 mL of BWW. A second centrifugation is followed by resuspension to a final volume of approximately 600 µL of BWW supplemented with 0.3 % bovine serum albumin (BSA) factor V. This resuspension, which contains a large number of spermatozoa, is divided in aliquots of 200 µL that are underlayered beneath 800 µL of protein-supplemented BWW. The tubes are loosely capped and placed in a 37 °C incubator under 5 % carbon dioxide in the air, at a 45° angle for 1 h. Motile sperm migrates from the sperm suspension to the upper portion of the culture medium. After this period, the supernatant containing the subpopulation of active motile sperm is removed [69]. Then, these spermatozoa are incubated under capacitating conditions in a BWW medium supplemented with 3 % BSA, under 5 % carbon dioxide in the air, for a minimum of 3 h [51]. To assess the HA status of such sperm, aliquots are analyzed on a computer-assisted semen analyzer (CASA) to assess their motion characteristics. This system reconstructs sperm trajectories in successive video frames, and movement parameters are derived. Then, the analyzer segregates the subpopulation of spermatozoa that meets operator-defined kinematics criteria. Hyperactivated spermatozoa can be distinguished from nonhyperactivated ones by their greater curvilinear velocity (VCL; µm/s) and amplitude of the lateral head displacement (ALH; µm), and lower linearity (LIN; %). Curvilinear velocity is the total distance between two points in a given sperm trajectory during the acquisition period, divided by the lime elapsed. The amplitude of lateral head displacement measures sperm head oscillation whereas linearity (calculated as straight line velocity [VSL] /VCL×100 with 100 % representing an absolute straight track; VSL being the straight-line distance between two points divided by the acquisition time) measures linear progression [70] (Fig. 11.3). The definition of HA, as proposed by Burkman, is commonly used and includes all the following criteria: VCL ≥ 100 µm/s, LIN ≤ 65 %, and ALH ≥ 7.5 µm) [71]. In a previous report using this algorithm and the Hamilton–Thorn sperm analyzer, we noted that the proportion of sperm exhibiting HA under capacitating conditions was 21.4 % in normal men [72].


Fig. 11.3
The illustration depicts sperm motion characteristics as assessed by computer-assisted analyzers. VCL = curvilinear velocity, ALH = amplitude of lateral head displacement, and LIN = linearity (see text for definitions)
The clinical significance of determining sperm HA is reflected by its correlation with IVF outcomes and natural pregnancy rates [73]. A significant decrease in the percentage of hyperactivated sperm was observed in infertile men compared with sperm from fertile donors [63]. Moreover, computerized assessment of follicular fluid (FF)-induced HA was shown to be significantly lower in patients with UI in comparison with normal fertile men [74]. In fact, the absence of HA after the addition of FF was observed in 39 % of patients with UI . It is, therefore, possible that spermatozoa from such patients have reduced ability to penetrate through the oocyte vestments a result of this abnormal HA response to FF. Despite the aforementioned evidence supporting the role of HA in UI, some authors argue that the determination of HA using “snapshot” of sperm trajectories, as routinely performed by computer-aided sperm analysis instruments, is limited in their ability to truly assess the multiphasic nature of HA [75].
Assessment of Sperm Acrosome Using Lectins
Assessment of the sperm’s ability to undergo AR has been used as a test of sperm function for more than two decades [76]. Several techniques have been described to visualize the sperm acrosome including those using stains (fluorescent lectins), monoclonal antibodies, and electron microscopy [76]. Fluorescent lectins are often used; such stains bind to either the outer membrane or the acrosomal contents thus discriminating sperm populations with intact or reacted acrosomes [32, 48, 76–81].
Fluorescein isothiocyanate-conjugated peanut agglutinin (FITC-PNA) and fluorescein isothiocyanate-conjugated Pisum sativum (FITC-PSA) are the most used probes to assess the sperm acrosome. FITC-PNA binds specifically to the outer acrosomal membrane (beta-fraction of D-galactose) while FITC-PSA binds to the alpha-methyl mannose and labels the acrosome contents [79]. Because acrosomal loss can result from sperm death, AR testing is usually performed in conjunction with a test to monitor sperm viability. This strategy allows the distinction of reacted acrosomes in nonviable and viable spermatozoa; the latter represents the population of sperm with “true” AR. In a report using ejaculated specimens from normal men, Esteves et al. showed that the percentage of sperm exhibiting AR would have been overestimated if a simultaneous assessment of viability had not been used (Fig. 11.4) [79].
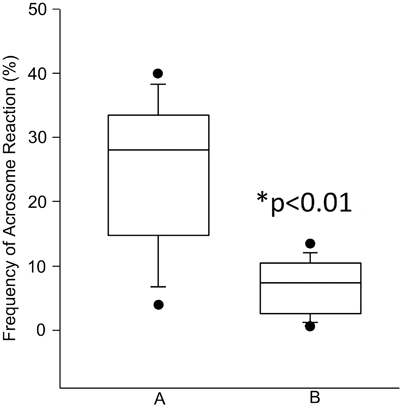
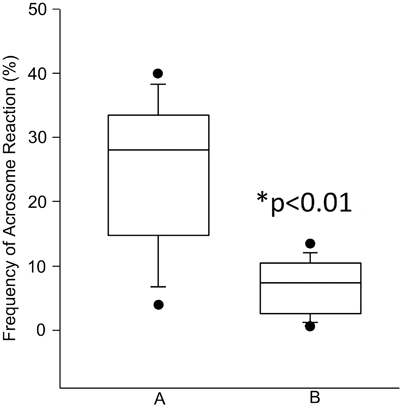
Fig. 11.4
Frequency of acrosome reaction (AR) in semen specimens of fertile donors. AR was determined by FITC-PNA, and sperm viability by Hoechst-33258 staining. a Total AR (normal reacted cells plus postmortem degeneration of the acrosomes). b AR in live human spermatozoa (* p< 0.01). Box covers the middle 50 % of the data values, between the lower and upper quartile. The central line is the median and the whiskers extend out to 80 % of the data. Bars represent values between the 5th and 95th percentile. (Reprinted from Esteves SC, Sharma RK, Thomas AJ Jr, Agarwal A. Evaluation of acrosomal status and sperm viability in fresh and cryopreserved specimens by the use of fluorescent peanut agglutinin lectin in conjunction with hypo-osmotic swelling test. Int Braz J Urol 2007; 33:364–74. With permission from International Brazilian Journal of Urology)
Cross et al. described the use of the supravital stain Hoechst-33258, a fluorescent DNA-binding dye with limited membrane permeability, combined with an immune-fluorescence technique to evaluate the AR in viable spermatozoa [78]. Alternatively, the viability of acrosome-reacted sperm can be assessed by the hypo-osmotic swelling test (HOST) [82]. HOST has potential advantages over protocols involving the use of fluorochrome-dye exclusion techniques. It precludes the use of an additional fluorescence filter cube to simultaneously assess the acrosome and to monitor viability thus reducing assay costs. We have previously demonstrated the usefulness of the HOST to monitor sperm viability [83]. In our study, the results of HOST and vital staining (Hoescht-33258 and eosin-nigrosin) were highly correlated in fresh sperm ( r = 0.95) but not in frozen-thawed ones ( r = 0.22) [83]. In a subsequent study, we confirmed that HOST can be used in conjunction with lectins to simultaneously assess viability and acrosome status of fresh sperm (Fig. 11.5) [79].
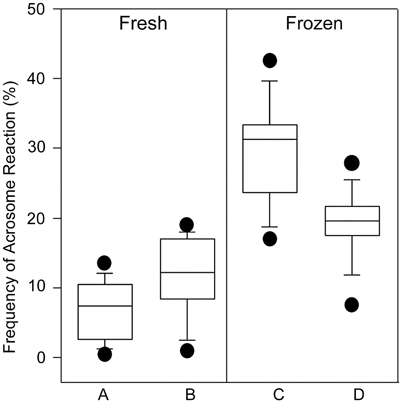
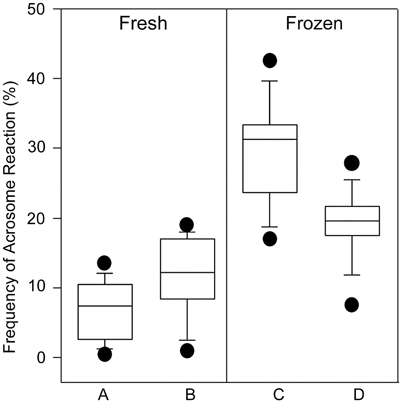
Fig. 11.5
Frequency of acrosome reaction (AR) in live human spermatozoa from 11 samples of fertile donors, as determined by FITC-PNA in conjunction with HOST (a and c), and FITC-PNA in conjunction with Hoechst-33258 (b and d), in fresh and post-thaw specimens. AR rates were not different in fresh specimens using either FITC-PNA/HOST or FITC-PNA/Hoechst-33258 ( p = 0.07). In frozen-thawed specimens, the frequencies of AR in viable spermatozoa assessed by the methods were significantly different ( p = 0.01). Box covers the middle 50 % of the data values between the lower and upper quartile. The central line is the median and the whiskers extend out to 80 % of the data. Bars represent values between the 5th and 95th percentile. (Reprinted from Esteves SC, Sharma RK, Thomas AJ Jr, Agarwal A. Evaluation of acrosomal status and sperm viability in fresh and cryopreserved specimens by the use of fluorescent peanut agglutinin lectin in conjunction with hypo-osmotic swelling test. Int Braz J Urol 2007; 33:364–74. With permission from International Brazilian Journal of Urology)
The use of FITC-PNA and HOST is our preferred approach to assess the acrosomal status of fresh sperm, and this method can be used in the investigation of men with UI . Briefly, specimens are incubated in hyposmotic conditions and smears are prepared on glass microscope slides. Sperm membranes are then permeabilized in ice-cold methanol and the fixed smears are immersed in a 40-μg/mL FITC-PNA solution. A microscope equipped with phase contrast and fluorescence epi-illumination module is used to examine the slides at 1000× magnification. Each spermatozoon is first examined for tail swelling using phase contrast with halogen illumination. Spermatozoa are classified as viable if tail swelling is observed after exposure to the hyposmotic solution. Then, illumination is changed to the mercury ultraviolet epi-illumination source for assessing FITC-PNA staining.
Alternatively, Hoechst-33258 can be used to monitor viability [79, 80]. In this method, sperm specimens are centrifuged and resuspended in 2 µg/mL Hoechst-33258 solution. The sperm suspension is incubated for 10 min in the dark and then spermatozoa are washed to remove excess stain. Smears are prepared on glass microscope slides and then immersed in ice-cold methanol to permeabilize the sperm membranes. The fixed smears are immersed in a 40-µg/mL FITC-PNA solution, incubated at room temperature, and washed gently in PBS to remove the excess label. A microscope equipped with phase contrast and fluorescence epi-illumination module is used to examine the slides at 1000× magnification. The illumination is selected to the mercury ultraviolet epi-illumination source for assessing FITC-PNA and Hoechst-33258 labeling by using appropriate filter cubes. FITC-PNA and Hoechst-33258 fluoresces “apple-green” and bright medium blue, respectively (Fig. 11.6). Examination of the same spermatozoon for FITC-PNA labeling and for Hoechst-33258 staining is performed by interchanging the fluorescence filter cubes.
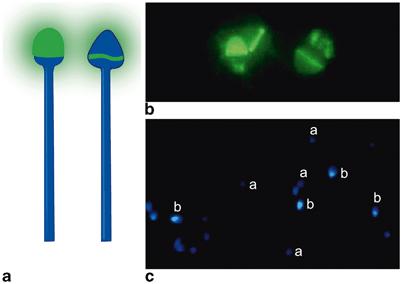
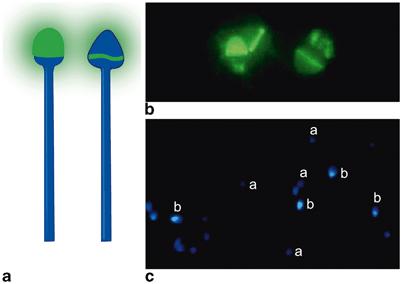
Fig. 11.6
Assessment of acrosome reaction (AR). a Illustration of spermatozoa labeled by FITC-PNA: spermatozoa with intact acrosomes show uniform apple-green fluorescence at the acrosomal region of the sperm head ( left); staining is limited to the equatorial segment of the acrosome in spermatozoa exhibiting AR ( right). b Photomicrographs of spermatozoa labeled by FITC-PNA: only the equatorial segment of the acrosome is stained in spermatozoa exhibiting AR. c Photomicrographs of spermatozoa stained with Hoechst-33258: live spermatozoa show pale-blue fluorescence due to the exclusion of the dye (a) while dead spermatozoa show bright blue-white fluorescence (b). (Adapted from Esteves SC, Sharma RK, Thomas AJ Jr, Agarwal A. Evaluation of acrosomal status and sperm viability in fresh and cryopreserved specimens by the use of fluorescent peanut agglutinin lectin in conjunction with hypo-osmotic swelling test. Int Braz J Urol 2007; 33:364–74. With permission from International Brazilian Journal of Urology)
To evaluate the enzymatic release from sperm acrosomes, artificial (ionophores) or physiological inducers (progesterone, FF, and ZP) are used. After stimulation by the aforementioned stimulants, the proportion of reacted spermatozoa is assessed. Our preference is to use calcium ionophore A23187, which exchanges Ca2+ for 2H+. The ionophore-induced AR, also termed “AR to ionophore challenge (ARIC)” has been validated as a sperm function test with high predictive value for the outcomes of assisted reproduction techniques [17, 39, 77, 84–86]. Briefly, motile spermatozoa are first capacitated by incubation in sperm culture medium at 37°C for 3 h. Then, capacitated sperms are divided into two equal aliquots: one is incubated with 2.5 μmol/L calcium ionophore A23187 solution, and the other is treated with dimethylsulfoxide (10 %, v/v) as a control [49, 80, 81]. Acrosome status can be determined by FITC-PNA or FITC-PSA in combination with the nuclear stain bis-benzimide or HOST to monitor viability. The ARIC test score is calculated by subtracting the baseline frequency of AR from the values obtained following ionophore challenge. ARIC results have shown to be a reliable indicator of fertility potential in IUI and conventional IVF treatment cycles [86]. The AR threshold values for predicting complete fertilization failure and pregnancy in IVF, as determined by receiver-operating curves (ROC) analysis, were 21 and 26 %, respectively [86]. Moreover, no pregnancies were obtained by IUI when ARIC results were below 11 % [86]. In ICSI , ZP penetration and oolemmal fusion are bypassed and thus the role of the AR is unclear. In fact, ARIC results do not seem to predict ICSI outcome since injections using sperm from different acrosome reacted sperm populations had no impact on fertilization rates [28, 86]. As an alternative to ionophores, AR can be induced by ZP (ZP-induced AR [ZPIAR]. In one study, defective ZPIAR was found in 25 % of normozoospermic subfertile men [65].
Patients with AR defects usually have UI and normal sperm–ZP binding. However, their sperm fail to penetrate the ZP and have zero or low rates of fertilization with conventional IVF [64]. The diagnostic feature is that very low proportions of sperm undergo AR after ionophore or ZP challenge. Nevertheless, these patients achieve normal fertilization and pregnancy rates with ICSI [87].
Interestingly, seminal zinc concentrations were shown to be significantly higher in a group of normozoospermic men with defective ZPIAR. Zinc has a role as a decapacitation factor by binding to the sperm plasma membrane. High seminal zinc concentrations seem to have an adverse effect not only on ZPIAR [88] but also in the AR induced by calcium ionophore [89]. The mechanism of AR induced by ionophores differs from physiological AR induced by the ZP [32]. While the physiological AR involves activation of several signal transduction pathways, the ionophore-induced AR involves mainly a chemical effect on calcium influx that partially reflects the physiological AR process [32].
Assessment of the Fusogenic Ability of the Acrosome-Reacted Sperm with the Oolema (Enhanced Sperm Penetration Assay (SPA))
The ability of the equatorial region of the acrosome-reacted human sperm to fuse with the vitelline membrane of the oocyte is tested using the SPA, also known as the zona-free hamster oocyte penetration test. Although this test does not assess sperm–ZP, it measures the spermatozoon’s ability to undergo capacitation, AR, fusion and penetration through the oolema, and decondensation within the cytoplasm of an oocyte [12].
Sperm preparation for the test includes two steps: (i) preparation of a selected motile sperm population free of seminal plasma (e.g., by swim-up or discontinuous gradient technique) and (ii) incubation and treatment of the selected sperm population under conditions that stimulate capacitation and AR. The calcium ionophore (A23187) incubation method is recommended for this step. The final volume should be calculated to obtain a sperm population of about 10 million motile cells per milliliter. Hamster oocytes from which the ZP had been removed are incubated with sperm specimens. A 20-µL oil-covered droplet of the sperm suspension is prepared using a Petri dish, and 10–15 zona-free eggs are transferred into the droplet. The dish is incubated under 5–6 % CO2 in the air at 37°C for 3 h. Afterwards, the zona-free eggs are examined using phase-contrast microscopy. The presence of either swollen sperm heads or male pronuclei (slightly larger than the female one and that contains small dark nucleoli), or both, are used to confirm sperm penetration [21] (Fig. 11.7).
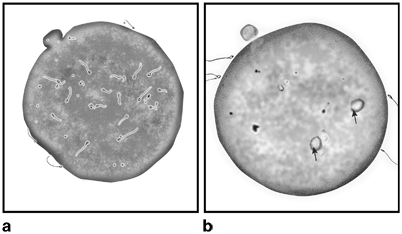
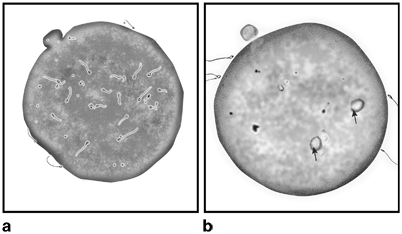
Fig. 11.7
Illustration depicting the sperm penetration assay (SPA). Human spermatozoa are seen into the hamster oocyte cytoplasm a. The arrows point to the swollen sperm heads indicative of sperm decondensation within the oocyte’s cytoplasm b.
The assay is scored by calculating either the percentage of ova that is penetrated by at least one spermatozoon or the average number of sperm penetrations per ovum. Normal sperm are able to penetrate 10–30 % of hamster ova [90]. The assay should be run in parallel with a positive control semen specimen exhibiting > 50 % penetration. Recent refinements of SPA include sperm incubation with more potent capacitating media, which allow the majority of ova to be penetrated; scores are obtained by calculating the number of sperm that penetrate each ovum. Aitken et al. reported that approximately one third of men with UI had less than 10 % ova penetration compared to none in the control group of fertile men [66]. The ability of the SPA to predict the success or failure of IVF is debatable. Some investigators have shown no correlation with an abnormal test [91], whereas others have claimed 100 % predictability [92]. Taking an average from different studies, a normal SPA seems to have 70 % predictability of fertilization in in vitro conditions [93]. Nevertheless, semen samples that fail to fertilize the hamster ova are usually unable to fertilize human ova. Although the SPA is considered a research tool, it may be of clinical value for men with UI with poor fertilization rate in IVF.
Oxidative Stress
ROS are byproducts of oxygen metabolism and energy production that act as regulators of vital physiological intracellular processes. In the male reproductive tract, the primary producers of ROS are the leukocytes and immature spermatozoa. Small quantities of ROS have important roles in sperm function including regulation of capacitation, AR, HA, and the fusion of spermatozoa with the oocyte [94]. In sperm, ROS are generated by two ways, (1) NADPH oxidase system at the level of the sperm plasma membrane [95], and (2) NADH-dependent oxido-reductase system at the mitochondrial level, which is the major source of ROS in sperm of infertile men [96, 97]. When ROS levels disproportionately increase, mainly by the presence of superoxide (O2 –), hydroxyl radicals (OH), or nitric derivatives (NO), compared with the neutralizing capacity of intracellular and extracellular antioxidants, or when a reduction in the antioxidant capacity occurs, oxidative stress usually follows and target lipids, proteins, sugars, and nucleic acids through a variety of mechanisms .
In sperm, ROS primarily target membranes and DNA. By having an available valence electron, oxidative species interact with the cell membrane and cause lipid peroxidation of which the final product is malondialdehyde (MDA), a measurable compound used to determine the extent of ongoing oxidative modification of membrane-polyunsaturated fatty acids (PFA). The peroxidation of PFAs has detrimental effects on the structure of the sperm head and midpiece membranes, causing changes in the sperm membrane fluidity that ultimately lead to suboptimal sperm motility and fertilization [94]. Oxidative species also target DNA molecules and cause a variety of defects in the cell genetic material. ROS can inflict serious damage to DNA, including point mutations, polymorphisms, deletions, chromosomal rearrangements, frame shifts, and single-stranded or double-stranded breaks [98]. The OS-induced sperm damage has been suggested to be a significant contributing factor in 30–80 % of all cases of male infertility [99]. Likewise immunologic infertility and fertilization defects , oxidative stress cannot be detected in the routine semen analysis .
Laboratory Tests to Measure ROS
The methods used for ROS detection are broadly divided into two major categories based on their ability to directly or indirectly measure oxidative radicals (Table 11.4). Indirect measurements involve the assessment of lipid peroxidation products (MDA), protein oxidation products (such as protein carbonyl) and oxidized DNA (8-hydroxy-2′-deoxyguanosine [8-OHdG]) [105] whereas direct oxidative-stress measurements include total or specific ROS level in semen and total antioxidant capacity (TAC) .
Table 11.4
Tests for measuring reactive oxygen species in UMI
Assay | Principle | Specimen | How results are expressed | Normal limits | Clinical relevance |
---|---|---|---|---|---|
Thiobarbituric acid reactive substances (TBARS) | Malondialdehyde (MDA), a byproduct of lipid peroxidation, condense with two equivalents thiobarbituric acid to give a fluorescent red derivative that can be assayed spectrophotometrically | Semen and seminal plasma | Absorbance at 532 nm is recorded and expressed as nmoL MDA/10 × 107 cells, nmoL MDA mL−1 seminal plasma, or nmoL MDA/total seminal plasma | ROS degrade polyunsaturated lipids of sperm membrane forming MDA, which is a reactive aldehyde toxic to sperm | |
Chemiluminescence | Intra- and extracellular ROS levels (mainly H2O2, O2 ●−, and OH−) react with probes and emit photons that can be measured using a luminometer. The final chemiluminescent signal is the integrated sum of the partial signals generated by every spermatozoon
![]() Stay updated, free articles. Join our Telegram channel![]() Full access? Get Clinical Tree![]() ![]() ![]() |