Introduction
Robotic-assisted surgical devices (RASDs) have revolutionized minimally invasive surgery since 1994. The market leader, Intuitive Surgical, reports their product involvement in over 7.2 million procedures worldwide, and in 2018, they generated $4.5 billion in revenue. This sector is expected to have year-over-year growth to $9.7 billion by 2023 as RASD adoption increases, leaving room for rival platforms.
Basic features of RASDs
Current RASDs represent computer assistance in surgery and lack autonomous elements. Telepresence immerses the surgeon into the operative field where mechanized arms manipulate tissue via end effectors and are controlled from a decoupled human-machine interface (HMI). The relationship leverages technology to augment visualization and dexterity beyond that in open or laparoscopic surgery. Detachment from the instruments also restores intuitive hand-eye coordination by lessening the impact of the fulcrum effect, whereby extracorporeal movements are inverted intracorporeally around a pivot. The fulcrum effect steepens the learning curve of laparoscopy and contributes to the ergonomic challenges posed by port triangulation to maintain range of motion.
With a stereoscopic endoscope, surgeon consoles provide high-definition (HD) video, magnification, and depth perception of the operative field. Simulating depth perception can necessitate an enclosed console with monitors for each eye or require three-dimensional glasses to view a single monitor open to the operating room. Closed consoles utilize microphones and speakers to communicate with operating room staff such that some RASDs favor an open design. Display(s) provide critical information, including the types of tools engaged, energy inputs, endoscope parameters, system warnings/errors, etc. In addition to white light, endoscopes may provide near-infrared light to display intravascular or intraluminal fluorescence by exciting injected indocyanine green (i.e., Firefly).
Movements of the HMI are mapped in three-dimensional space and translated to movement at the tool tips. For example, Intuitive Surgical’s finger grip HMI is commonly referenced and is used to move/reorient a virtual “control point” located between the fingers. Data processing allows motion scaling, which converts larger movements by the surgeon into precise movements intracorporeally. Tremor reduction is applied by separating purposeful movements from high-frequency oscillations detected in the interface.
At the bedside, a variable number of motorized arms manipulate a variety of interchangeable instruments and endoscopes passed intracorporeally through trocars. Arms mounted on individual carts can facilitate arm positioning while allowing the minimum number to be used for a surgery, freeing unused arms for concomitant surgeries. A remote center is created by each arm to reduce forces on the body wall. The joints of the arms plus instruments provide varying degrees of freedom (DOF), the total number of dimensions linked joints can maneuver within to move a tool tip. Traditional laparoscopic instruments have five DOF (including end effector function) while RASDs commonly provide seven DOF by enabling wristed function ( Fig. 5.1 ). Even more dexterity is provided by the wrist’s proximity to the tool tip relative to open surgery.

Brief history of robot-assisted surgery
RASDs originated from the commercialization of technology developed from ambitious aims. Industrial robots were already used in surgery in the late 1980s but did not involve laparoscopy and were procedure-specific. Two distinct collaborations pushed history forward ( Fig. 5.2 ).

Computer Motion, a company founded in 1990, and the National Aeronautics and Space Administration (NASA) envisioned remote-controlled arms to robotize tasks in space. AESOP, or Automated Endoscopic System For Optimal Positioning, was subsequently commercialized as a voice-controlled arm used to move an endoscope hands-free in laparoscopy. In 1994, AESOP became the first FDA-approved surgical robot through the 510K pathway by comparing itself to nonrobotic laparoscopic accessories. This decision enabled rapid commercialization and set a precedent for rivals to follow. To this day, RASDs obtain FDA clearance as class II devices under “endoscope and accessories”.
At the same time, the Stanford Research Institute (SRI) alongside the Defense Advanced Research Products Agency (DARPA) imagined a means for telesurgery in the battlefield whereby time-sensitive procedures are performed nearest to the site of casualty. Construction of SRI’s telemanipulation with telepresence prototype began in 1989 with features similar to RASDs today ( Fig. 5.3 ). By 1995, patents filed by SRI were licensed to the newly founded company, Intuitive Surgical, and the technology was developed in parallel for civilian use. Intuitive Surgical added wrist function to their first prototype, Lenny, named after Leonardo da Vinci. Notably, the prototype was patented as the EndoWrist in 1999. Their second prototype, Mona, allowed sterile instrument changes and was used in their first human trial in 1997 to perform a cholecystectomy. The da Vinci surgical system was the third iteration and received FDA approval in 2000 for general surgery procedures under the precedent 510K pathway.

The da Vinci surgical system consolidated its three arms into a single bedside cart, and a fourth arm was added in 2003. The RASD has seen iterative improvements over time (summarized in Table 5.1 ). Ever since FDA approval of robot-assisted radical prostatectomy in 2001, Intuitive Surgical’s systems have been applied to the entire spectrum of urologic care and represent a benchmark RASD. Indeed, Computer Motion went on to develop Zeus, a complete surgical system based on AESOP’s architecture. Not surprisingly, the two companies traded many firsts and several lawsuits until their merger in 2003. Zeus was subsequently abandoned, and some of its technologies were incorporated into the da Vinci surgical system.
S (2006) | Si (2009) | Xi (2014) |
---|---|---|
|
|
|
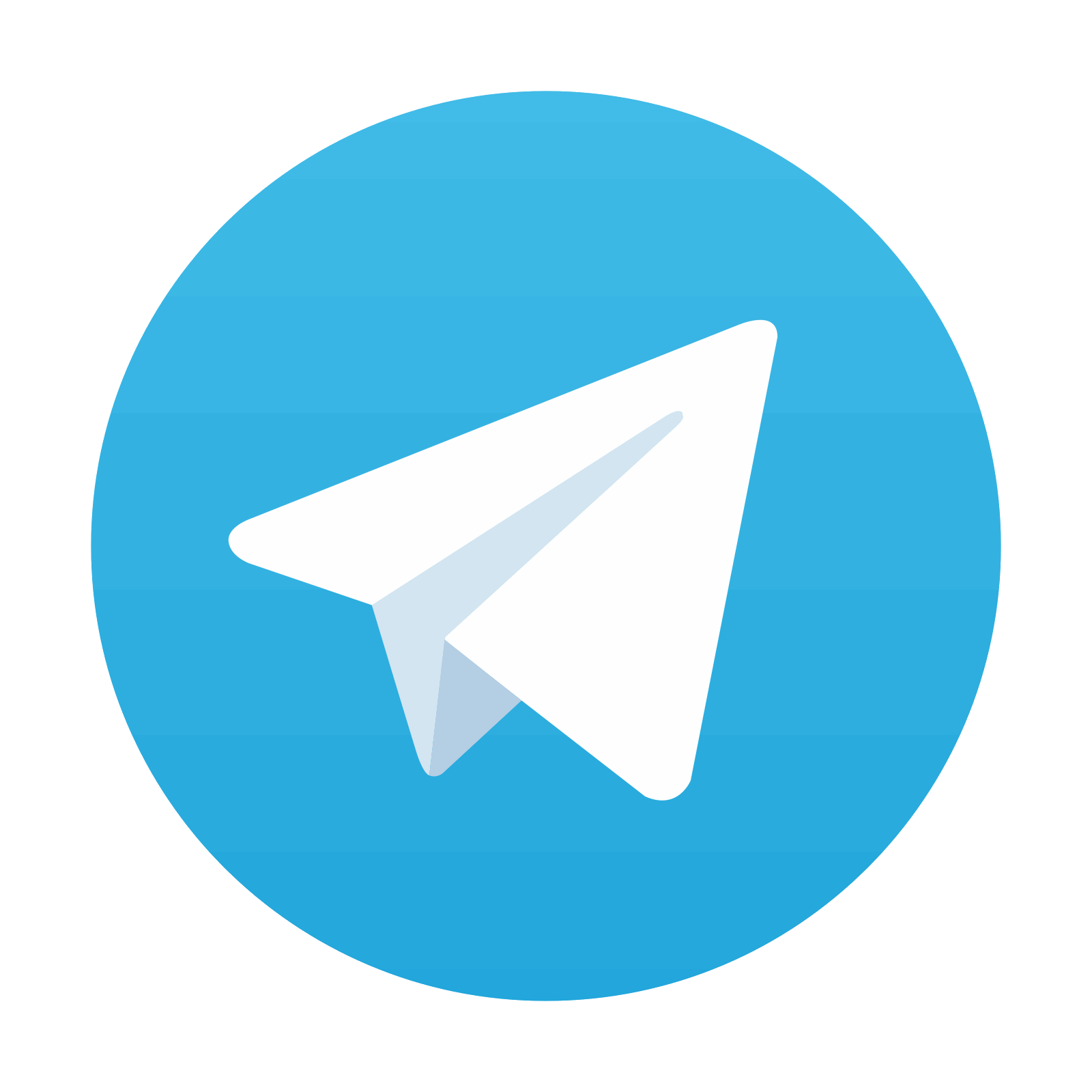
Stay updated, free articles. Join our Telegram channel

Full access? Get Clinical Tree
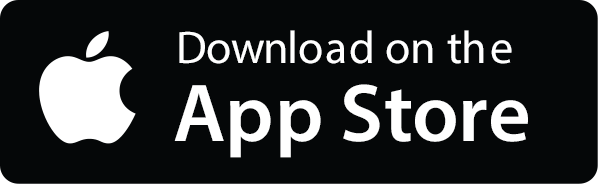
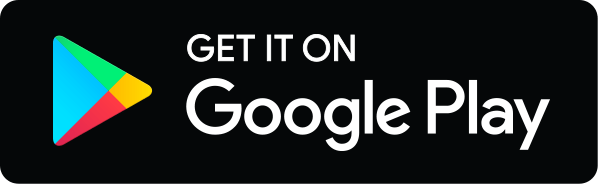
