39 Omar M. Aboumarzouk1 & Francis X. Keeley Jr.2 1 Department of Urology, Glasgow Urological Research Unit, Queen Elizabeth University Hospital, Glasgow, UK 2 Bristol Urological Institute, Bristol, UK The natural progression from open surgery to minimally invasive procedures is a testament to the technological evolution that has brought about the modern day ureteroscope. Young performed the first recorded ureteroscopy in 1912 using a 9.5 Fr pediatric cystoscope [1]. Since then, advancements in endoscopic design and the use of fiber‐optic technology has led to smaller scopes with improved maneuverability, allowing for not only rigid and semirigid ureteroscopes, but also flexible ureteroscopes. The latest generation of ureteroscopes now provides high‐definition digital images of the ureter and intrarenal collecting system, allowing procedures to be carried out safely, with high success rates and minimal morbidity in adults and children. To appreciate how rigid and semirigid ureteroscopes have evolved through time, this chapter will portray a summary of key technical developments, starting with the first endoscope, the effect of changes in instrumentation, and progressing through to the latest digital technology. A description of key features of ureteroscopes is then given, discussing their similarities and differences and potential future trends for their further development. The history of the endoscope dates back to 1853 [2–4] and has developed considerably to the present day ureteroscopes (Table 39.1). The traditional components required to carry out a rigid endoscopic procedure are the telescope with working channels for irrigation and instrumentation, a light source, optics to convey the image, and a camera system to capture the image. This has been revolutionized by the use of fibroptic technology; however, with the increasing speed of technological evolution, fiber‐optics will one day make way for the digital era. Table 39.1 Landmark evolutionary steps in the development of the rigid endoscope. The forerunner of the endoscope can be traced back to 1806 with the construction of the light‐guiding instrument or “Lichtleiter” by Phillip Bozzini for direct inspection of internal organs [5]. This scope was basic in design, consisting of a direct vision hollow tube through which candlelight was transmitted by a mirror. The French surgeon Antoine Jean Desormeaux further developed the Lichtleiter, producing the “endoscope” in 1853 using a system of mirrors and lens, and a kerosene flame to provide light to examine the urethra and bladder (Figure 39.1). Gustave Trouvé further upgraded indirect illumination by candlelight to electrical illumination using glowing heated platinum wires at the tip of the instrument in 1873 [5]. The filaments were later replaced by Edison’s incandescent lightbulb. The Nitze‐Leiter cystoscope, a rudimentary version of the modern cystoscope, was constructed in 1879 and incorporated a simple lens system into the viewing tube [5]. Figure 39.1 Antoine Jean Desormeaux modified the “Lichtleiter” to produce the “endoscope.” Source: A.J. Desormeaux, De l’endoscope. 1865. Reproduced courtesy of Wellcome Library, London, UK. Fiber‐optic lighting technology was introduced in the 1960s, replacing the incandescent bulb and revolutionizing the manufacture of rigid endoscopes. The design was based on the principle of total internal reflection: when light travels in a transparent medium such as glass, internal reflection of the light occurs at the interface between the medium and its surroundings, as demonstrated by John Tyndall in 1854 [6]. This physical property of internal reflection allows the “bending” of light within flexible glass. Thus, light travelling inside a small‐diameter flexible glass fiber surrounded by cladding of lower refractory index can be transmitted over a long distance with minimal degradation. The benefit of modern fiber‐optic lighting is greater illumination by cooler light, ultimately making it safer. An additional benefit is that the scopes can be made with a smaller profile shaft, allowing more room within the shaft for the addition of an irrigation and instrument channel. The fiber‐optic cable is often attached via a light post to the endoscope, but may alternatively be built into the design of the scope. The innovative work of the British physicist Harold Hopkins led to the next major breakthrough in rigid endoscopic design with the introduction of the rod–lens system in the 1960s [7]. Until then, the shaft of the scope consisted of a hollow tube with a series of thin relay lenses separated by long air spaces. The relay lenses had to remain in precise alignment and any displacement of the lens resulted in a significant loss of image transmission. There was also a significant loss of light transmission with this system. Hopkins replaced the thin relay lenses within the shaft of the endoscope with long, contoured glass rods acting as the transmission medium, while the thin pockets interspersed between the glass rods acted as lenses. With the telescope primarily being made of glass, which has a higher refractive index than air, light transmission could be increased ninefold over previous lens systems, with the additional benefit of reduced image distortion and a wider viewing angle. The outer diameter of the endoscope shaft could also be reduced, paving the way for the development of the rigid ureteroscope and the introduction of working channels. The old lens system was notorious for its lack of durability and the Hopkins rod–lens system produced a significant improvement in this area. There were a few apparent weaknesses in the rod–lens design: any deviation in the use of the cystoscope from being used in straight lines, as can occur with the application of torque to the shaft, could lead to misalignment of the rod–lens setup and the permanent disappearance of up to half the image. The problem manifests itself as a half‐moon or crescent‐shaped defect when viewing through the scope eyepiece. This design flaw would be seen to be disadvantageous in the passage of the endoscope through the natural undulations of the ureter. A further consideration for ureteroscopic design centers on the diameter of the lens that dictates the size, or degree of magnification, of the image; the reduced diameter of the ureteroscope would inadvertently have a smaller image than the larger cystoscope. Young undertook the first recorded ureteroscopy in 1912, and it took another 65 years before Goodman [8] and Lyon et al. [9] ventured into the ureter, performing distal ureteroscopy in both male and female patients (Table 39.2). Goodman reported using an 11 Fr pediatric rod–lens cystoscope to inspect the distal ureter in three adults [8]. In one of these patients, a distal ureteral tumor was fulgurated, marking the first ureteroscopically treated tumor. Lyon et al. reported ureteroscopy in five adults using sound dilators for ureteral dilation before passing an 11 Fr pediatric cystoscope [9]. However, most of these early ureteroscopy patients were women. Lyon et al. reported in 1979 the use of specially designed ureteral dilators attached to the tip of a flexible probe. They were able to dilate the orifice to 16 Fr, permitting insertion of a standard‐length 13 Fr cystoscope for distal ureteroscopy in men. These early investigators demonstrated the ease and safety of rigid ureteroscopy for the distal ureter in both sexes. Table 39.2 Landmark evolutionary steps in the development of the rigid ureteroscope. The first rigid ureteroscope specifically designed for ureteric use was produced in 1979 by Richard Wolf Medical Instruments and was used by Lyon et al. [10]. Modeled on a pediatric cystoscope, this 13 Fr instrument had a working length of 23 cm and sheath sizes of 14.5 and 16 Fr; a 16 Fr resectoscope sheath was also available. The 13 Fr sheath was used only for inspection, but the larger sheaths allowed simultaneous passage of a ureteral catheter or basket for stone manipulation and removal. For the first time, ureteral calculi were visualized, engaged in a basket, and removed [10, 11]. A longer ureteroscope that could reach the renal pelvis was developed by Enriqué Pérez‐Castro in collaboration with Karl Storz Endoscopy in 1980 [12]. Its 39 cm length allowed inspection of the renal pelvis for the first time. Further ureteroscopic modification was not due to advances in scope technology, rather the introduction of and progression in instrumentation. The launch of ureteroscopic ultrasonic lithotripsy for the treatment of urolithiasis led to modifications in ureteroscopic configuration. Initially, the probes were hollow and 8 Fr in diameter, requiring removal of the telescope and blind stone lithotripsy. These were replaced by smaller solid probes (1.5–2.0 mm) that could pass through the working channels but that were too rigid to negotiate the angles of the instrument working ports. This led to the development of the offset eyepiece which allowed the direct passage of the probe through a straight working channel under direct vision. Rigid ureteroscopes were later designed with an interchangeable offset eyepiece and standard telescope, allowing easier passage of the scope. Due to difficulties and complications from inserting rigid rod–lens type ureteroscopes with diameters approaching 14.5–15 Fr, it became apparent that smaller ureteroscopes were needed. With the availability of smaller working instruments, Huffman described a compact ureteroscope that was 8.5 Fr in diameter and had a working channel of 3.5 Fr [13]. The rod–lens telescope was integrated into the ureteroscope, which helped decrease the outer diameter while maintaining a good‐sized working channel. The rigid ureteroscope has not deviated significantly from the original pediatric cystoscope used to inspect the ureter. Any development has centered on the improvement in the light source, the introduction of the rod–lens optics system, the launch of a single working channel, and changes in the overall ureteroscope dimensions. That said, over the years the ureteroscope has become narrow enough to ensure easier ureteric access and long enough to venture into the renal pelvis, and has a working channel to allow instrumentation for intervention and diagnostic purposes. The rod–lens systems has provided excellent optical quality over the years, but scopes incorporating this design tend to have larger diameters and are prone to damage following angulation of the ureteroscope. The “angle of view” in an endoscope can vary from 0°, providing a straight view of the structure in question, to 70°, which allows inspection of tissues at an oblique angle from the straight axis. These rod–lens ureteroscopes were either interchangeable (from 0 to 70° angle of view) or integrated within the ureteroscope itself. The 70° lens was primarily used to inspect the renal pelvis and calyces, although these lenses are now virtually obsolete with the development of flexible ureterorenoscopes. The few current rod–lens ureteroscopes have integrated telescopes because of their smaller size advantage, and the angle of view through the objective lens is fixed at 0–5°. The advantage of a slight angulation on the direct vision lens is easier and quicker visualization of working instruments being passed out of the tip, reducing the potential for inadvertent iatrogenic injury to normal urothelium. The “field of view” is often quoted for ureteroscopes and is the entire view seen by the eye when it is trained in any particular direction. For endoscopes, this can vary from 60° to 90°, depending on the type of instrument. Ureteroscopes had a standard eyepiece design, which sees the ocular lens drawn in a straight line through the shaft of the scope (Figure 39.2). When rigid ultrasound probes were first introduced for ureteroscopic lithotripsy, an offset ocular configuration was trialed that allowed for direct access to the instrumentation port, allowing easy entry and removal of rigid accessories without impairing the view of the scope.
Rigid Ureteroscopes
Introduction
Rigid and semirigid ureteroscopes
Rigid ureteroscopes
Historical perspective
Year
Pioneer
Rigid endoscope and technologic advances
1806
Phillip Bozzini
German doctor who introduced the “Lichtleiter” or light‐guiding instrument
1853
Antoine Jean Desormeaux
French surgeon who first introduced the “endoscope.” Often considered as the father of endoscopy
1873
Gustave Trouvé
French inventor who moved the light source from outside to the inner tip of the endoscope and developed the “polyscope”
1879
Maximilian Nitze and Joseph Leiter
German urologist (Nitze) and Viennese electro‐optician (Leiter) credited with the invention of the modern cystoscope
1960s
Several contributors
Various people introduced the use of fiber‐optic cable, enabling transmission of light from an external source
1960s
Harold Hopkins
British physicist who filed a patent for the “rod–lens” system that was later adopted by Karl Storz in 1965
Endoscopic evolution
Year
Pioneer
Rigid ureteroscope technological advances
1977
TM Goodman and Edward Lyon
Provided the first reported series of distal ureteroscopically performed cases
1979
Richard Wolf
Credited with the design and construction of the first rigid ureteroscope
1980
Enriqué Pérez‐Castro
Developed the “long” ureteroscope in collaboration with Karl Storz Endoscopy
1980
ACMI
The Rigiflex was the first ureteroscope to adopt the use of fiber‐optics to allow the “gooseneck” eyepiece
1989
Huffman
Produced the 8.5 Fr “compact ureteroscope” with a 3.5 Fr working channel. The rod–lens was integrated into the ureteroscope
General properties
Optics, angle, field of view, and eyepiece
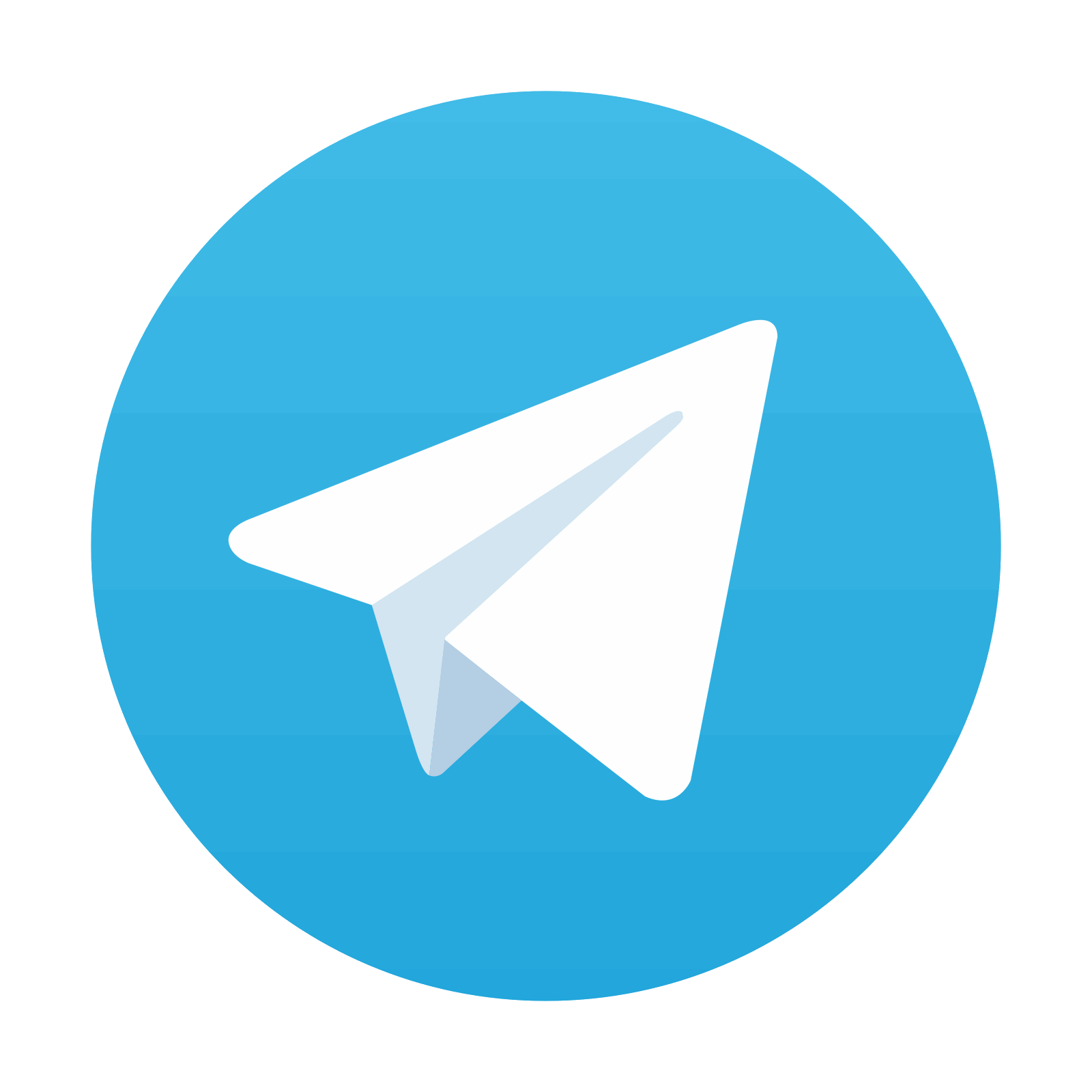
Stay updated, free articles. Join our Telegram channel

Full access? Get Clinical Tree
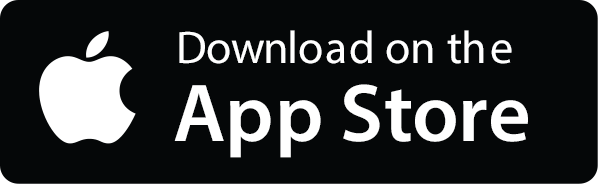
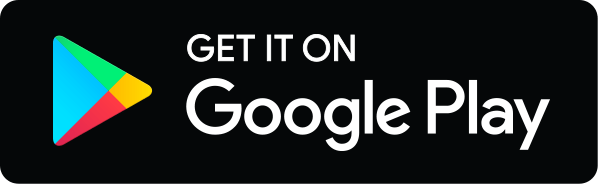